Inherited Metabolic Disorders
Main Text
Preamble
Inherited metabolic disorders—a.k.a. inborn errors of metabolism—represent conditions in which a genetic defect leads to a deficiency of a protein (e.g., enzyme or nonenzyme protein) that subsequently affects mechanisms of synthesis, degradation, transport, &/or storage of molecules in the body. Inherited metabolic disorders can present at virtually any age from infancy well into the fifth and sixth decades, although infantile and childhood presentation are most common.
Inherited metabolic disorders (IMDs) are relatively uncommon diseases. The informed radiologist can merge their understanding of the pathogenetic and pathomorphologic underpinnings of the various IMDs with imaging observations. Specifically, we observe what part of the brain is involved[e.g., gray matter (GM) vs. white matter (WM)], what kind of involvement is present (e.g., cortex, basal ganglia, WM—subcortical, deep, or periventricular), and what locations are most affected (e.g., frontal lobes). Additional information, such as the presence of cysts, calcifications, diffusion restriction, and pathologic enhancement, aids in crystallizing the imaging differential diagnosis.
Because WM abnormalities form a constant part of many, if not most, inborn metabolic diseases, familiarization with the normal progression of WM myelination is a prerequisite for detecting and understanding IMDs. We therefore begin this chapter with a brief review of how normal myelination progresses from birth through two years of life.
Once we have reviewed the patterns of normal myelination as assessed with MR, we continue with an overview and introduction of the IMDs. A discussion of classification systems and a practical approach to analyzing imaging is delineated. A detailed discussion of the leukodystrophies and nonleukodystrophic WM disorders is beyond the scope of this text, but selected IMDs representative of their groupings will be presented.
Normal Myelination and White Matter Development
General Considerations
Myelination
Myelination is an orderly, highly regulated, multistep process that begins during the fifth fetal month and is largely complete by 18-24 postnatal months. Some structures (e.g., cranial nerves) myelinate relatively early in fetal development, whereas others (e.g., optic radiations and fibers to/from association areas) often do not completely myelinate until the third or even the fourth decade of life.
Brain myelination follows a typical topographic pattern, progressing from inferior to superior, central to peripheral, and posterior to anterior. For example, the brainstem myelinates before the peripheral cerebellar hemispheres, the posterior limbs of the internal capsules myelinate before the anterior limbs, and the deep periventricular WM myelinates before the subcortical U-fibers. The dorsal brainstem myelinates before the anterior brainstem, and—with the exception of the parietooccipital association tracts—occipital WM myelinates earlier than WM in the anterior temporal and frontal lobes.
CT
At birth, the WM is largely unmyelinated, so it appears symmetrically hypoattenuating compared with regional GM due to the comparatively high water content of unmyelinated WM.
MR
The MR appearance of WM maturation varies with two important factors, i.e., patient age and the imaging sequence employed. Unmyelinated WM is hypointense relative to GM on T1WI and hyperintense to GM on T2WI.
As WM matures, it becomes more hyperintense on T1WI. Progressive T2 shortening (hypointensity) occurs in the first two years of life as myelin matures and proton density decreases.
During the first 6-8 months, T1 hyperintensity occurs earlier and is more conspicuous than T2 hypointensity, so T1WIs are best to evaluate both WM maturation and brain morphology (36-1) (36-2). Heavily weighted T2 sequences are sensitive to follow WM maturation between 6-18 months. Fully myelinated WM has high T1 signal and low T2 signal.
Selected major milestones of normal myelination on T1- and T2-weighted images are summarized in the accompanying table (Table 36-1).
Classification of Inherited Metabolic Disorders
Overview
An exhaustive discussion of IMDs is far beyond the scope of this book. The interested reader is referred to the superb definitive texts by A. James Barkovich. In this chapter, we consider the major inherited neurometabolic diseases, summarizing the pathoetiology, genetics, demographics, clinical presentation, and key imaging findings of each.
There are several strategies that can be used to conceptually frame IMDs. One way is to divide IMDs according to which cellular organelle (e.g., mitochondria, lysosomes) is predominantly affected. Another characterizes them by defects in a specific metabolic pathway (e.g., disorders of carbohydrate metabolism). However, these methods lack the pragmatic approach needed by the radiologist to be a contributing member of the clinical care team. We therefore emphasize—and advocate the use of—an approach to imaging analysis pioneered by A. James Barkovich that is primarily based on anatomic location and specific imaging features with an emphasis on MR—the imaging-based approach.
Organelle-Based Approach
Three cellular organelles are primarily affected in IMDs, i.e., the lysosomes, peroxisomes, and mitochondria. Classifying IMDs according to the affected organelle has the benefit of conceptual simplicity, but many IMDs do not arise from disordered organelle formation or function, making this classification scheme less than comprehensive. The organelle-based approach to IMDs is summarized in the accompanying box.
