Understanding the inherited neurodegenerative diseases of childhood has evolved dramatically in the past 40 years. Fundamental discoveries in biochemistry uncovered the metabolic basis for many of these diseases. The subsequent identification of variant forms created additional challenges for the clinician. These variant forms share similar, if not identical, biochemical defects, but exhibit widely differing clinical expressions. Recent progress followed the rapid development and application of molecular-genetic strategies and the close linkage between clinical observations and basic science advances. The successes of molecular genetics have provided significant clarifications, but have also generated further examples of the marked heterogeneity within these disorders. These advances explain how disorders with clearly different phenotypic expression can result from different mutations within the same gene and, conversely, how disorders with similar clinical features result from mutations in different genes. Examples of the former include GM2-gangliosidosis variants and examples of the latter include the neuronal ceroid lipofuscinoses. Thus, at the clinical, biochemical, and molecular level, the clinician is faced with an increasingly complex knowledge base, the unraveling of which may present major diagnostic hurdles.
Inherited neurodegenerative diseases represent a significant proportion of referrals to tertiary care centers, especially those specializing in the care of children. For all inherited neurometabolic diseases as a group, the overall incidence is about 1 in 3700 births, and for those neurodegenerative disorders considered here, incidence may approach 1 in 5000 births. Technological advancements have in no way diminished the need for careful characterization of the clinical phenotype. Rather, enhanced diagnostic capabilities raise important public health policy issues including development of the necessary infrastructure to monitor outcome of diagnosis and treatment and to analyze the overall impact on society.
Regardless of the biochemical and molecular mechanisms, common patterns of disease expression emerge according to age at onset. Disorders presenting in infancy and early childhood are characterized by delayed development or loss of acquired developmental milestones that follows a period of delay or plateau in development. Onset in school-aged children or adolescents is characterized by declining school performance and behavioral or personality changes. In adulthood, faltering personal or work habits suggest declining cognitive abilities, movement disorder, or gait difficulties. As such, clinical assessment should include historical information regarding changes in development or behavior, evidence of cognitive difficulties, and altered motor control and a careful family history including consanguinity, previous deaths in childhood or adolescence, early-onset dementia, or a diagnosis of progressive cerebral palsy. Progressive neurological manifestations fall outside the established pathogenesis for cerebral palsy. A thorough clinical examination should incorporate assessment for dysmorphic features, hepatosplenomegaly, or skeletal deformities, and careful neurological and ophthalmologic evaluations, the latter for abnormalities of voluntary eye movement, corneal clouding, lens opacities, and retinal abnormalities such as retinitis pigmentosa, macular degeneration, cherry-red spot maculae, and optic atrophy.
Neurodegenerative disease may be explained by a number of processes that should be excluded including other metabolic conditions such as diabetes mellitus or porphyria, toxic encephalopathies (heavy metal poisoning, antiepileptic or neuroleptic preparations, or drugs of abuse), chronic viral infections (subacute sclerosing panencephalitis and the AIDS complex group), immunopathic disorders (systemic lupus erythematosis and related conditions), intracranial neoplasms, and psychiatric or behavioral disorders including autistic spectrum disorder and environmental deprivation.
Laboratory diagnosis involves a variety of biochemical, enzymatic, and molecular methodologies as well as clinical neurophysiology, neuroimaging, and histopathology. Careful clinical assessment should allow a quite precise tailored approach. However, for this to occur, a high index of suspicion for an inherited neurodegenerative process is essential.
In sum, the inherited neurodegenerative diseases represent important opportunities for advancement of knowledge, but at the same time present significant challenges to the neurologist in providing timely diagnosis, appropriate family counseling, and the consideration of new therapeutic strategies.
This chapter explores four groups of inherited neurodegenerative diseases presenting in childhood: the sphingolipidoses, the adrenoleukodystrophy complex, the neuronal ceroid lipofuscinoses, and the sialidosis/sialuria complex.1,2 Clinical heterogeneity is summarized and current approaches to diagnosis and treatment examined. In the past, these disorders have been classified in discrete forms according to age at onset and clinical severity. We now recognize that age at onset and clinical severity fall along a clinical continuum. Detailed descriptions of these diseases can be found elsewhere.
The sphingolipidoses, adrenoleukodystrophy complex, neuronal ceroid lipofuscinoses, and sialidosis/sialuria complex include disorders affecting both neuronal and glial function.1,2 Within each group, the principal clinical variants will be discussed in terms of age at onset, clinical features, prognosis, mode of inheritance, stored material when relevant, and enzyme defects when known. Variant forms that may begin in adolescence or adulthood are included for comparison.
