Acknowledgment
Special thanks to Britt Gott, MS, for her editorial input and assistance with the compilation of this chapter.
Introduction
Fortunately, most individuals who suffer from major depressive disorder (MDD) respond to first-line antidepressant treatments [pharmacotherapy and psychotherapy; ( ; )]. Over the course of the past 20 years, there has been increasing awareness that a significant subset of patients suffer from a more virulent form of MDD that does not respond to a series of standard antidepressant treatments. These patients are said to suffer from “treatment-resistant major depression” (TRMD). Though the field of psychiatry has yet to agree upon how many failed treatments constitute TRMD, there is emerging consensus that failure to respond to two first-line treatments from different antidepressant classes of adequate dose and duration is a clinically useful definition, as the likelihood of responding to a third treatment is substantially lower ( ; ).
Though a relatively small subset (20%–30%) of MDD patients have TRMD, given the relatively high lifetime prevalence of MDD in the United States (10%–20%), approximately 7–20 million Americans suffer from TRMD, which represents a significant and increasingly prevalent public health concern ( ; ; ). Evidence supports that TRMD patients are at significantly higher risk for suicide attempts, have greater rates of hospitalization and health care utilization, and per patient are much more costly to treat than non-TRMD MDD patients ( ; ; ; ; ; ; ).
Over the course of the past 15 years, there have been important discoveries allowing the investigation and clinical application of novel approaches to the treatment of TRMD. One of these novel treatment classes explored is inhaled (gaseous) agents. This chapter reviews three emerging inhaled treatments which target TRMD: nitrous oxide (laughing gas), isoflurane, and xenon. We also briefly discuss the use of aromatic compounds in the treatment of mood disorders.
Nitrous oxide
Colloquially referred to as “laughing gas,” nitrous oxide is a colorless, odorless anesthetic gas with a long history of medical use dating back 150 years ( ). The current clinical uses of nitrous oxide are primarily as an inhalational anesthetic agent or employed during short, painful procedures in dentistry, labor and delivery, and emergency medicine ( ; ; ). Most recently, nitrous oxide has emerged as a potential treatment for depression, suicidal ideation, and posttraumatic stress disorder.
Nitrous oxide for major depressive disorder
Similar to ketamine, which has rapid efficacy for treatment-resistant depression, nitrous oxide is a highly potent N -methyl- d -aspartate receptor (NMDAR) antagonist. This knowledge, along with the well-established euphoric effects of nitrous oxide, prompted speculation that it may have clinical antidepressant effects.
conducted a proof-of-concept double-blind, prospective, cross-over trial of 20 TRMD patients using inhaled nitrous oxide. TRMD patients underwent serial treatments with an hour-long inhalation with either 50% nitrous oxide/50% oxygen (active treatment); or 50% nitrogen/50% oxygen (placebo). These sessions were randomized in order and separated by 1 week. To be eligible for the trial, patients had to have failed at least 2 adequate dose-duration antidepressant trials (confirmed by medical record review), and could not have recent history of substance abuse (past 12 months, except nicotine), any lifetime history of psychotic or bipolar illness, or severe personality disorder. Patients were allowed to maintain their existing antidepressant treatments but were requested not to start any new antidepressant treatment or modify their treatment dosage in the 4 weeks prior to trial entry. To avoid nitrous oxide’s potential euphoric effects being confounded with antidepressant effects, the group selected 24 h postinhalation as the primary endpoint for assessment of antidepressant effects. TRMD patients enrolled in the trial suffered from sustained and severe refractory MDD: patients had a mean of 19 years of lifetime MDD, history of 8 average failed antidepressant treatments, and 4 had a history of failing to respond to ECT.
The results of the pilot trial were promising. At 24 h postinhalation, there was a statistically significant reduction in depressive symptoms in those who had received nitrous oxide versus placebo. Those receiving nitrous oxide experienced a mean decrease of 5.5 points on the Hamilton Depression Rating Scale (HDRS; 95 CI − 2.5 to − 8.5 points) at 24 h. There were 4 TRMD patients achieving antidepressant response (≥ 50% reduction from baseline HDRS score) and one achieving remission at 24 h; whereas only one placebo patient achieved response, and no placebo patients achieved remission ( ).