ORGANELLE-BASED CLASSIFICATION OF INHERITED METABOLIC DISORDERS
Lysosomal Disorders
• Mucopolysaccharidoses
• Gangliosidoses
• Metachromatic leukodystrophy
• Krabbe disease
• Fabry disease
Peroxisomal Disorders
• Abnormal peroxisomal formation
Zellweger syndrome
Neonatal adrenoleukodystrophy
Infantile Refsum disease
• Abnormal peroxisomal function
X-linked adrenoleukodystrophy
Classic Refsum disease
Mitochondrial Disorders
• Leigh syndrome
• Mitochondrial encephalomyopathy with lactic acidosis and stroke-like episodes (MELAS)
• Myoclonic epilepsy with ragged red fibers (MERRF)
• Kearns-Sayre
• Glutaric aciduria types 1 and 2
Imaging-Based Approach
Barkovich et al have elaborated a practical imaging-based approach to the diagnosis of IMDs derived from the seminal work of van der Knaap and Valk. This approach is based on determining whether the disease involves primarily or exclusively (1) WM, (2) mostly GM, or (3) both. Furthermore, a heightened awareness of the region of the brain most heavily involved (e.g., periventricular WM vs. subcortical WM or frontal lobe vs. parietal occipital lobes) and the presence of miscellaneous findings (e.g., cysts &/or calcifications) leads to greater specificity.
In this text, we follow the imaging-based approach, the clinically practical classification based on three categories of predominant imaging features (e.g., WM, GM, or both being involved). We then discuss the major diagnostic entities in each imaging-based group.
Inherited Metabolic Disorders Predominantly Affecting White Matter
Preamble
Historically, nearly all abnormalities of the WM have been described as “leukodystrophies.”Leukodystrophies have been divided into three categories: (1) Dys myelinating disorders (i.e., normal myelination does not occur), (2) de myelinating disease (i.e., myelin forms normally, is deposited around axons, but later breaks down or is destroyed), and (3) hypo myelinating diseases (i.e., here, the WM may partially myelinate but never myelinates completely). Hypomyelinating leukoencephalopathies represent an important yet uncommon group of genetic disorders that cause delayed myelin maturation or undermyelination.
From an imaging perspective, it can be difficult to determine whether a disorder is dys myelinating, de myelinating, or hypo myelinating. In structuring a differential diagnosis, it is important to determine whether the disorder primarily affects deep (periventricular) WM or the subcortical short association WM fibers (U-fibers). In a few diseases, both the deep and peripheral WM are affected.
Examples of leukodystrophies that exhibit early deep WM predominance include metachromatic leukodystrophy (MLD) and X-linked adrenoleukodystrophy (X-ALD). Leukodystrophies that involve the subcortical U-fibers early in the disease course include megaloencephalic leukoencephalopathy with cysts and infantile Alexander disease (AxD). The latter two diagnoses also present with a large head.
Diseases in which virtually all the WM (both periventricular and subcortical) remains unmyelinated are rare. The imaging appearance in these disorders resembles that of a normal newborn brain with immature, almost completely unmyelinated, WM. Here, the entire WM—including the subcortical U-fibers—appears uniformly hyperintense on T2WI.
Hypomyelinating Disorders
The list of hypomyelinating disorders is long and beyond the scope of this book, but it is worth being aware of the imaging findings that may accompany such disorders. The prototypical hypomyelinating disorder is Pelizaeus-Merzbacher disease (PMD), which may result in complete lack of myelination or hypomyelination, depending on severity.
Pelizaeus-Merzbacher Disease
Etiology
Caused by a mutation in PLP1, which codes for proteolipid protein 1 and DM20, two primary components of myelin.
Clinical Issues
The classic form is X-linked. A more severe “connatal” form presents earlier and generally has a more severe clinical course. Patients usually present in the first year of life. Symptoms include nystagmus, delayed cognitive and motor development, and ataxia.
Imaging
CT typically shows decreased attenuation throughout the WM.
MR shows absent or significantly reduced myelination for age with lack of bright T1 signal and dark T2 signal that would be expected with normal myelination (36-3). In milder forms, there may be some degree of myelination in the internal capsules and optic radiations. Cerebellar atrophy may be present.
Differential Diagnosis
Primary differential diagnosis includes other hypomyelinating disorders, such as 18q syndrome, fucosidosis, and Tay syndrome, among others. In very young patients, it is important to know the gestational age so as not to mistake the normal brain appearance of prematurity for lack of myelination.