The sphingolipidoses (Table 24-1) represent a model for understanding the inherited neurodegenerative diseases of children, encompassing within the respective disorders both neuronal storage and white matter involvement.3-11 The sphingolipids are normal lipid constituents of biological membranes. The sphingolipidoses reflect accumulation of the disease-related sphingolipid within the affected organ(s) as the result of defective catabolism. Catabolism of sphingolipids occurs within lysosomes by specific acid hydrolases (lysosomal hydrolases). With the exception of galactosylceramide lipidosis (Krabbe disease), the disease-related sphingolipid accumulates up to 100-fold that seen in normal tissue. Accumulation of the sphingolipid, galactosylceramide, does not occur in Krabbe disease, except within globoid bodies, the neuropathologic hallmark of this disease. In contrast, galactosylsphingosine (psychosine), the deacylated form of galactosylceramide and the principal cytotoxic component of Krabbe disease does accumulate.
Disease | Onset Age | Clinical Features | Longevity | Inheritance |
---|---|---|---|---|
Sphingolipidoses | ||||
GM2-Gangliosidosis | 6-12 mo | Psychomotor decline Seizures Cherry-red maculae | Death by age 5 | AR |
Juvenile type | 2-5 y | Seizures Gait difficulty Psychomotor decline | Death by age 15 | AR |
Adult type | Adolescence to adulthood | Spinocerebellar degeneration Distal muscle wasting Dystonia Intact mentation | Slow progression usual | AR |
Sandhoff type | 6-12 mo | Hepatosplenomegaly Psychomotor decline Seizures Cherry-red maculae | Death by age 5 | AR |
GM1-Gangliosidosis (Generalized gangliosidosis) | ||||
Type I—Infantile | Infancy | Hurler-like appearance Hepatomegaly Psychomotor decline | Death by age 2 | AR |
Type II—Late infantile | 6-12 mo | Psychomotor decline | Death in 3-10 y | AR |
Type III—Juvenile | 5 y | Ataxia Dysarthria Mild dementia | Prolonged survival | AR |
Type IV—Adult | Adolescence to adulthood Gait difficulty Variable dementia | Dystonia Bradykinesia | Prolonged survival | AR |
Globotriaosylceramidelipidosis (Fabry disease) | Adolescence to adulthood | Cutaneous angiokeratoma Painful neuropathy Heat intolerance Renal disease Cardiac/CNS disease | Middle age | XLR |
Sphingomyelin Lipidosis (Niemann-Pick Disease) | ||||
Type A–Infantile (85% of total) | Infancy | Hepatosplenomegaly Failure to thrive Psychomotor decline | Death by age 4 | AR |
Type B—Adult (10%-15% of total) | Childhood | Hepatomegaly Pulmonary infections | Prolonged survival | AR |
Type C—Late infantile | 1-6 y | Initial hepatosplenomegaly, then gait difficulty Psychomotor decline | Death in teens | AR |
Type D—Nova Scotia Glucosylceramide Lipidosis (Gaucher Disease) | 1-6 y | Similar to type III | Death in teens | AR |
Type I—Adult (80% of total) | By teens | Hepatosplenomegaly | Prolonged survival | AR |
Type II—Infantile (15% of total) | Infancy | Hepatosplenomegaly Laryngospasm Extensor posturing Psychomotor decline | Death by age 2 | AR |
Type III—Juvenile (Norrbotten type) | 4-8 y | Initial hepatosplenomegaly Seizures Dementia Motor difficulties | Death by age 10-15 with some prolonged | AR |
Galactosylceramide Lipidosis (Krabbe Disease) | ||||
Type I—Infantile | Infancy | Irritability Extensor posturing Optic atrophy Cortical blindness Psychomotor deterioration | Death by age 12 mo | AR |
Type II—Late infantile | 1-3 y | Gait difficulty Psychomotor decline Visual failure | Death by age 10-12 | AR |
Sulfatide Lipidosis (Metachromatic Leukodystrophy) | ||||
Type I—Late infantile | 6-24 mo | Gait difficulty Ataxia Hypotonia | Death in 5-6 y | AR |
Type II—Juvenile | 4-8 y | Gait difficulty Ataxia prominent Psychomotor decline | Death in 10-15 y | AR |
Type III—Adult | 15 y or older | Dementia Depression Psychotic behavior Motor difficulty | Slow progression usual | AR |
Type IV—Mucosulfatidosis | 6–18 mo | Hepatosplenomegaly Skeletal deformity, Psychomotor decline | Death by age 10-12 | AR |
Ceramide Lipidosis (Farber Disease) | Early infancy | Subcutaneous granuloma Painful, fixed joints Failure to thrive Hypotonia | Death by age 2 | AR |
Adrenoleukodystrophy Complex | ||||
Adrenoleukodystrophy | 4-15 y | Behavioral difficulty Poor school performance Quadriparesis Seizures Visual decline Hypoadrenalism in ~50% | Death in 6-10 y from onset | XLR |
Adrenomyeloneuropathy | 20-40 y | Spastic paraparesis Urinary retention Impotence Distal neuropathy Hypoadrenalism from childhood | Prolonged survival | XLR |
Adrenoleukomyeloneuropathy | 10-20 y | Behavioral difficulty Pyramidal tract and cerebellar difficulty Pseudobulbar palsy Mild dementia Hypoadrenalism | Death in 10 y | XLR |