Interestingly, not all antidepressant symptoms improved equally, i.e., certain depressive symptoms appeared to be more responsive than others to nitrous oxide, including anxiety, depressed mood, suicidal ideation, and guilt, which has directed future studies (see “ Future studies of nitrous oxide ” section below). Similar to the observations of ketamine’s rapid antidepressant effects in TRMD, the effects of a single inhalation of nitrous oxide were frequently sustained; several patients who were randomized to receive nitrous oxide first showed sustained antidepressant effects on assessment at 1 week. Hence, this prompted future cross-over studies of nitrous oxide in TRMD having longer durations between exposures.
Also of importance, nitrous oxide was well-tolerated; the side effects were infrequent, minor, and short-lived. These included nausea and vomiting (15%), headache (10%), and paradoxical anxiety (10%) in a small subset of patients.
Most recently, conducted a double-blind, randomized, cross-over dose finding study of nitrous oxide. In this trial, 24 patients with severe TRD were randomly assigned to receive a one-hour inhalation with either (1) 50% nitrous oxide, (2) 25% nitrous oxide, or (3) placebo (air/oxygen). The primary outcome was the Hamilton Depression Rating Scale (HDRS-21). Both nitrous groups combined demonstrated significant improvement versus placebo (P = 0.01); however, there was no acute difference in antidepressant effects between 25% and 50% nitrous oxide (P = 0.58). However, adverse events (nausea, headache, dissociative feeling) were statistically higher in the 50% group (P < 0.001). There was a suggestion that 50% nitrous may have had more sustained antidepressant benefit. Further study of optimal dose finding is warranted.
Though limited in scope, this study (and our group’s continued studies in this area) support that nitrous oxide may have several advantages over ketamine as a rapid acting antidepressant. Unlike ketamine, existing data do not support emergence of psychosis as a common side effect, and nitrous oxide inhalation does not influence systemic blood pressure. Though nitrous oxide does have limited abuse potential (see ” Abuse potential of nitrous oxide ” section below), it has considerably less addictive potential than ketamine. Last, given that nitrous oxide is not hepatically or renally metabolized, it is rapidly cleared from the system, allowing patients to safely operate motor vehicles following treatments, whereas ketamine has a markedly longer recovery time and requires a driver postadministration.
Clinical administration of nitrous oxide for TRMD
From a regulatory standpoint, nitrous oxide is not regulated by the Drug Enforcement Agency. Occupational Safety and Health Administration (OSHA) does not have standards for nitrous exposure. The National Institute for Occupational Safety and Health, NIOSH, however, has a recommended exposure limit of 25 ppm in closed rooms.
Potential effects on psychomotor and cognitive function in health care workers leads to the need of minimizing leaks in gas delivery circuits and adequate exhaust through wall-suction waste gas scavenging systems. Closely fitting masks minimize leakage around the face. Even with a closed system, nitrous oxide is not effective as a single-agent general anesthetic.
Medical grade nitrous oxide can be acquired from gas distributors as E -cylinder tanks that can be dispensed through FDA-approved inhalation circuits or anesthesia ventilators. Nitrous oxide and oxygen/air flow meters allow accurate dispensing. Proportioning systems link the flows of oxygen and nitrous oxide to prevent the administration of mixtures that would induce hypoxia. Infrared spectroscopic gas analyzers and infrared pulse oximeters allow additional safety monitoring for detecting potential hypoxemia. Suction and airway equipment should be readily available. Staff trained in administering sedatives and airway support ensure safety, as per American Society of Anesthesia guidelines on sedation and analgesia by nonanesthesiologists.
Patient preparation and selection may minimize the risk of adverse events even for short inhalations. Fasting guidelines should be considered to minimize the possibility of aspiration events. A history of motion sickness or postoperative vomiting may portend susceptibility to the side effect of nausea. Respiratory depressant effects of narcotics and sedatives are augmented by nitrous oxide. As nitrous oxide accumulates in tissues with collected air, it is contraindicated in patients with pneumothorax, pneumomediastinum, or gastrointestinal distention. Patients with B12 deficiency or particular mutations in the methylenetetrahydrofolate reductase ( MTHFR ) gene should be reconsidered given the inhibition of methionine synthase by nitrous oxide.
With adherence to these considerations the clinical administration of nitrous oxide can be administered safely for TRMD.