Periventricular White Matter Predominance
The prototypical disorder that typically begins with symmetric deep WM involvement and spares the subcortical U-fibers until late in the disease course is MLD. Others with a similar pattern of periventricular predominance include Krabbe disease (globoid cell leukodystrophy), X-ALD, and vanishing WM disease (VWMD).
MAJOR INHERITED METABOLIC DISORDERS WITH PERIVENTRICULAR WHITE MATTER PREDOMINANCE
Common
• Metachromatic leukodystrophy
• Classic X-linked adrenoleukodystrophy
Less Common
• Globoid cell leukodystrophy (Krabbe disease)
• Vanishing WM disease
Rare but Important
• Phenylketonuria
• Maple syrup urine disease
• Merosin-deficient congenital muscular dystrophy
Metachromatic Leukodystrophy
Etiology
MLD is a devastating lysosomal storage disease caused by a reduction in or complete absence of arylsulfatase A (ARSA). Reduced or absent ARSA leads to increased lysosomal storage of sulfatide and, eventually, lethal demyelination.
Pathology
Sulfatide deposition occurs within glial cells, plasma membranes, inner layer of myelin sheath, neurons, Schwann cells, and macrophages. The periventricular WM shows a grayish discoloration (e.g., tigroid or leopard pattern) with relatively normal-appearing subcortical U-fibers.
Clinical Issues
MLD is one of the most common of all inherited WM disorders. Three distinct clinical forms are currently recognized: Late infantile (onset earlier than three years), juvenile (onset earlier than 16 years), and adult MLD. The late infantile form is the most common and typically presents in the second year of life with visuomotor impairment, gait disorder, and abdominal pain. Progressive decline and death within four years are expected. The juvenile form presents between 5-10 years, often with deteriorating school performance. Survival beyond 20 years is rare. The adult form may present with early-onset dementia, MS-like symptoms, and progressive cerebellar signs.
Imaging
CT Findings
Early NECT shows symmetric diminished attenuation involving the central hemispheric WM (36-4).
MR Findings
The typical MR features of early MLD are confluent, symmetric, butterfly-shaped T2/FLAIR hyperintensities involving the periventricular WM (36-8). The subcortical U-fibers and cerebellum are typically spared until late in the disease.
With disease progression, centrifugal spread of demyelination involves the corpus callosum (i.e., splenium), parietooccipital WM, and the frontal and then the temporal WM. Islands of normal myelin around medullary veins in the WM may produce a striking tiger, tigroid, or leopard pattern with linear hypointensities in a sea of confluent hyperintensity (36-5) (36-6). WM signal abnormalities show no enhancement. Another important finding of MLD is cranial nerve enhancement, which is often striking (36-7).
METACHROMATIC LEUKODYSTROPHY
Etiology and Pathology
• Lysosomal storage disorder
• Decreased ARSA → sphingolipid accumulation
• Periventricular demyelination
Clinical Issues
• Most common inherited leukodystrophy
• 3 forms
Late infantile (most common)
Juvenile
Adult (late onset)
Imaging
• Centrifugal spread of demyelination
Starts in corpus callosum splenium, deep parietooccipital white matter
Frontal, temporal white matter affected later
Spares subcortical U-fibers, cerebellum
• Classic = butterfly pattern
Symmetric hyperintensities around frontal horns, atria
• Tiger pattern
“Stripes” of perivenular myelin sparing in white matter
Differential Diagnosis
• Other disorders that predominantly affect periventricular white matter
Globoid cell leukodystrophy (Krabbe disease)
Pelizaeus-Merzbacher disease
Vanishing white matter disease
• Destructive disorders
Periventricular leukomalacia
Miscellaneous Imaging
Reduction of diffusivity in zones of active demyelination is seen. Regions of “burned-out,” aging or chronic demyelination demonstrate increased diffusivity. MRS is nonspecific; choline and myoinositol may be elevated in early and active disease.
Differential Diagnosis
The major differential diagnosis of MLD includes other IMDs that primarily affect the periventricular WM. Globoid cell leukoencephalopathy (Krabbe disease)shows bithalamic hyperattenuation on NECT, involves the cerebellum early, and often demonstrates enlarged optic nerves and optic chiasm.
PMD usually presents in neonates and shows almost a total lack of myelination that does not show interval improvement on serial MRs. The cerebellum may be markedly atrophic.
Periventricular WM injury (PVL)is associated with a history of low-birth-weight/preterm deliveries and clinical static spastic di- or quadriparesis and shows nonprogressive periventricular volume loss and T2/FLAIR hyperintensity.
VWMD begins in the periventricular WM but eventually involves all of the hemispheric WM. VWMD often cavitates and does not enhance.