Neonatal adrenoleukodystrophy | Infancy | Severe hypotonia Seizures Profound psychomotor decline Ultimately blind and deaf Normal adrenal function Hypoplastic adrenals Optic atrophy or dysplasia | Death in 1-5 y | AR |
Neuronal Ceroid Lipofuscinoses | ||||
Infantile type (Haltia-Santavuori) | 6 mo-2 y | Myoclonus Seizures Blindness Profound psychomotor decline | Death by age 10 | AR |
Late-infantile type (Jansky-Bielschowsky) | 2-4 y | Seizures Dementia Visual disturbance later | Death by age 10-20 | AR |
Juvenile type (Spielmeyer-Vogt) | 5-10 y | Early visual loss Seizures and dementia later | Death by age 20 | AR |
Sialidosis/Sialuria Complex | ||||
Sialidosis I | Adolescence | Cherry-red maculae Myoclonus Gait difficulties and ataxia Normal intellect common Visual acuity decline | Prolonged survival | AR |
Sialidosis II | ||||
Infantile type (Mucolipidosis I) | Infancy | Seizures Myoclonus Hurler-like coarse features Corneal clouding Cherry-red maculate Hepatosplenomegaly Growth failure Profound psychomotor delay | Death by age 5 | AR |
Juvenile type | 5–15 y | Myoclonus Ataxia Coarse features Psychomotor delay Cherry-red maculae Visual acuity decline | Death in adolescence to young adulthood | AR |
Sialuria Complex | ||||
Infantile type | At birth | Profound psychomotor delay Coarse features Hepatosplenomegaly Sparse white hair Diarrhea Anemia | Death by age 2 | AR |
Salla type | 1 y | Psychomotor delay Coarse features Ataxia Athetosis Quadriparesis | Prolonged survival | AR |
The GM2 gangliosidoses (Box 24-1) are generally classified as acute (infantile), subacute (juvenile), and chronic (adult) types according to age at onset (Table 24-1). The majority manifest the acute-onset form, that is, classic Tay-Sachs disease. At least 10 clinical phenotypes are recognized,12 each caused by deficiency of hexosaminidase A, except in the case of Sandhoff disease and its variant forms that feature deficiencies of both hexosaminidase A and B (Hex A and Hex B), or the very rare form caused by deficiency of the GM2 activator protein. Tay-Sachs disease has been recognized in several population isolates, including Ashkenazi Jews, French-Canadians, Pennsylvania Dutch, and Cajuns (from Acadia). The incidence of Tay-Sachs disease is approximately 1 in 4000 among Ashkenazi Jews and about 1 in 200,000 in the general population. Only Hex A metabolizes GM2 ganglioside. Hex B metabolizes the neutral sphingolipid, globoside, in addition to the other compounds noted above. Children with GM2 activator deficiency resemble Tay-Sachs disease clinically. They have normal Hex A and Hex B activity, but lack the activator protein required for metabolism of GM2 ganglioside.
|
Acute GM2 gangliosidosis type I (Tay-Sachs disease) has its onset in infancy, following uneventful early development, marked by increasing irritability, seizures, exaggerated startle response, and rapidly progressive psychomotor deterioration. Weakness, hypotonia, and hyporeflexia appear initially and are gradually replaced by spasticity and extensor posturing. Seizures include repetitive generalized myoclonic jerks of the trunk and extremities. EEG may reveal hypsarrhythmia or multifocal cortical spike discharges and may be associated with laughter (gelastic seizures). Neuroimaging techniques demonstrate hyperdense cerebral cortical zones, decreased white matter, ventricular dilatation, and small cerebellum and brainstem. Electroretinography (ERG) is normal, but VERs are delayed. Death occurs by age 5. Cherry-red maculae, reflecting storage material within retinal ganglion cells and obscuring the choroidal vessels, were once considered a hallmark of the disease. They may also appear in other storage diseases, including GM1 gangliosidosis, metachromatic leukodystrophy, sphingomyelin lipidosis, and sialidase deficiency. Cherry-red maculae are easily detected at the onset, but may be obscured during later stages, so their absence does not rule out the diagnosis. Macrocephaly, if present, is due to gliosis and not GM2-ganglioside storage.
GM2 gangliosidosis type II (Sandhoff disease) differs clinically from type I by the presence of hepato-splenomegaly and biochemically by deficiency of both hexosaminidase A and B. GM2 ganglioside accumulates within the central nervous system and globoside, the principal glycosphingolipid of red cells, accumulates in visceral organs. The clinical onset, pattern, and progression of type II GM2 gangliosidosis are similar to Tay-Sachs disease. Death occurs by age 5.
Subacute (Juvenile) forms of Tay-Sachs and Sandhoff diseases present between 2 and 5 years as gait disturbance with ataxia and psychomotor deterioration.13 Neither cherry-red maculae nor hepatosplenomegaly are noted. Progression is relentless, with death occurring by adolescence.