Putative potential mechanism of action of nitrous oxide in TRMD
Despite over 150 years of clinical use for analgesia and anesthesia, how nitrous oxide brings about its effects is not fully understood. Below we discuss possible mechanisms of action.
Effects on the NMDAR
Extensive research over the past 20 years has conclusively demonstrated that nitrous oxide acts as a noncompetitive inhibitor of NMDARs by blocking NMDAR responses at approximately a half-maximal effective concentration of 30%–40%; however, nitrous oxide is only a partial NMDAR antagonist at concentrations up to 80% ( ; ; ; ). The effects of nitrous oxide do not alter the decay of NMDAR-mediated currents, and are weakly voltage dependent ( ). Additionally, work in animal models provides supporting evidence that nitrous acts in vivo via NMDAR-antagonistic behavioral effects ( ; ). Further, nitrous oxide also acts as a weak inhibitor of AMPA/kainite-type glutamate receptors.
Other nitrous oxide receptor interactions
In addition to acting on the NMDAR, nitrous oxide is known to act on numerous other receptors, including GABA-A, GABA-C, serotonin-3, potassium and calcium channel receptors, nicotinic acetylcholine receptors, alpha-adrenergic receptors, and opioid receptors.
Nitrous oxide, unlike many other anesthetic agents, has weak potentiating effects at the GABA-A receptor ( ), while weakly inhibiting the GABA-C receptors ( ). Nitrous oxide inhibits low voltage activated (LVA, T-type) calcium channels, producing depression of LVA currents at 80% nitrous oxide ( ), without having an effect on the high voltage activated (L-type) calcium currents ( ). Further, nitrous oxide is a weak antagonist of serotonin-type 3 receptors, and partially inhibits a subtype of nicotinic acetylcholine receptors ( ). Nitrous oxide is an agonist at the two-pore domain potassium channel (TREK-1), which is expressed throughout the central nervous system ( ), as well as activating alpha-adrenergic receptors associated with antinociceptive effects in the brainstem and spinal cord in rat models ( ; ).
Finally, nitrous oxide appears to have modulatory effects on the endogenous opioid system, which could be linked to both analgesia and psychotropic effects ( ; ; ; ). This is potentially highly relevant in TRMD, as evidence suggests that ketamine has diminished antidepressant effects when combined with an opioid antagonist ( ). Existing evidence supports that nitrous oxide may preferentially act on kappa-type opiate receptors over mu-type receptors ( ; ).
Nitrous oxide effects on brain circuits
There is currently limited understanding of the effects of nitrous oxide on brain pathways. Animal models suggest that nitrous oxide significantly impacts the hippocampus: nitrous oxide brings about disinhibition of network-driven hippocampal spike firing ( ). Another study demonstrated that nitrous oxide can increase cellular proliferation in dentate gyrus, possibly via neurogenesis ( ).
Limited electroencephalography studies in humans demonstrated that nitrous oxide decreases functional connectivity in superficial parietal networks ( ) and leads to an increase in theta activity and a decrease in frontal slow wave activity (delta) with drug discontinuation ( ). How these changes are associated with proposed behavioral changes is not yet understood.
Abuse potential of nitrous oxide
There is longstanding history of recreational use of nitrous oxide, with descriptions of “laughing gas parties” during the Victorian era ( ), and recreational use which continues to the present. Currently, recreational nitrous oxide use typically involves inhalation with either bulbs or balloons and is primarily limited to teens and young adults, with an estimated prevalence of 20% in this age group ( ). Contributing to the sustained popularity of recreational nitrous oxide is its wide availability, low cost, and relative safety. Though there are reports of psychological dependence and tolerance ( ), such cases are relatively rare, with most recreational users not developing physical or psychological dependence: over 90% report using once per month or less frequently ( ).
Acute effects include rapid onset of euphoria, excitement, and dissociation, typically resolving within minutes. Though relatively rare, long-term abuse of nitrous oxide can be associated with permanent neurological damage, including spinal cord degeneration ( ), which is thought to be brought about in part by nitrous oxide-induced vitamin B12 inactivation ( ). Other known safety concerns previously associated with nitrous oxide use include dyspnea, headache, impaired judgment, and even impaired fertility with repeated exposure ( ). Further, high concentration inhaled nitrous oxide (> 70%) can lead to hypoxia and associated sequalae. As the use of nitrous oxide expands for the TRMD indication, greater awareness of this abuse potential and associated dangers will be necessary.
