In the acute setting, the fields of Neurology and Internal Medicine are often intertwined, necessitating a working understanding of general principles of Internal Medicine for the practicing neurologist. This chapter explores the neurologic manifestations of selective hematologic disease, direct neurologic involvement of systemic malignancy, and neurologic complications of commonly performed inpatient procedures. Examples of hematologic diseases include red blood cell disorders (eg, sickle cell disease, nutritional anemia, neuroacanthocytosis, and polycythemia vera), bleeding diatheses and platelet disorders (eg, disseminated intravascular coagulation, immune thrombocytopenic purpura, thrombotic thrombocytopenic purpura, hemolytic uremic syndrome, and essential thrombocytosis), and white blood cell disorders (eg, plasma cell disorders such as monoclonal gammopathy of uncertain significance, polyneuropathy, organomegaly, endocrinopathy, M protein, and skin changes [POEMS] syndrome, multiple myeloma, and Waldenströ m macroglobulinemia, chronic myelogenous leukemia, and acute leukemia). Discussion of systemic malignancies includes breast cancer, colon cancer, pancreatic cancer, prostate cancer, and Pancoast tumor. Descriptions of commonly encountered inpatient procedures include cardiac catheterization, cesarean section, upper gastrointestinal endoscopy, and arthroplasty.
CASE 51-1
A 26-year-old man with sickle cell disease is admitted for acute vaso-occlusive pain crisis. Over the course of his life, he has received inconsistent care for his known hemoglobin SS disease. On the third day of hospitalization, the patient develops sudden onset of slurred speech and right-sided weakness.
The primary function of red blood cells is the transportation of oxygen via hemoglobin, a protein molecule comprising 2 α- and two β-globin chains. Disruptions in red blood cell production (diminished or accelerated), alterations of red blood cell membrane structure, and abnormalities in hemoglobin all risk directly inhibiting neurologic cellular function via impaired oxygen delivery. The varied underlying pathophysiologic mechanisms of red blood cell abnormalities, both directly and indirectly, pose the risk of contributing to additional neurologic sequela.
Reassuringly, the initial serological assessment for hematologic disease, the complete blood count, provides very helpful information when a red blood cell disorder is suspected. The number, morphology, and hemoglobin content of red blood cells are all reported in the red cell indices. Evaluation of the peripheral blood smear provides further morphologic detail. With the addition of another common blood test, the reticulocyte count, bone marrow activity can also be assessed.
A clinician’s responsibility in the investigation of suspected red blood cell disease is to use pertinent historical information—including family and social history—along with physical examination findings, to pursue more directed testing.
The presence of sickle hemoglobin (HbS) is the hallmark of sickle cell disease and can be measured by hemoglobin electrophoresis. In patients with the most common and severe form of sickle cell disease (HbSS), HbS can represent over 90% of the circulating hemoglobin. Hemoglobin electrophoresis can also identify the presence of compensatory fetal hemoglobin (HbF) and an additional hemoglobin variant, hemoglobin C, that is present in the less clinically severe genotype, HbSC.1
When sickle hemoglobin—created by an amino acid substitution in the β-globin chain—is deoxygenated, red blood cells are deformed into an inflexible sickle or crescent shape that increases blood viscosity through alterations in red blood cell interactions with leukocytes, platelets, vascular endothelium, and clotting factors. Impaired micro- and macrovascular circulation and its resultant ischemia in any location of the body, including but not limited to the kidneys, liver, lungs, heart, and brain, is the fundamental basis of clinical disease in sickle cell anemia.1
Two genotypes of sickle cell disease, HbSS and HbSβ0-thalassemia, are often phenotypically indistinguishable and commonly referred to as sickle cell anemia. These genotypes are associated with the most severe clinical manifestations of sickle cell disease. Additional genotypes, HbSβ+-thalassemia and HbSC, represent milder disease states. In all, sickle cell disease affects between 70,000 and 100,000 people in the United States, most of whom are of African ancestry, with a minority being of Hispanic, Middle Eastern, or Asian Indian descent.