Chronic forms of GM2 gangliosidosis present a variety of neurological manifestations, typically appearing in adolescents and adults, reflecting dysfunction of specific neuronal populations including anterior horn cells, brainstem, basal ganglia, thalamus, and cerebellum.14,15 These include spinal muscular atrophy, motor neuron disease, spinocerebellar degeneration, and progressive dystonia. Neurological involvement of one system often dominates, but significant overlap may occur. Mentation is generally preserved although progressive dementia or significant psychiatric manifestations ranging from depression to schizophrenia may occur.16,17 Most chronic forms of GM2 gangliosidosis appear to be due to deficiency of Hex A.
Brain weight and volume increase markedly secondary to gliosis during the second year of life, often exceeding 2000 g (normal = 1000 g). Cystic degeneration of the cerebral white matter, along with atrophy of the cerebellar hemispheres, is frequently present. Neurons, including anterior horn cells, and glia store GM2 ganglioside. Myenteric plexus ganglion cells are also ballooned and distorted. Historically, rectal biopsy was performed as a diagnostic procedure for the storage diseases, but is obsolete due to the availability of biochemical and molecular diagnostic methodologies. Electron microscopy reveals lipid profiles within neuronal cytoplasm (most likely within lysosomes) referred to as membranous cytoplasmic bodies. In late-onset GM2 gangliosidosis, storage is preferentially localized to thalamus, substantia nigra, brainstem nuclei, and cerebellum.
GM2 ganglioside accumulates in this group of disorders in neurons in the central, peripheral, and autonomic nervous systems (Table 24-2). Asialo-GM2-ganglioside (GM2 ganglioside minus sialic acid) and lyso-GM2 (GM2 ganglioside minus the fatty acid) also accumulate. The lyso-compound may be cytotoxic. Hex A activity is deficient in tissues of patients with Tay-Sachs disease and its variant forms, although total hexosaminidase activity may be increased. Hex A and B activities are deficient in Sandhoff disease and its variants. Diagnosis of each form of GM2 gangliosidosis can be made in leukocytes or cultured skin fibroblasts. In GM2 activator deficiency, Hex A and B activities are normal versus synthetic substrate, but GM2 metabolism is deficient in the absence of the activator. Multiple mutations in the respective genes have been identified.18
Disease | Stored Material | Enzyme Defect | Tissue for Diagnosis |
---|---|---|---|
Sphingolipidoses | |||
GM2-Gangliosidosis | GM2-ganglioside | Hexosaminidase A | Leucocytes |
Asialo GM2-analogue | Cultured skin fibroblasts | ||
GM2-Gangliosidosis | GM2-ganglioside | Hexosaminidase A and B | Leucocytes |
Sandhoff Type | Asialo GM2-analogue Globoside in non-neural organs | Cultured skin fibroblasts | |
GM1-Gangliosidosis | GM1-ganglioside | GM1-ganglioside | Leucocytes |
Asialo GM2-ganglioside Keratan sulfate | beta-galactosidase | Cultured skin fibroblasts | |
Globotriaosylceramide lipidosis | Globotriaosylceramide | Alpha-galactosidase | Leucocytes Cultured skin fibroblasts |
Sphingomyelin Lipidosis Types I/II | Sphingomyelin | Sphingomyelinase (phospholipase C) | Leucocytes Cultured skin fibroblasts |
Glucosylceramide Lipidosis | Glucosylceramide Glucosylsphingosine | Glucosylceramide beta-glucosidase | Leucocytes Cultured skin fibroblasts |
Galactosylceramide Lipidosis | Galactosylceramide Galactosylsphingosine | Galactosylceramide beta-galactosidase | Leucocytes Cultured skin fibroblasts |
Sulfatide Lipidosis | Sulfatide | Sulfatide sulfatase (arylsulfatase a) | Leucocytes Cultured skin fibroblasts |
Mucosulfatidosis | Sulfatide Cholesterol sulfate Mucopolysaccharide sulfate | Multiple sulfatases | Leucocytes Cultured skin fibroblasts |
Ceramide Lipidosis (Farber Disease) | Ceramide | Ceramidase | Leucocytes Cultured skin fibroblasts |
Adrenoleukodystrophy Group Neuronal Ceroid Lipofuscinoses | Very-long-chain fatty acids | Defective peroxisomal fatty acid oxidation | Plasma Cultured skin fibroblasts |
CLN1 | Saposins A and D | Palmitoylprotein thioesterase | Leucocytes Cultured skin fibroblasts Plasma Cerebrospinal fluid |
CLN2 | ATP synthase subunit c | ||
Saposins A and D | Pepstatin-insensitive peptidase | Leucocytes Cultured skin fibroblasts | |
CLN3 | ATP synthase subunit c Saposins A and D | None known | CLN3 mutations |
CLN5 | ATP synthase subunit c Saposins A and D | None known | CLN5 mutations |
CLN6 | Unknown | None known | CLN6 mutations |
CLN8 | ATP synthase subunit c | None known | CLN8 mutations |
Sialidosis | |||
Type I and II | Sialic acid-containing oligosaccharides/glycoproteins | Oligosaccharide alpha-sialidase | Culture skin fibroblasts |
Sialic Acid Storage Disorders | |||
Infantile Type | Sialic acid in urine and tissues | Unknown | Increased sialic acid in urine |
Salla Type | Sialic acid in urine and fibroblasts | Unknown | Increased sialic acid in urine |
Type I GM1 gangliosidosis, the most common form, is a rapidly progressive disorder of early infancy manifesting as profound psychomotor deterioration and hepatosplenomegaly as well as the dysmorphic and radiologic features of Hurler disease (Table 24-1, Box 24-2). The last consist of multiple bone deformities (skull, vertebral bodies, ribs, and extremities) called dysostosis multiplex, hence the former name of Hurler variant or Tay-Sachs disease with Hurler features.