Future studies of nitrous oxide
Along with the exciting emergence of another potentially efficacious rapid-acting antidepressant, many questions remain. Similar to studies demonstrating reduced suicidal ideation using ketamine, demonstrated that in addition to rapidly decreasing depressive symptoms, nitrous oxide also lowered suicidal ideation, prompting current investigation of antisuicidal properties. Further, consistent with animal studies demonstrating the NMDAR antagonism occurring at nitrous oxide concentrations far below the heretofore tested concentration of 50% ( ), dose-finding studies in patients with TRMD are currently underway. Additionally, more precise functional brain imaging studies to test the acute and chronic effects of nitrous oxide along with elucidating mechanism of action, on both nondepressed controls and TRMD patients should be undertaken.
Isoflurane
Introduction
Isoflurane can be dosed to induce cortical burst suppression on the electroencephalogram. The burst suppression state has been likened to the transient postictal generalized electroencephalographic suppression seen after electroconvulsive therapy (ECT). Initial clinical trials suggest that burst suppression anesthesia has antidepressant activity similar to ECT while avoiding associated adverse effects which limit its use. The use of anesthetic induced burst suppression with isoflurane (or propofol, see below) is an area of active interest and investigation.
Pharmacology
Isoflurane is a halogenated methyl ethyl ether compound and isomer of enflurane. It was approved for use in 1979. Its anesthetic effects are largely attributed to GABA-A agonism but other pharmacological effects have been reported including NMDA antagonism ( ). Isoflurane is well-tolerated and, relative to previously developed inhaled anesthetics, it demonstrates cardiovascular safety and decreased renal and hepatic toxicity ( ). Of particular interest, isoflurane produces predictable, well-characterized, dose-related changes on the electroencephalogram (EEG) ( ). At high doses, isoflurane administration is associated with EEG burst suppression—a state during which bursts of activity alternate with periods of quiescence. For isoflurane, this burst suppression pattern initially occurs at approximately 1.5 MAC and progresses to form a near isoelectric pattern at approximately 2 MAC. As discussed below, this burst suppression activity may have therapeutic effects in TRMD.
Clinical studies
Anesthetic-induced cortical burst suppression was first suggested as a potential alternative to ECT in the 1980s. observed that after an ECT-induced seizure, patients experienced a transient period (i.e., minutes) of postictal suppression on EEG. They speculated that a seizure was not necessary for antidepressant efficacy, but rather the induction of EEG suppression was more important to ECT’s therapeutic effects. In support of this idea, postictal suppression has been suggested to be at least as predictive of ECT efficacy as seizure duration ( ; ). Therefore, they hypothesized that isoflurane-induced burst suppression (described as “isoflurane narcotherapy”) could potentially provide similar antidepressant efficacy to ECT while avoiding seizure-associated adverse effects, which include headache, body aches, and cognitive impairment.
published the first open-label trial of isoflurane-induced burst suppression (ISO) for TRMD in which significant reduction of depressive symptoms was experienced by 9 of 11 patients. Subsequent open-label work by others also suggested that ISO may have similar antidepressant therapeutic efficacy to ECT ( ; ). reported the results of a small double-blind randomized controlled trial comparing the antidepressant efficacy of six ISO treatments ( n = 9) to six ECT treatments ( n = 10) in women. The ISO group was treated to a “predominant burst suppression pattern” on EEG and maintained for 15 min. Patients were treated twice a week. Assessments were done prior to each treatment, at series end, and 2 weeks posttreatment series. ISO patients showed a significant and superior antidepressant response to ECT. At series end, six of nine showed a clinically significant (> 50% improvement) response and three of nine achieved remission. Effects were durable, and at the 2-week follow-up, there were six of nine responders and five of nine remitters. ISO patients suffered no adverse cognitive effects, but rather demonstrated neuropsychological improvements across several domains. In contrast, the ECT cohort patients showed significant cognitive deficits.