Sickle cell trait, the heterozygous carrier state, does not carry a significant risk for clinical disease but is present in an estimated 3.5 million people.1
CASE 51-1 (continued)
The patient in case 1 is emergently taken for MRI/MRA. Imaging confirms the presence of an acute left middle cerebral artery (MCA) territory infarction, in addition to evidence of prior strokes.
Epidemiologic data presented by Strouse et al in 20112 estimated the incidence of first stroke in all adults with sickle cell disease patients at 360–1160 per 100,000 person-years, compared with 12 per 100,000 person-years in African Americans less than 35 years of age and 202 per 100,000 for African Americans aged 35–54 years. Both ischemic and hemorrhagic stroke are common in the sickle cell population, with a greater proportion of hemorrhagic strokes occurring in children and young adults compared to their older counterparts.3
In addition to risk factors known in the general population, such as diabetes mellitus, atrial fibrillation, and hyperlipidemia, sickle cell genotype, age, hypertension, and anemia severity have all been identified as clinical risk factors for ischemic stroke in sickle cell patients. In children, a temporal association between ischemic stroke and both acute chest syndrome and Parvovirus-induced aplastic crisis has been identified.
Risk factors for hemorrhagic stroke include low baseline hemoglobin and high baseline leukocyte count. Recent transfusion, corticosteroid therapy, or acute chest syndrome, as well as hypertension and coagulopathy, have all been implicated.2
Current guidelines recommend annual screening of children ages 2 through 16 with transcranial Doppler (TCD).1 The Stroke Prevention Trial in Sickle Cell Anemia (STOP) demonstrated that chronic transfusions in children and adolescents found to have abnormal TCD velocities provided a significant reduction in strokes. For those sickle cell patients unable to tolerate chronic transfusion or whose TCD velocities are borderline, hydroxyurea is a promising therapeutic option.2,4
As in nonsickle cell patients, neuroimaging is necessary in the setting of acute stroke. Vascular imaging is particularly important in sickle cell disease due to the risk for vasculopathy and concomitant moyamoya. Transfusion, particularly exchange transfusion, should be strongly considered given its immediate benefit in reducing the burden of sickle hemoglobin. A typical goal is to reduce HbS concentration to <30%. In addition to transfusion therapy, thrombolytics and aspirin can be used effectively in the appropriate clinical circumstances.2,4
Anemia due to nutritional deficiencies or toxins—B12, lead poisoning
Structural abnormalities—hereditary spherocytosis, neuroacanthocytosis
Disorders of production—aplastic anemia, polycythemia vera (PV)
Disorders of destruction—autoimmune hemolytic anemia, microangiopathic hemolytic anemia associated with autoimmune disease or platelet disorders (see Part 1.2)
The complete blood count and peripheral blood smear are helpful in initial laboratory studies for the identification of nutritional deficiencies associated with hematologic abnormalities (see Figure 51-1). Based on the combination of clinical suspicion (eg, dorsal column dysfunction) and suggestive abnormalities (eg, hypersegmented neutrophils with macrocytic anemia), more specific testing (eg, vitamin B12, methylmalonic acid, and homocysteine levels) can help to arrive at the appropriate diagnosis (eg, B12 deficiency).
Figure 51-1
Hypersegmented neutrophils in vitamin B12 deficiency. Reproduced with permission from Soupir CP and Hasserjian RP: Myeloid Neoplasms and Mastocytosis: Therapy-related myeloid neoplasm session from the 2007 Workshop of Society for Hematopathology and European Association for Haematopathology.

Often, replacement of the deficient product or removal of the offending agent will correct the associated abnormalities. Even in the hospital setting, repletion can be guided by evidence-based literature to ensure safe, effective, and efficient delivery of care. To continue the example illustrated above, a 2005 Cochrane review found that high oral doses of vitamin B12 could achieve hematologic and neurologic responses as effectively as intramuscular administration.5
Unfortunately, not all hematologic disorders are as common and readily diagnosed as nutritional deficiencies.
What other red blood cell findings could suggest an underlying disorder with neurological consequences?