|
Onset occurs within the first weeks of life with evident psychomotor delay marked by apathy, exaggerated startle, generalized seizures, and hypotonia that progresses to spasticity. Cherry-red maculae are found in about 50% of cases. Systemic features include corneal clouding, gingival hyperplasia, macroglossia, kyphoscoliosis, broad hands, and flexion contractures, particularly of fingers, elbows, and knees. Death occurs by age 2.
Neuroimaging reveals prominent atrophy, increased density of thalami and reduced density of basal ganglia by CT, and hyperintensity of thalami and basal ganglia on T1 and hypointensity on T2, along with evidence of delayed myelination by MRI.21 Electroretinography is normal whereas VERs are delayed. EEG findings generally consist of nonspecific slowing.
Type II GM1 gangliosidosis presents at 6 to 12 months with psychomotor regression after normal early development. Neurological features are dominated by spasticity, ataxia, dystonia, and prominent myoclonic and generalized seizures. Corneal clouding, cherry-red maculae, dysmorphic facies, and hepatosplenomegaly are not seen. Bony changes are typically limited to moderate beaking of vertebral bodies and hypoplasia of the hips. EEG and neuroimaging findings are similar to type I. Progression is relentless leading to death by age 3 to 10 years.
Type III begins in early childhood with slowly progressive ataxia and mild cognitive impairment followed by slurred speech and progressive extrapyramidal signs (dystonia and rigidity) in adulthood.22 Dysmorphic features, cherry-red maculae, corneal clouding, and hepatosplenomegaly are absent. Bony abnormalities of the vertebral bodies and hips are mild. MRI may show increased intensity of the putamen on T2. Longevity appears normal.
Pathologic findings are described best for type I. Neurons, including those in Meissner plexus, and glia are involved diffusely, their cytoplasm stuffed with PAS-positive storage material. Bone pathology is similar to that for Hurler disease. Diffuse neuronal involvement is less evident in later onset forms. Neuronal involvement in type III is most prominent in caudate and putamen.
GM1 ganglioside comprises 80% to 90% (normal = 20%) of total gray matter gangliosides (Table 24-2). Asialo-GM1 (GM1 minus sialic acid) also accumulates, exceeding normal values by up to 20 times. In the acute form, GM1 also accumulates in liver and spleen. In the chronic form, GM1 accumulation is generally limited to caudate and putamen. Deficient β-galactosidase activity in leukocytes or cultured skin fibroblasts establishes the diagnosis. Multiple mutations have been identified in GBL1, although five common mutations account for the majority.
A separate transcript of GBL1 encodes a β-galactosidase-like protein without enzyme activity and identical to the elastin-binding protein (EBP). Six infants with GM1 gangliosidosis and prominent cardiac involvement had GBL1 mutations in the region common to both enzyme and EBP proteins.
A unique feature of the acute-onset form is increased keratan sulfate levels in various tissues and excessive urinary excretion accounting for the Hurler-like appearance. Both GM1 ganglioside and keratan sulfate have a terminal β-galactose. Morquio disease, a mucopolysaccharidosis, is characterized by keratan sulfate accumulation and deficient β-galactosidase activity. As such, Morquio B disease is allelic with GM1 gangliosidosis, that is, both diseases are due to mutations in the same gene.
A number of children with mild to moderate reduction of β-galactosidase activity were once considered as variants of GM1 gangliosidosis. This disorder, galactosialidosis, is due to deficiency of a protective protein for sialidase deficiency and β-galactosidase.
|
Globotriaosylceramide lipidosis (angiokeratoma corporis diffusum universale, Fabry disease) is the lone X-linked sphingolipid storage disease (Table 24-1). The principal clinical manifestations involve skin, heart, and kidney. The cutaneous features (angiokeratoma) consist of violaceous angiectatic lesions over the genitalia, thighs, buttock, back, and lower abdomen. These are not unique to Fabry disease, occurring in fucosidosis, β-mannosidosis, galactosialidosis, and Schindler disease. Difficulties with sweating (hypohidrosis) result in temperature intolerance. Lymphedema and episodic diarrhea are also common. Fabry disease has its onset in adolescence or early adulthood,23-25 but despite major clinical issues, survival is often quite prolonged with death from renal or cardiac involvement in the fourth or fifth decades. Later-onset variants are now recognized.26 Cardiac involvement includes left ventricular hypertrophy, valvular dysfunction (especially mitral insufficiency), and conduction abnormalities.27 Obstructive pulmonary disease occurs in about one-third, usually after age 25. Interestingly, subclinical hypothyroidism appears to be more frequent as well.28
The principal neurological problem is painful peripheral neuropathy, generally involving the distal extremities (acroparesthesias). Both severe crises of lancinating pain precipitated by exercise, fatigue, stress, or exposure to sunshine or hot weather, and chronic, unremitting pain, may occur. Corneal and lenticular opacifications and retinal vasculopathies are common. CNS vaso-occlusive events, especially in the posterior circulation and periventricular white matter supply, should place Fabry disease in the differential diagnosis of cerebrovascular accidents in the young male.29 Cranial imaging may suggest prior vaso-occlusive events even without clinical accompaniment.30
Female carriers may develop signs similar to affected males, but usually in middle life. Corneal opacifications occur in approximately 75% of carriers. Other manifestations, while less common, may be as severe as in males, especially the painful peripheral neuropathy.