Not all burst suppression anesthesia studies have reported similar impressive antidepressive outcomes. treated six elderly depressed patients (including five aged 74–82 years), in an open-label pilot study, with little reported improvement. Predictably, hypotension was an issue for these elderly ISO-treated patients and two patients (both with past medical histories of hypertension and one with coronary artery disease) were not able to complete the series. reported a small randomized trial of sevoflurane, a gas anesthetic related to isoflurane in which subjects underwent four 1-h sevoflurane-induced burst suppression treatments ( n = 9) versus four sham treatments (induced using propofol but allowed to awaken) ( n = 7). In this study, two of the nine sevoflurane patients showed more than 50% decrease in depression scores, and two more showed between 25% and 50% improvement. A 24% decrease in depression scores was observed, but given the small sample size, this result did not reach statistical significance. Of interest, however, for those responding to treatment, the effects lasted for at least for 1–3 months. There are several methodological considerations worth noting in this trial. First, sevoflurane was administered at lower relative concentration [average sevoflurane 2.5% (~ 1.25 MAC) versus isoflurane 2.5% (~ 2 MAC) maintenance]. Second, sevoflurane, even when administered at equipotent doses, is likely less effective than isoflurane at inducing burst suppression ( ). Third, patients received only four treatments versus six for Langer and 8–12 for a typical ECT series. While patients showed improvement in the sevoflurane study, it is not surprising that the antidepressant effects were smaller than those reported for ISO. As a result of these mixed and negatively interpreted data, there was a pause in related work for a number of years.
Recently, encouraging studies by a number of groups have renewed interest in burst suppression anesthesia for TRMD. reported the first preclinical experiment of ISO’s antidepressant-like effects. In that study, the forced swim test (FST) was used. In the FST, mice are placed in an inescapable cylinder of water, and efforts to escape are quantified over 6 min. Immobility in the FST is interpreted as a measure of behavioral despair. Conversely, increased efforts to escape are associated with antidepressant efficacy ( ). In Tadler’s study at 24 h post-ISO treatment, mice showed increased mobility relative to controls and comparable to the behavioral effects of a conventional antidepressant, desipramine. subsequently showed antidepressant-like effects in rodents treated with isoflurane (2% for 30 min) assessed both acutely, using the FST, and 6 days postexposure, in a test of learned helplessness. reported that isoflurane dosed to achieve burst suppression (3% induction and 2% maintenance for 2 h) prevents the development of learned helplessness in rats and reverses it in mice. Interestingly, halothane, which fails to induce burst suppression even at high doses, shows no antidepressant effect with equivalent high-dose administration.
Given the initially encouraging results of burst suppression anesthesia, methodological differences between Langer’s and later work, and supportive animal work, a small clinical trial was performed, similar to Langer’s initial work ( ). In the study, eight patients were treated open-label with 10 sessions ISO and compared to 20 patients treated naturalistically with bifrontal ECT. ISO was dosed to produce a predominant burst suppression pattern on cortical EEG (burst suppression ratio > 80%) for 15 min per session. ISO showed an antidepressant response (> 50% improvement in Hamilton depression score) in 75% of patients and an identical rate of full remission as ECT (50%). Improvement was durable and continued to the 4-week follow-up for most patients.
Adverse effects and toxicology
No serious adverse events have been reported in clinical trials of isoflurane-induced burst suppression. reported that while ECT led to temporary declines in memory and cognitive function, ISO patients actually improved in many neuropsychological domains. Expected dose-dependent decreases in blood pressure were the most significant effect of isoflurane administration. Hypotension was managed with intravenous isotonic fluid coadministration, Trendelenburg positioning, and intermittent phenylephrine boluses as necessary. Treatments were well tolerated by patients with minimal associated nausea and vomiting facilitated by the use of a proprietary isoflurane adsorption device used at emergence.
Summary and future directions
In summary, for isoflurane, there is preliminary support, both preclinical and clinical, that ISO has rapid, robust and durable antidepressant effects and lacks ECT-associated adverse effects. Patients awaken alert, without confusion. It also lacks the psychotomimetic side effects associated with ketamine. Whether the induction of burst suppression using ISO or other anesthetics will ultimately provide a viable alternative to ECT with its undesirable seizure-associated adverse effects remains to be definitively shown. A well-powered blinded randomized placebo-controlled trial of isoflurane anesthesia for TRMD has yet to be completed.