One important, but rare, cause of neurologic dysfunction associated with hematologic abnormality is the group of genetic neurodegenerative diseases referred to as neuroacanthocytosis. In addition to the presence of characteristic spiky red blood cells, known as acanthocytes, on peripheral smear, these diseases include features such as dystonia, chorea, and neurodegeneration of the basal ganglia. Current classification of neuroacanthocytosis consists of 4 subtypes: chorea-acanthocytosis, McLeod syndrome, Huntington disease-like 2, and pantothenate kinase-associated neurodegeneration.6
Patients exhibiting the signs and symptoms of a movement disorder with negative testing for Huntington disease and suggestive neuroimaging findings, such as iron deposition in the basal ganglia, should be considered for this disease, particularly if acanthocytes are identified on peripheral blood smear. Genetic testing can both confirm the diagnosis and distinguish the various subtypes.
As the role of acanthocytes in the neurologic manifestations of neuroacanthocytosis is unclear, numerous medical therapies directed at alleviating symptoms—botulinum toxin A, tetrabenazine, and atypical neuroleptics, for example—have produced disappointing long-term results. More aggressive interventions may hold promise but must be undertaken carefully and with expert consultation. A case report by Lim et al7 described marked improvement in dystonia, chorea, and overall quality of life in a 32-year-old patient who underwent deep brain stimulation (DBS) but only after a failed first attempt due to intraoperative complications.
Are there conditions where the red blood cell disorder causes both direct and indirect neurological manifestations?
PV is a condition classified as a myeloproliferative disorder where myeloid expansion in the peripheral blood leads to excessive numbers of circulating red blood cells (see Table 51-1). Interestingly, while the hyperviscosity of PV carries its own neurological risks, hemorrhage and thrombosis alone do not decisively explain all neurologic symptoms associated with the disease.
Blood Samples of Patients, Acanthocyte Count, and Clinical Information from a Sample of Neuroacanthocytosis Patients
Sample | Sex | Age | Acanthocytes, % | Mutations Found | Prominent Clinical Features |
---|---|---|---|---|---|
ChAc1 | F | 33 | 39.8 | VPS13A, c.4282GC, c.7806GA | Chorea, epilepsy, tongue dystonia, dysarthria |
ChAc2 | F | 40 | 21.1 | VPS13A, 1208delAGAC, 7867C>T | Chorea, epilepsy, tongue dystonia, dysphagia, dysarthria |
ChAc3 | M | 46 | 24.8 | VPS13A, c.8529_8530het_dupA, c.9078-2A>G | Epilepsy, dysarthria, symmetric Parkinson syndrome, cognitive impairment |
ChAc4 | M | 48 | 25.6 | VPS13A, 237delT, 9429delAGAG | Chorea, epilepsy, tongue biting, dysarthria |
ChAc5 | F | 39 | 45.9 | n/a | Chorea, dysarthria |
ChAc6 | M | 26 | 26.7 | VPS13A, c.6059delC | Chorea, epilepsy |
ChAc7 | M | 21 | 25.8 | VPS13A, c.6059delC | Epilepsy |
ChAc8 | F | 43 | 19.4 | n/a | Chorea, epilepsy, dysarthria, cognitive impairment |
ChAc9 | M | 53 | 12.5 | n/a | Chorea |
ChAc10 | F | 47 | 1.5 | VPS13A, exon 54 deletion | n/a |
ChAc11 | M | 45 | 40.0 | VPS13A, exon 54 deletion | n/a |
ChAc12 | M | 38 | 49.0 | VPS13A, exon 54 deletion | n/a |
MLS1 | M | 63 | 33.3 | XK, c.1023G>A | Profound orofacial dyskinesia, dysphagia, dysarthria, severe sensorimotor neuropathy with generalized muscle wasting |
PKAN+1 | M | 7 | 29.2 | PANK2, c.1561GA p.G521R | n/a |
PKAN+2 | M | 12 | 22.1 | PANK2, c.628+2TG | Generalized dystonia, rigidity, pyramidal signs |
PKAN+3 | F | 8 | 42.3 | PANK2, c.664CT | Generalized dystonic, pyramidal signs in lower extremities |