Natural history studies demonstrate cerebral lesions in some males in their mid-twenties but involvement is found in all males by age 55, whereas chronic renal insufficiency and end-stage renal disease are noted by age 40 to 50. Overall survival in males is age 50 and in carrier females age 70.
A variant form is limited to cardiac involvement. The specific gene defect in this form results in more residual enzyme activity. Onset of cardiac disease is typically at age 40 or older but without the other features of Fabry disease except for mild proteinuria.
Lipid accumulation occurs in vascular smooth muscle and endothelium, kidney, cornea, peripheral nerves, and in neurons of the autonomic nervous system, both central and peripheral. Electron microscopy of peripheral nerves reveals lamellar inclusions in perineurial cells. Peripheral nerve involvement can be easily assessed by analysis of skin biopsy.
Globotriaosylceramide is a quantitatively significant sphingolipid in kidney and is also the first intermediate in the degradation of globoside, the prominent sphingolipid of red cell membranes (Table 24-2). Accumulation occurs in kidney, liver, and lung ranging from 30 to 300 times normal. Alpha-glactosidase (globotriaosylceramide α-galactosidase) activity is deficient. Diagnosis can be made in leukocytes and cultured skin fibroblasts. Numerous mutations have been described.31 A more benign mutation is associated with the cardiac variant.
Renal transplantation may be required for renal failure, but may not result in overall improvement. Enzyme replacement therapy can reduce lipid storage and neuropathic pain and improve renal function and cerebral perfusion.32-38 Replacement therapy has also been safe and effective in symptomatic females.39 Individuals with the cardiac variant may benefit from infusion of galactose, which acts as a molecular chaperone to stabilize mutant enzyme activity. Carbamazepine, phenytoin, or gabapentin may be effective therapy for neuropathic pain or dysesthesias.
|
Sphingomyelin lipidosis is characterized by storage of sphingomyelin (Table 24-1). Acute (type A) and chronic (type B) forms are clinically distinct. Two variants (types C and D), previously thought to have similar etiologies, are not disorders of sphingomyelin catabolism.
Type A is the predominant form, occurring prominently in Ashkenazi Jews and accounting for 85% of the total. Onset occurs in infancy, characterized by severe failure to thrive, hepatosplenomegaly, psychomotor retardation, and frequent respiratory infections. Persistent neonatal jaundice and ascites in later stages may be noted. Neurological abnormalities include hypotonia and weakness initially with progression to spasticity and rigidity. Seizures are rare. Cherry-red maculae are seen in approximately 50% and the ERG response may be diminished. EEG findings consist of nonspecific slowing and voltage attenuation. ABRs may be abnormal and nerve conduction velocities reduced. Death occurs by age 4.
Type B sphingomyelin lipidosis typically spares the central nervous system. Onset occurs in childhood marked by hepatosplenomegaly and respiratory infections. Progression is remarkably indolent, the principal problems arising from the hepatosplenomegaly, cirrhosis, and chronic pulmonary disease. Survival into middle adulthood is usual.
Neuropathologic findings are derived from type A. Brain weight is approximately 75% of normal. Light microscopy reveals large, distended neurons lacking their pyramidal shape. Neuronal storage is prominent, but lipid accumulation also occurs in liver, spleen, lymph nodes, bone marrow, and lung. Peripheral nerves are abnormal with segmental demyelination and cytoplasmic inclusions within axoplasm and Schwann cells.
Sphingomyelin accumulation in brain and other tissues is characteristic of type A (Table 24-2). Sphingomyelin in brain is increased up to 50 times and may represent two-thirds of total phospholipid (normal = 20%). Tissue cholesterol levels are also increased up to 10-fold. Another phospholipid, bis(monoacylglycero)phosphate, is increased nearly 100-fold, and lyso-sphingomyelin or sphingosylphosphorylcholine is also increased in brain, liver, and spleen in type A, but only in liver and spleen in type B. Their role in the pathogenesis of neurological abnormalities is unknown. Brain content of sphingomyelin is normal in type B. Deficient sphingomyelinase activity in leucocytes and cultured skin fibroblasts is profound in both types. Sparing of the central nervous system and prolonged survival in type B patients reflects greater residual enzyme activity and possibly other factors. Multiple mutations have been defined in ASM, but three account for most of type A in the Ashkenazi population. A separate and less severe mutation is found in the majority of type B.