While current evidence suggests that isoflurane doses adequate to induce burst suppression may be necessary to realize an antidepressant effect, the optimal dosing, duration, and number of treatments is not known. Post-ECT cortical suppression lasts only a few minutes. noted antidepressant effects with as little as 5 min of suppression in open-label studies, but 15 min was targeted in the RCT. aimed for a 1 h burst suppression treatment interval, administered fewer treatments, used a lower relative dose, and did not observe as robust effects as others. , using isoflurane, more closely replicated Langer’s work with similar outcomes. Animal studies have shown effects with varied doses and durations of isoflurane. Clarifying the optimal dose, duration, and frequency of treatments will be important in future investigations, as will elucidating associated molecular mechanisms.
Subsequent to isoflurane studies, an open-label trial has provided the first evidence that the induction of burst suppression using another anesthetic agent, propofol, may have antidepressant effects similar to ECT ( ). As of 2020, ongoing work (led by SCT and BJM) is testing the antidepressant effects of deep propofol anesthesia in a double-blind, randomized, controlled trial.
If anesthetic drugs do indeed have clinical antidepressant effects, a better understanding of their mechanisms will be an important step in refining these interventions. Rantamaki et al. have recently suggested that ketamine, nitrous oxide, and ECT may act by similar mechanisms to initiate synaptic changes that result in antidepressant efficacy ( ). EEG delta wave prominence in the early posttreatment period are associated with immediate early gene transcription and activation of BDNF-TrkB signaling. Identification of translational biomarkers like this, which can be investigated in humans and animal models, will be critical for understanding therapeutic mechanisms and developing optimized interventions.
Xenon
Xenon is a noble gas with anesthetic, analgesic, neuroprotective, and cardioprotective properties. Xenon has been proposed as a therapeutic agent for depression and other psychiatric conditions when administered at subanesthetic doses. As of late 2020, no definitive evidence of antidepressant effects in humans has yet been reported.
Chemistry
This unique gas is a mono-atomic gas element that exists in the atmosphere in trace concentrations. Xenon is manufactured through the distillation of liquefied air ( ). Like other noble gases, xenon does not react with other chemicals under ordinary conditions.
Pharmacology
Mechanisms of action
Despite the fact that xenon is chemically nonreactive, it is biologically active. Xenon inhibits NMDA receptor function by binding the glycine site, and this mechanism of action is thought to mediate its hypnotic and neuroprotective effects ( ; ; ). Direct actions at other glutamate receptors, potassium channels, and other targets have also been reported ( ). Along with nitrous oxide and ketamine, xenon is one of the few anesthetics that do not act directly at GABA receptors ( ).
Pharmacokinetics
The minimum alveolar concentration (MAC) for surgical anesthesia has been estimated between 51% and 71%, with lower values in women, and xenon’s MAC-awake is ~ 33% ( ). Both onset and offset of action are more rapid than other inhaled agents ( ; ). Because xenon is chemically nonreactive, it is excreted via the lungs without biotransformation by renal or hepatic systems ( ; ).
In addition to the inhalation route, xenon has been administered intravenously and intraperitoneally to experimental animals. The gas can be dissolved in saline ( ) or encapsulated in liposomes which can be subsequently uncaged using ultrasound ( ). Oral formulations of xenon have been developed for administration to humans ( ).
Indications
The anesthetic properties of xenon were discovered more than 70 years ago ( ) but only in the 2000s did xenon receive regulatory approval as an anesthetic agent in Europe and Russia ( ). Although not yet an approved anesthetic in the United States, certain radioisotopes of xenon (e.g., 133 Xe) are approved by the FDA as nuclear medicine contrast agents.
Clinical studies
General efficacy profile
Pivotal clinical trials have demonstrated the efficacy of xenon as a general anesthetic along with its potential cardioprotective and neuroprotective benefits ( ; ).
Efficacy for treatment-resistant depression
Several recent investigations have examined the antidepressant-like and anxiolytic-like effects of xenon in rodents. An early behavioral study using a rat model of posttraumatic stress disorder found that administration of subanesthetic xenon gas interfered with reconsolidation of fear memories ( ). administered xenon-containing liposomes intravenously to male rats and applied ultrasound over the carotid artery to uncage the xenon gas. Antidepressant-like effects were found at 6 mg/kg but not 9 mg/kg. administered xenon gas at a subanesthetic dose to rats and found improvements in some anxiety-like and social behaviors but no significant antidepressant-like effects. Finally, administered subanesthetic xenon gas via inhalation or xenon-rich saline intraperitoneally to male mice and found antidepressant-like and anxiolytic-like effects at 30 min.