PKAN+4 | M | 15 | 33.1 | PANK2, c.664CT | Generalized dystonic, pyramidal signs in lower extremities |
PKAN+5 | M | 7 | 17.5 | PANK2, c.215insA | n/a |
PKAN+6 | M | 9 | 41.1 | PANK2, c.1325_1328ATAG | Oromandibular, axial dystonia |
MPAN-1 | F | 14 | 3.4 | c19orf12, c.194GA p.G65E | Dystonic movements, pyramidal and cerebellar signs |
PKAN-2 | M | 33 | 3.7 | PANK2, c.1466TC, 1583C>T | Severe dystonia, some pyramidal signs |
PKAN-3 | F | 27 | 1.0 | PANK2, c.1231GA, c.1255AG | n/a |
PKAN-4 | M | 25 | 0.0 | PANK2, c.1231GA, c.1255AG | n/a |
PKAN-5 | F | 25 | 2.4 | PANK2, c.987del, c.1253CT | n/a |
PKAN-6 | F | 17 | 1.4 | PANK2 (details n/a) | n/a |
PV has an estimated incidence of 2–10 cases per million, with a slight predilection for those of European descent. Onset is typically insidious, and presenting symptoms range from aquagenic pruritus to gastrointestinal complaints to neurologic disturbances such as headache. Physical examination may be normal, reveal suggestive findings such as ruddy cyanosis (facial plethora) or splenomegaly, or demonstrate a less specific abnormality such as chorea. Laboratory investigation is likely to identify elevated hemoglobin (above 18.5 g/dL in men and 16.5 g/dL in women) and mutation in the Janus Kinase 2 (JAK2) gene but may also reveal hypercellular bone marrow or decreased serum erythropoietin.8,9
Common neurological manifestations of PV include headache, dizziness, paresthesias, tinnitus, ischemic and hemorrhagic stroke, and chorea.9
The mainstay of therapy in PV is reducing red cell mass through serial phlebotomy. Even the more enigmatic consequences, such as chorea, have been found to improve with normalization of packed red blood cell volume. The addition of cytoreductive therapy, most commonly hydroxyurea, has been shown to further reduce the incidence of adverse consequences, such as thrombosis, and improve disease survival. In fact, median survival is extended from less than 18 months to more than 10 years with appropriate treatment.9,10
Red blood cell disorders can impact the neurologic system through abnormalities in structure, function, or production.
The initial tests to perform in suspected red cell disorders are the complete blood count and evaluation of the peripheral blood smear with or without a reticulocyte count.
While management principles for acute neurologic consequences are similar regardless of etiology, disease-specific treatment may be of uniquely important benefit and often warrants expert consultation.
Identifying and attenuating the risk for hemorrhagic complications is an important facet of inpatient care. The most commonly used tests for assessing bleeding risk include evaluation of the platelet count on a complete blood count and coagulation studies, namely prothrombin time (PT) and activated partial thromboplastin time (aPTT). Due to its operator dependence and resultant poor reproducibility, the bleeding time is no longer considered a valuable investigation for bleeding risk. While small studies have been performed, highly specialized biomarkers, such as factor XIII level, fibrinopeptide, and even C-reactive protein, have not been statistically proven to predict devastating bleeding events, such as intracerebral hemorrhage.11
Despite their frequent clinical use, even the predictive ability of the more “reliable” PT and aPTT studies to identify high risk for bleeding has been called into question. Indeed, one of the most dreaded hematologic complications of systemic illness, disseminated intravascular coagulation (DIC), has been reported to have abnormal PT and aPTT in less than 50% of cases.12
If typical coagulation studies may be normal, how is disseminated intravascular coagulation diagnosed?