Niemann-Pick C (formerly types C and D) has its onset in early childhood (ages 1-6) with hepato-splenomegaly and hepatic dysfunction, gait difficulties, ataxia, vertical gaze deficit, and cognitive decline. Progression is relentless with death usually in adolescence. Lipid storage consists mainly of cholesterol but also both sphingomyelin and glucosylceramide. Sphingomyelin catabolism is normal in cultured skin fibroblasts. Deficient cholesterol esterification is the hallmark of Nieman-Pick C.
Effective treatment is lacking for type A. Enzyme replacement should be effective in type B, but systematic trials have not been initiated.40
Glucosylceramide lipidosis (Gaucher disease; Box 24-5) has three principal forms (Table 24-1). Type I is prominent in Ashkenazi Jews with an incidence of 1:1600, the general incidence being 1:80,000-160,000. The variant forms are explained by different mutations in the gene for glucosylceramide β-glucosidase.41
|
Type I, the chronic non-neuronopathic form, is the most common, representing approximately 85% of the total.42 The CNS is generally spared, although oculomotor apraxia, seen in acute and subacute forms, has been described. Onset occurs in childhood or adolescence with progressive hepatosplenomegaly.43 It may begin in early adulthood or later. Significant intrafamilial variability has been noted.44 Survival is typically prolonged, although early death from severe pancytopenia or other complications is possible. The principal clinical problems are hypersplenism, bony abnormalities including infarction, osteomyelitis, pathologic fractures, arthritis, chronic pulmonary hypertension, and growth retardation. Secondary neurological problems have been described.45 An association exists between Gaucher disease and Parkinson disease in the Ashkenazi population, but has not been noted in Norway.46-49 With the exception of multiple myeloma, cancer rates are not elevated.50
Type II (neuronopathic) or infantile glucosylceramide lipidosis is a fulminant disorder. Prominent features are failure to thrive, extensor posturing, laryngospasm, profound psychomotor retardation, and hepato-splenomegaly. Weakness and hypotonia quickly progress to rigidity. Oculomotor apraxia or strabismus is typical. Seizures may occur in later stages. Onset occurs in early infancy with survival rare beyond age 2. Fetal hydrops, also seen in other lysosomal disorders (ceramide lipidosis, sialidosis type II, galactosialidosis, β-glucuronidase deficiency, and infantile sialic acid storage disease) may be the presenting sign.51
Type III (Norrbottnian form; subacute neuronopathic) is an intermediate form. Particularly prevalent in Sweden, this form occurs in childhood (4-8 years) with hepatosplenomegaly, horizontal gaze apraxia, motor dysfunction, seizures, and progressive cognitive impairment. In particular, progressive myoclonic epilepsy may be noted.52 Significant intrafamilial variability occurs, some presenting in early childhood, others with a more indolent course. Death often occurs during adolescence, whereas survival into middle age is possible.
Lipid-laden cells are seen in liver, spleen, and bone marrow. The Gaucher cell, a derivative of the reticuloendothelial system, is characterized by wrinkled blue cytoplasm and easily distinguished from cells with foamy cytoplasm in Niemann-Pick disease. Neuronal loss and perivascular accumulation of glucosylceramide are noted in CNS.47 Neuronal loss (type III in particular) is most pronounced in the bulbar nuclei. Typical Gaucher cells are found in pyramidal cell layers of cerebral cortex.
Glucosylceramide levels in liver and spleen may reach 100 times normal (Table 24-2). In types II and III, glucosylceramide content is increased by 80-fold in cerebral cortex and 40-fold in cerebellar cortex. Levels of glucosylsphingosine (lyso-glucosylceramide) are increased up to 1000-fold. Glycosphingosine accumulation, a potential cytotoxin, is prominent already in early fetal life.
Glucosylceramide β-glucosidase activity in leukocytes and cultured skin fibroblasts is markedly deficient. Variable levels of residual enzyme activity within the broad range of GBA mutations are responsible for the clinical variants. However, other biological or epigenetic factors could explain intrafamilial variability in type III (Norrbottnian).
Multiple mutations in GBA have been identified although five mutations account for more than 95% of those in the Ashkenazi population, but only about 50% of those in non-Jewish individuals.53 Different mutations are most common in neuronopathic forms.54 In the African-American population, the most common type I mutations are not seen, and seven other mutations are noted.
Deficiency of an activator protein that is essential for normal glucosylceramide β-glucosidase activity underlies a variant form of Gaucher disease similar to the subacute form. In this variant, glucosylceramide content is elevated in liver, spleen, and brain as in types II and III, while glucosylceramide β-glucosidase activity is normal.
Replacement therapy using recombinant enzyme (glucosylceramide β-glucosidase) results in decreased accumulation of lipid in juvenile and adult forms of Gaucher disease.55-61 In chronic Gaucher disease, complete reversal of systemic involvement is possible. In type III, high-dose enzyme replacement ameliorates systemic involvement and stabilizes neurological involvement in the majority although pulmonary involvement is not improved.