Xenon is being studied as a potential treatment for major depression, panic disorder, and posttraumatic stress disorder, but few clinical studies have yet been published. reported an open-label clinical trial of subanesthetic xenon for panic disorder. Eighty-one patients received a series of 6 treatments, each lasting up to 4 min. The inhaled xenon/oxygen mixture was titrated from 15%/85% to 30%/70% over 9 days. Scores on the anxiety subscale of the Hospital Anxiety and Depression Scale decreased by ~ 60% on average after 6 treatments, improvements on the depression subscale were reported, and treatments were generally well-tolerated (6% dropout). More recently, reported a blinded, randomized controlled, cross-over trial of xenon versus placebo in 14 healthy men. Participants received a single treatment with either xenon/nitrogen/oxygen (25%/45%/30%) or nitrogen/oxygen (70%/30%) for 15 min. Self-reported positive emotions increased with xenon in a subset of participants when assessed 12 min after drug discontinuation. Beneficial effects of xenon for major depressive disorder have been described in abstract form ( ), but a full report has not been published as of late 2020.
Adverse effects and toxicology
Compared to volatile anesthetics and propofol, postoperative nausea and vomiting are more common with xenon ( ), but xenon provides better hemodynamic stability and faster recovery ( ; ). Studies to date indicate that xenon is nontoxic and, in fact, can be organoprotective ( ; ).
Summary and future directions
Xenon is an NMDA receptor antagonist that holds potential as a treatment for depression and other neuropsychiatric disorders. Preclinical studies indicate this agent has antidepressant-like and anxiolytic-like effects in some assays. No controlled clinical trials of xenon’s antidepressant or antianxiety effects have yet appeared in the literature.
As of late 2020, several active clinical trials of xenon are registered in the United States and European Union for indications that include major depressive disorder, bipolar disorder, posttraumatic stress disorder, and panic disorder.
The use of inhalants in complimentary medicine: Aromatherapy
Within the past 25 years, there has been a significant increase in the use of complimentary alternative medicine (CAM) techniques to attempt to treat depression. Broadly defined, CAM is a collection of healing resources (such as medical practices and products) for the prevention, diagnosis, and treatment of diseases, which are complimentary to mainstream medicine ( ). The use of CAM in the treatment of depression has become widespread: recent estimates support that CAM is employed by approximately 54% of depressed patients ( ).
Emerging from the CAM field in the past 30 years is the use of aromatic compounds, most especially “essential oils” in the treatment of depression. Aromatherapy is the therapeutic use of plant-derived highly concentrated oils ( ; ). Currently, there is no standardization of dosage or dilution used; hence, it is difficult to do comparative studies ( ).
Though to date these compounds have not been systematically targeted in TRMD, recent controlled trials have attempted to verify the antidepressant efficacy in different depressed populations, particularly in patient groups for whom standard antidepressant treatments are potentially unsafe or poorly tolerated (e.g., pregnancy, cancer patients). For a recent summary of these findings, see .
Currently, there are four controlled studies of aromatherapy in MDD ( ; ; ; ), and of these four studies, only one reported antidepressant efficacy ( ; ).
Summary and future directions
The field of CAM, and aromatherapy in particular, is only recently undergoing careful scientific inquiry. Given the widespread use of these treatments and the relative potential advantages (sensitive populations, safety in combination with other treatments), future carefully designed study of these treatments is critical. The current use aromatherapy for MDD currently suffers from a lack of standardization of dosing, delivery methods, and frequency of treatments; all areas that would benefit from additional research.