X-Linked Adrenoleukodystrophy
Etiology
X-ALD is an inherited disorder of peroxisomal metabolism. Absent or deficient acyl-CoA synthetase leads to impaired oxidation of very-long-chain fatty acids (VLCFAs). VLCFAs accumulate in the WM, causing severe inflammatory demyelination (“brittle” myelin). Axonal degeneration in the posterior fossa and spinal cord are also typical of the disease. The definitive diagnosis of X-ALD is established by tissue assays for increased amounts of VLCFAs.
Pathology
Three distinct zones of myelin loss are seen in adrenoleukodystrophy (ALD) (36-9A). The innermost zone consists of a necrotic core of demyelination with astrogliosis, ± calcification. An intermediate zone of active demyelination and perivascular inflammation lies just outside the necrotic, “burned-out” core of the lesion. The most peripheral zone represents the advanced edge of ongoing demyelination without inflammatory changes.
ADRENOLEUKODYSTROPHY: ETIOLOGY, PATHOLOGY, AND CLINICAL ISSUES
Etiology
• Peroxisomal disorder
• Impaired oxidation of very-long-chain fatty acids
Pathology
• Severe inflammatory demyelination
• 3 zones
Necrotic, “burned-out” core
Intermediate zone of active demyelination + inflammation
Peripheral demyelination without inflammation
Clinical Issues
• Classic X-linked adrenoleukodystrophy
Most common form (45%)
Preteen boys
Deteriorating cognition, school performance
• Adrenomyeloneuropathy
2nd most common form (35%)
Most common in male patients
• Addison disease without CNS involvement (20%)
Clinical Issues
X-ALD is the most common single protein or enzyme deficiency disease to present in childhood. Several clinical forms of ALD and related disorders have been described. Classic X-ALD is the most common form (45%) and is seen almost exclusively in boys 5-12 years of age. Behavioral difficulties and deteriorating school performance are common. Approximately 10% of affected patients present acutely with seizures, adrenal crisis, acute encephalopathy, or coma.
Adrenomyeloneuropathy (AMN) is the second most common type (35%). It is another X-linked disorder that occurs primarily in male patients. It presents between 14-60 years, thus presenting later than classic X-ALD. AMN is characterized by axonal degeneration in the spinal cord more than the brain and peripheral nerves.
Imaging
Although CT scans are sometimes obtained as an initial screening study in children with encephalopathy of unknown origin, MR ± IV contrast is the procedure of choice.
CT Findings
NECT scans demonstrate hypoattenuation involving the corpus callosum splenium and WM around the atria and occipital horns. Calcification in the affected WM may be seen. CECT typically shows enhancement around the central hypoattenuating WM.
MR Findings
A posterior-predominant pattern is seen in 80% of patients with X-ALD (36-9). The earliest finding is T2/FLAIR hyperintensity in the middle of the corpus callosum splenium. As the disease progresses, hyperintensity spreads from posterior to anterior and from the center to the periphery. The peritrigonal WM, corticospinal tracts, fornix, commissural fibers, + the visual and auditory pathways can all eventually become involved.
The leading edge of demyelination appears hyperintense on T1WI but does not enhance. The intermediate zone of active inflammatory demyelination typically enhances on T1 C+(36-9C).
X-ALD may present at an atypical age, demonstrate atypical sites of involvement, and lack enhancement. Approximately 10-15% of all patients with classic X-ALD have an anterior-predominant demyelination; T2/FLAIR hyperintensity initially appears in the corpus callosum genu (not the splenium) and spreads into the frontal lobe WM (36-10).
In patients with AMN, the cerebral hemispheres are relatively spared with predominant involvement of the cerebellum, corticospinal tracts, and spinal cord. Enhancement is typically absent.
DWI/DTI shows reduced diffusivity in active zones of demyelination and increased diffusivity in regions of “burned-out” demyelination. DTI shows reduced connectivity (i.e., loss of fractional anisotropy) in WM that MR demonstrates as abnormal and in “normal” WM.
MRS shows, at TE of 35 ms, peaks at 0.8-1.4 (i.e., cytosolic amino acids and VLCFA macromolecules). Reduced N-acetyl-L-aspartate (NAA) may be detected prior to observed MR abnormality and predicts progression. Increased myoinositol, choline, and lactate doublet are typical findings.
Differential Diagnosis
When X-ALD presents in patients of classic age and sex (i.e., 5- to 12-year-old boys) and with typical posterior predominance on imaging studies, the differential diagnosis is very limited.