Bone marrow transplantation has led to significant improvement, particularly in type III. Inhibitors of glycosphingolipid synthesis have provided modest benefit and may be adjunctive, especially in individuals who may not tolerate enzyme replacement therapy.62,63
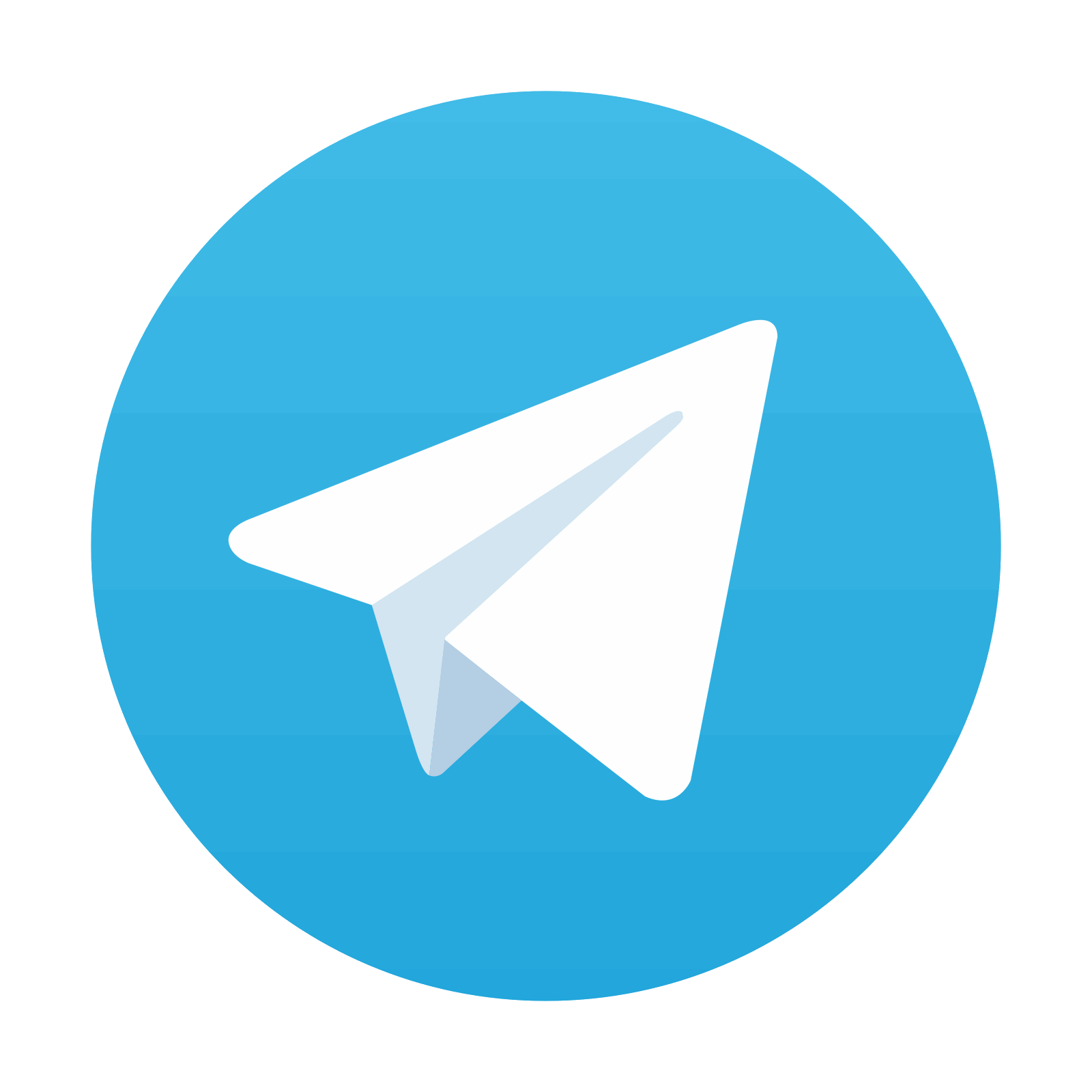
Stay updated, free articles. Join our Telegram channel

Full access? Get Clinical Tree
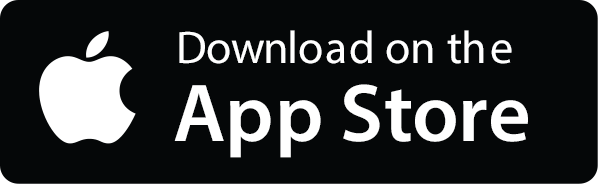
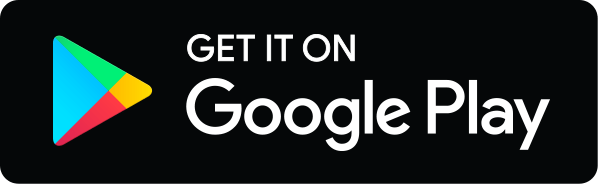