DIC is an acquired process of overstimulation of the coagulation cascade that results in thrombosis, platelet and coagulation factor consumption, and, ultimately, hemorrhage. A presumptive clinical diagnosis can be made when bleeding from more than 3 unrelated sites is identified in the setting of a disorder known to be associated with disseminated intravascular coagulation. However, diagnosis can be aided by use of an objective scoring system, such as that offered by the International Society of Thrombosis and Haemostasis, wherein a low platelet count, elevated fibrin markers, such as D-dimer or fibrin split products, prolonged PT, and low fibrinogen level all increase the likelihood of disease.12,13 See Table 51-2.
International Society of Thrombosis and Haemostasis (ISTH) Diagnostic Criteria to Diagnose Disseminated Intravascular Coagulation (DIC)
Does the patient have an underlying disorder known to be associated with overt DIC? | ||||
---|---|---|---|---|
1. If yes, proceed; if no, do not use this algorithm | ||||
2. Order global coagulation tests (prothrombin time, platelet count, fibrinogen, fibrin-related marker—D-dimer, fibrin degradation products) | ||||
3. Score the test results
|
Platelet count Elevated fibrin marker Prolonged PT Fibrinogen level |
>100 × 109/l = 0 No increase = 0 <3 s = 0 >1 g/L = 0 |
<100 × 109/l = 1 Moderate increase = 2 >3 s = 1 <1 g/L = 1 |
<50 × 109/l = 2 Strong increase = 3 >6 s = 2 |
4. Calculate score
|
≥5 <5 |
Compatible with overt DIC: repeat score daily Suggestive of nonovert DIC: repeat next 1–2 days |
Why is heparin a suggested treatment modality in a hemorrhagic condition such as disseminated intravascular coagulation?
Heparin prevents the conversion of fibrinogen to fibrin by augmenting the action of the anticoagulant factor antithrombin and is not universally recommended in all cases of DIC. Specifically, heparin use should be considered when thromboembolic disease, retained products of conception, or a condition known as purpura fulminans are present.13
In addition to the low platelet state in disseminated intravascular coagulation, thrombocytopenic conditions such as immune thrombocytopenic purpura (ITP), thrombotic thrombocytopenic purpura (TTP), and hemolytic uremic syndrome (HUS) should be considered in the appropriate clinical circumstances.
While commonly discussed in the pediatric population, ITP actually has a significant prevalence in adults, as well, at an estimated 100 cases per million people.13 As primary ITP is a diagnosis of exclusion, causes of secondary ITP, such as infection, medications, and cancer, should be thoroughly investigated. Complete blood count with reticulocyte count, peripheral blood smear, Coombs test, and blood group testing are all appropriate components of the initial evaluation of suspected ITP.
Fortunately, despite the often profound thrombocytopenia of ITP, severe bleeding is unusual and intracranial hemorrhage is considered rare. As a consequence, adult mortality is estimated at 5%. While a direct correlation between platelet counts and spontaneous, life-threatening bleeding is not definitively established, the greatest risk for severe hemorrhage occurs at platelet counts less than 10,000/mm3. Treatment for asymptomatic patients with platelet counts above 30,000/mm3 is not recommended according to management guidelines proposed by the American Society of Hematology.13 First-line therapy for adults with bleeding or platelet counts below 30,000/mm3 is corticosteroid administration, namely prednisone at a dose of 1 mg/kg per day. Intravenous immunoglobulin (IVIg) and anti-Rh(D) infusions have also been effective. Severe or life-threatening bleeding, including intracerebral hemorrhage, requires resuscitation and consideration of platelet transfusion, despite the known immune destruction of donor platelets in ITP.
Unlike ITP, neurologic consequences are more common in TTP, a condition whose diagnostic criteria classically comprise the pentad of fever, hemolytic anemia, thrombocytopenia, renal impairment, and neurologic manifestations. The variety of neurologic conditions, including headache, seizure, altered mental status, focal neurologic deficit, or aphasia, among others, speaks to the variable extent of severe systemic thrombosis that distinguishes the condition from ITP. Untreated TTP has a mortality rate up to 90% with prompt recognition and treatment, reducing mortality to 10–20%. Reassuringly, at an estimated 3.7 cases per million people, the condition is decidedly rarer than ITP.13
A 2009 Cochrane review14 found that plasma exchange therapy with fresh-frozen plasma remains the most effective treatment for TTP. Plasma infusion therapy (transfusion of fresh-frozen plasma) is considered a temporizing measure only. Platelet transfusion is contraindicated due to its demonstrated worsening of the thrombosis.