ADRENOLEUKODYSTROPHY: IMAGING AND DIFFERENTIAL DIAGNOSIS
Imaging
• X-linked adrenoleukodystrophy posterior predominance in 80%
Earliest finding: Corpus callosum splenium hyperintensity
Spreads posterior to anterior, center to periphery
Intermediate zone often enhances, restricts
• Variant patterns
X-linked adrenoleukodystrophy with anterior predominance (10-15%)
Adrenomyeloneuropathy involves corticospinal tracts, cerebellum, cord more than hemispheric white matter
Differential Diagnosis
• X-linked adrenoleukodystrophy pathognomonic if sex, age, imaging findings classic
Vanishing White Matter Disease
Vanishing WM disease (VWMD) has become recognized as one of the most prevalent inherited leukoencephalopathies. VWMD is characterized by diffusely abnormal cerebral WM that literally “vanishes” over time. VWMD is an autosomal recessive disorder caused by point mutation of genes responsible for encoding any of the five subunits of the eukaryotic translation inhibiting factor 2B (EIF2B).
Pathology
VWMD is a slowly progressive, eventually cavitating WM disease that predominately involves the deep frontoparietal with lesser involvement of the temporal lobes. The basal ganglia, corpus callosum, anterior commissure, internal capsules, and cortex are characteristically spared. The eventual appearance is areas of cystic degeneration with frank WM cavitation.
Clinical Issues
Classic VWMD presents in children 2-5 years of age. Development is initially normal, but progressive motor and cognitive impairment with cerebellar and pyramidal signs follow. Progression is typically slow. Death by adolescence is typical.
Approximately 15% of VWMD cases occur in adolescents and adults. Mean age of late-onset VWMD is 30 years.
Imaging
WM hypoattenuation without calcifications is typical in the early stages of VWMD (36-12B). Eventually, profound WM volume loss is seen (36-12C).
Extensive confluent WM T1 hypointensity with T2/FLAIR hyperintensity is typical. The disease is initially periventricular but later spreads to involve the subcortical arcuate fibers. Over time, the affected WM undergoes rarefaction. Cavitary foci of CSF-like signal intensity eventually develop (36-12C). Diffuse volume loss with enlarged ventricles and sulci is seen on serial studies. DWI typically shows diffusion restriction in active areas of WM destruction and facilitated diffusion in the CSF-like cavitary areas (36-12A). VWMD does not enhance.
Differential Diagnosis
VWMD is not the only leukoencephalopathy that causes “melting away” or “vanishing” of the cerebral WM. AxD and mitochondrial encephalopathies can be associated with WM rarefaction and cystic degeneration. AxD presents with macrocephaly and is not associated with the episodic neurologic deterioration characteristic of VWMD; it demonstrates a frontoparietal gradient of disease. Frontal WM cysts can occur in end-stage disease. Approximately 10% of mitochondrial encephalopathies predominantly affect the WM and may form cavitations.
Phenylketonuria
Phenylketonuria (PKU) is the most common inborn error of amino acid metabolism. Phenylalanine (Phe) accumulates and is toxic to the developing brain. Newborns are usually asymptomatic and most cases are diagnosed with newborn metabolic disease-screening programs. With adherence to dietary protein restriction, mitigation of the ravages of this disease occurs. Early treatment is key to minimizing cognitive impairment.
Initial imaging in PKU can appear normal! When abnormality is detected, T2/FLAIR imaging shows hyperintensity in the periventricular WM, particularly frontal and peritrigonal regions (36-13). The subcortical arcuate fibers are spared. There is no enhancement following contrast administration. Centrifugal progression eventually leads to end-stage disease. MRS shows a Phe peak resonating at 7.37 ppm (which is missed on routine clinical MRS that only displays to 4 ppm).
The differential diagnoses include PVL (at-risk population of low-birth-weight, premature neonates who eventually manifest signs and symptoms of cerebral palsy, MLD (demonstrating more confluent zones of deep WM T2/FLAIR hyperintensity), and Krabbe disease (optic and cranial nerve enlargement, thalamic hyperattenuation on NECT, MR showing T2/FLAIR hyperintensity in corticospinal tracts, deep cerebral WM, and early cerebellar involvement).
Maple Syrup Urine Disease
Maple syrup urine disease (MSUD) is an autosomal recessive disorder of branched-chain amino acid (leucine, isoleucine, valine) metabolism. Elevated levels of leucine and other leukotoxic metabolites induce cytotoxic or intramyelinic edema and spongiform degeneration.
Infants with classic MSUD are initially normal. Within days after birth, poor feeding, lethargy, vomiting, seizures, and encephalopathy may occur. In severe cases, the urine smells of maple syrup or burned sugar.
NECT scans show profound hypoattenuation within the myelinated WM as well as within the dorsal brainstem, cerebellum, cerebral peduncles, and posterior limb of the internal capsule (36-14). MR shows striking T2/FLAIR hyperintensity involving the cerebellar WM, dorsal brainstem, cerebral peduncles, thalami, globi pallidi (GP), posterior limbs of internal capsule, internal medullary lamina, and pyramidal and tegmental tracts.