References
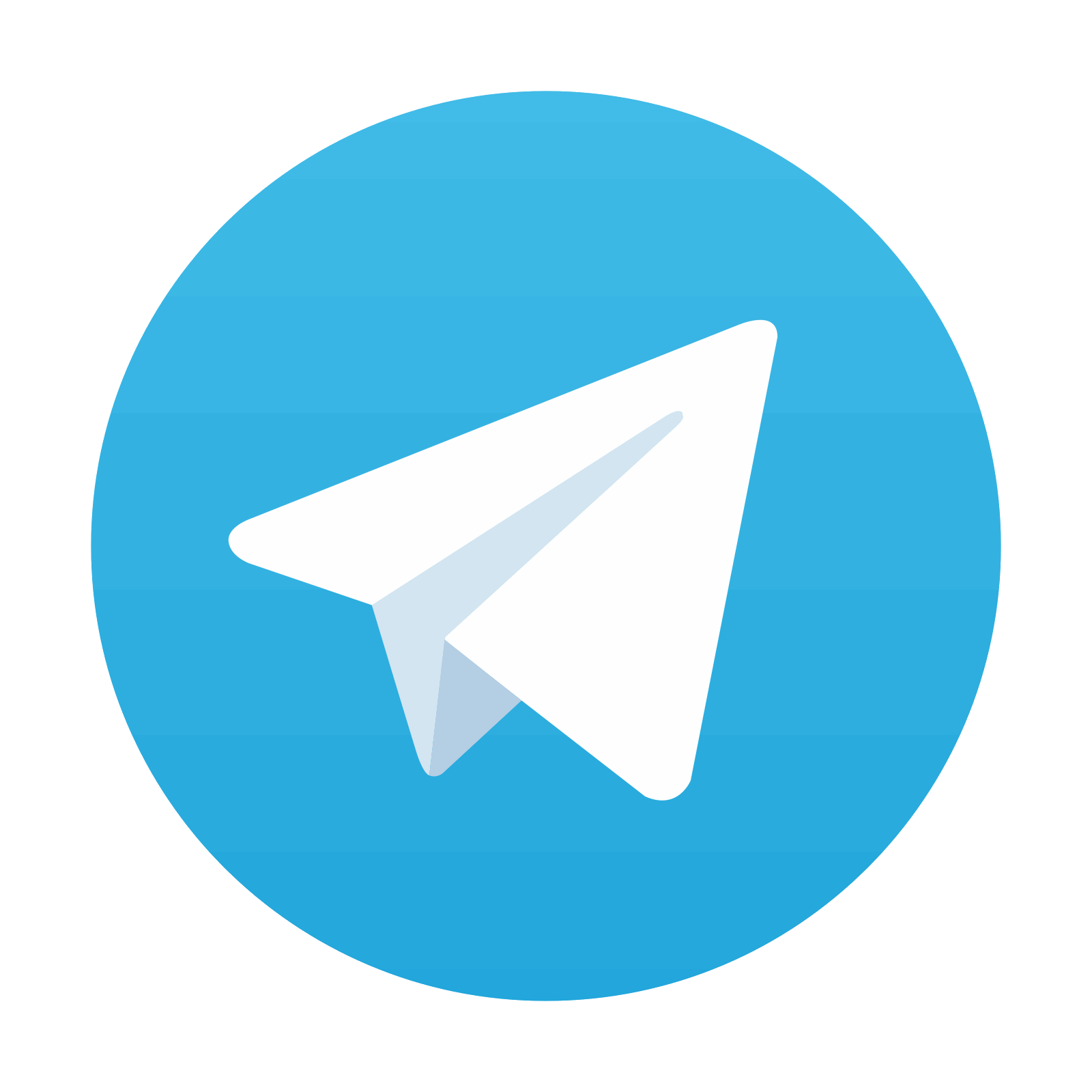
Stay updated, free articles. Join our Telegram channel

Full access? Get Clinical Tree
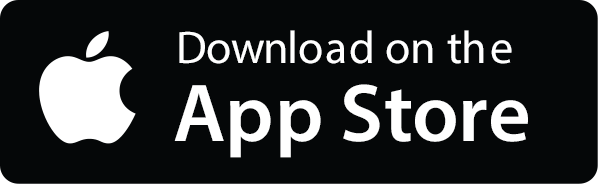
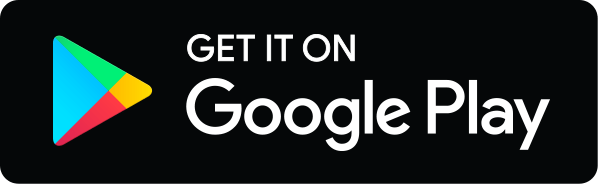