MRS shows a peak at 0.9 ppm caused by accumulation of branched-chain α-keto amino acids. This peak is present at short (35 ms), intermediate (144 ms), and long (288 ms) PRESS MRS acquisitions, distinguishing them from the broad cytosolic amino acid peaks that only resonate at a short TE (35 ms).
The differential diagnosis includes AxD (T2 hyperintensity of the frontal WM and enhancement), hypoxic-ischemic injury (HIE) (with history of periparturitional distress, no significant symptom-free period), and mitochondrial cytopathy (episodic stroke-like clinical events of varied severity depending on the genotype of the disorder).
Subcortical White Matter Predominance
IMDs that initially or predominantly affect the subcortical WM are much less common than those that begin with deep periventricular involvement. The most striking IMD with preferential involvement of the subcortical WM is megaloencephalic leukoencephaly with subcortical cysts (MLC).
Megaloencephalic Leukodystrophy With Subcortical Cysts
MLC, a.k.a. vacuolating megaloencephalic leukoencephalopathy, is a rare autosomal recessive disorder with characteristic MR features.
Pathology, Clinical Features
Gross pathology shows a swollen cerebral hemispheric WM, relative occipital sparing, variable involvement of the subcortical arcuate fibers, frequent involvement of the external capsules, and sparing of the internal capsules. Multiple variably sized subcortical cysts, initially involving the temporal lobes, are typical (36-15)(36-16).
MLC is distinguished clinically from other leukoencephalopathies by its remarkably slow course of neurologic deterioration. Infantile-onset macrocephaly is characteristic, but neurologic deterioration is often delayed. Age at symptom onset varies widely, ranging from birth to 25 years. Slow cognitive decline is observed as the disease progresses.
Imaging
Cranial US in symptomatic infants shows unexplained hyperechogenicity of affected WM. NECT will demonstrate hypoattenuation of the cerebral hemispheric WM.
The diagnosis of MLC is typically established by MR. Macrocephaly with diffuse confluent WM T2/FLAIR hyperintensity in the subcortical WM is typical. The affected subcortical WM appears “watery” and swollen. The overlying gyri seemingly stretch over the swollen WM. The optic radiations, occipital subcortical WM, corpus callosum, as well as the basal ganglia and internal capsule, are usually spared.
Characteristic CSF-like subcortical cysts develop in the anterior temporal lobes followed in frequency by frontoparietal cysts. Unlike the “watery” WM, which exhibits T2/FLAIR hyperintensity, the cysts approximate the signal intensity of CSF on FLAIR. The number and size of the cysts may increase over time. The abnormal WM and cysts do not enhance on T1 C+.
Differential Diagnosis
MLC must be distinguished from other IMDs with macrocrania. The two major considerations are Canavan disease (CD) and AxD, both of which are characterized by much greater clinical disability.
CD almost always involves the basal ganglia, lacks the development of subcortical cysts, and demonstrates a large NAA peak on MRS. CD also shows very early involvement of the subcortical U-fibers, which may appear uninvolved in the early presentation of MLC.
AxD demonstrates a frontal WM gradient, involves the basal ganglia, and often enhances following contrast administration.
Inherited Metabolic Disorders Predominantly Affecting Gray Matter
Preamble
Inherited metabolic disorders (IMDs) that involve the gray matter (GM) without affecting the white matter (WM) can be subdivided into those that involve the cortex and those that mostly affect the deep gray nuclei. Inherited GM disorders that involve the deep gray nuclei are significantly more common than those that primarily affect the cortex.
Inherited Metabolic Disorders Primarily Affecting Deep Gray Nuclei
A number of inherited disorders affect mostly the basal ganglia and thalami. Three inborn errors of metabolism with specific predilection for the deep gray nuclei include (1) pantothenate kinase-associated neurodegeneration (PKAN), (2) creatine deficiency syndromes, and (3) a cytosine-adenine-guanine (CAG) repeat disorder called Huntington disease (HD).
We begin this section with an overview of brain iron accumulation disorders before turning our attention specifically to PKAN and HD. We close the section with a discussion of the most important inherited disorder with abnormal copper metabolism, Wilson disease (WD) .
Brain Iron Accumulation Disorders
Some iron accumulation within the basal ganglia and dentate nuclei occurs as part of normal aging (see Chapter 38). Neurodegeneration with brain iron accumulation (NBIA) represents a clinically and genetically heterogeneous group of conditions characterized by progressive neurodegeneration and abnormally elevated brain iron. We focus our discussion on PKAN, the most common type of NBIA, and HD.
MR is especially useful in diagnosing NBIAs. All feature iron deposition in the GP but differ in other associated findings. The distribution of T2 or T2* hypointensity can help distinguish between the different NBIA subtypes (see accompanying box).
NBIA: T2* HYPOINTENSITY
Pantothenate Kinase-Associated Neurodegeneration
• Globus pallidus, substantia nigra, dentate nuclei
• Eye of the tiger sign variable
• Spares cortex
Infantile Neuroaxonal Dystrophy
• Cerebellar atrophy (95%)
• T2* hypointensity in globus pallidus, substantia nigra (50%)
• Spares cortex
Neuroferritinopathy
• T2* hypointensity in globus pallidus, substantia nigra
• Then dentate/caudate nuclei, thalami
• Affects cortex
Aceruloplasminemia
• Globus pallidus, caudate nuclei, putamen, thalamus
• Red nucleus, substantia nigra, dentate nuclei
• Affects cerebral, cerebellar cortices
Pantothenate Kinase-Associated Neurodegeneration
Terminology and Etiology
PKAN, formerly known as Hallervorden-Spatz disease, is a rare familial autosomal recessive disorder characterized by excessive iron deposition in the GP and substantia nigra (SN).
Pathology
Grossly, PKAN is characterized by shrinkage and rust-brown discoloration of the medial GP, the reticular zone of the SN, and, sometimes, the dentate nuclei. The red nuclei are generally spared. Microscopically, increased iron content is found in the GP interna and pars reticulata SN. Iron deposition is found within astrocytes, microglial cells, neurons, and around vessels. The “eye of the tiger” corresponds to regions of reactive astrocytes, dystrophic axons, and vacuoles in the anteromedial GP.
Clinical Issues
PKAN, or NBIA type 1, can develop at any age. Most cases are diagnosed late in the first decade or during early adolescence. The disorder classically begins with slowly progressive gait disturbances and delayed psychomotor development. Progressive mental deterioration finally leads to dementia.
Imaging
Imaging findings reflect the anatomic distribution of the excessive iron accumulation. T2WI demonstrates marked hypointensity in the GP and SN. A small focus of central hyperintensity in the medial aspect of the very hypointense GP (the classic eye of the tiger sign) is caused by tissue gliosis and vacuolization (36-17). Note that not all cases of PKAN demonstrate the “eye of the tiger”(36-18).
PKAN does not enhance on T1 C+ nor does it demonstrate restricted diffusion.
Differential Diagnosis
Abnormal iron deposition in the basal ganglia occurs with PKAN as well as other NBIAs. Aceruloplasminemia and neuroferritinopathy are both adult-onset disorders. Both involve the cortex, which is spared in PKAN.
Disorders with increased T2 signal within the GP can be grouped within metabolic derangements and toxic/ischemic insults. These entities lack pallidal GRE and SWI “blooming.”
IMDs to consider in the differential diagnosis of basal ganglia T2 hyperintensity include neuroferritinopathy (variable GP T2 hyperintensity), WD, Leigh syndrome, infantile bilateral striatal necrosis, and mitochondrial encephalopathies, which show striatal hyperintensity (not hypointensity or “blooming”). These disorders predominantly involve the caudate and putamen, not the medial GP.
Toxic/ischemic insults include HIE (positive health history, T2 hyperintensity involving striatum, GP, thalami, corticospinal tracts, ± cortical involvement), CO poisoning (increased T2 signal involving GP, other deep nuclei, cortex, and WM), cyanide toxicity (T2 increased within basal ganglia ± hemorrhagic necrosis), and kernicterus (neonate) (increased T1/T2 GP).
Huntington Disease
Terminology and Etiology
HD is an autosomal dominant chronic hereditary neurodegenerative disorder with complete penetrance. The huntingtin gene includes a repeating CAG trinucleotide segment of variable length. The presence of > 38 repeats confirms the diagnosis of HD.
Pathology
The most characteristic gross abnormality is volume loss with rarefaction of the caudate nucleus, putamen, and GP (36-19) (36-20). The cerebellum is also atrophic in juvenile-onset HD.
Clinical Issues
Mean age at symptom onset is 35-45 years. Only 5-10% of patients present before the age of 20 years (juvenile-onset HD). CAG repeat length and age influence both the expression and the progression of HD.
Adult-onset HD is characterized by progressive loss of normal motor function, development of stereotypic choreiform movements, and declining cognition. Once symptoms appear, HD typically results in death within 10-20 years.
HUNTINGTON DISEASE
Etiology
• Autosomal dominant, complete penetrance
• CAG trinucleotide repeat disorder
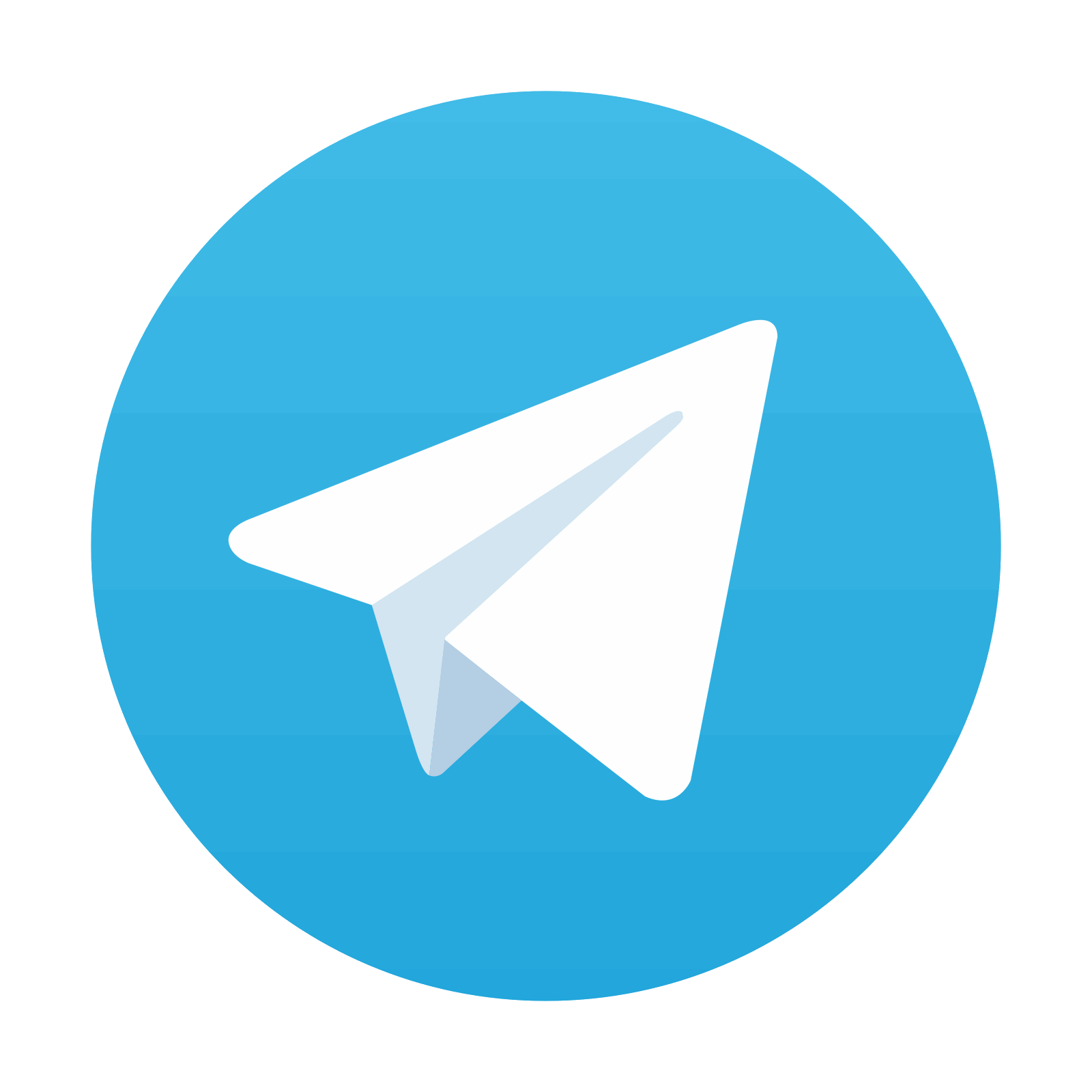
Stay updated, free articles. Join our Telegram channel

Full access? Get Clinical Tree
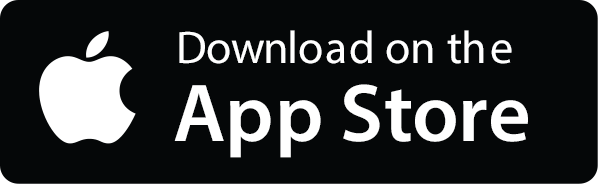
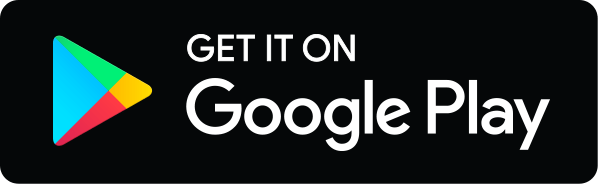