Despite the growing body of evidence distinguishing the two conditions clinically, pathophysiologically, and genetically, TTP and HUS are still considered to exist on a disease spectrum. Given the presence of microangiopathic hemolytic anemia in both diseases, the presence of schistocytes on peripheral blood smear does not provide a diagnostic distinction. While renal insufficiency can be seen in both conditions, renal involvement is typically more severe in HUS. Its Shiga toxin-producing bacterial association (eg, E. coli O157:H7) with resultant bloody diarrhea is one helpful characteristic of HUS that is not present in TTP. ADAMSTS-13 activity, the inherited or acquired deficiency of which defines the pathophysiology of TTP, is not a feature of HUS.
As reconstitution of ADAMSTS-13 activity is the fundamental objective in TTP management, the disparate pathophysiology of HUS is not amenable to the same form of treatment. In the same Cochrane review14 supporting the use of plasma exchange with fresh-frozen plasma in TTP, supportive therapy, including hemodialysis, was found to be the most effective treatment of HUS. However, due to the challenging clinical overlap of the diseases and the potentially devastating consequences of untreated TTP, initial management with plasma exchange should be considered in suspected HUS patients with severe neurologic abnormalities until a definitive diagnosis is made.13
While the often marked platelet elevation found in the inflammatory condition known as reactive thrombocytosis carries only minimal risk for circulatory consequences, the myeloproliferative disorder of platelets, essential thrombocytosis, warrants special treatment and clinical consideration.
The estimated incidence of essential thrombocytosis is 1–2.5 cases per 100,000 people per year with a suggested female predominance and peak incidence between 50 and 70 years of age. Patients are commonly asymptomatic but may present with the unique condition of erythromelalgia, which is characterized by hyperemia, edema, and neuropathic pain of the distal extremities. While the logical complication of excess circulating platelets, thrombosis, does occur in approximately 20% of essential thrombocytosis patients, hemorrhage is also surprisingly common at 10%.
Micro- and macrovascular thrombotic complications are effectively reduced with the mainstay of treatment, low-dose aspirin. The presence of risk factors for higher rates of thrombosis (eg, advanced age, prior thrombosis, and identified JAK2 mutation) may necessitate hydroxyurea therapy.8
Bleeding is a dreaded complication of inpatient hospitalization, and evaluation for its risk should be considered, particularly in severely ill patients.
PT and aPTT alone do not provide a complete assessment of bleeding risk.
High clinical suspicion and a willingness to initiate early plasma exchange (PLEX) therapy are necessary in the setting of thrombocytopenia to reduce the severe mortality of thrombotic thrombocytopenic purpura.
While hyperviscosity syndrome and its resultant circulatory compromise is a common concern in many states of hyperproliferative disease, various white blood cell disorders also carry the risk of central nervous system involvement by external compression, obstruction, mass effect, and even direct invasion. The heterogeneity of plasma cell disorders is an excellent example of such diverse pathology.
The most common neurologic involvement in plasma cell disorders is peripheral neuropathy (see Table 51-3). In fact, the demyelinating neuropathy of monoclonal gammopathy of undetermined significance (MGUS) is considered to be the only clinically significant neurologic complication of the disorder and is often the only clinically apparent symptom. Given the underlying monoclonal cell population and its resultant gammopathy, it is not surprising that the peripheral neuropathy associated with plasma cell disorders is immunoglobulin-mediated.15
Plasma Cell Disorders and Peripheral Neuropathy
Peripheral Neuropathy | Associated Disease | Ig | Incidence of PN | Symptoms |
---|---|---|---|---|
Sensory > motor demyelinating | MGUS—anti-MAG positive WM—anti-MAG positive
MGUS—anti-MAG negative WM—anti-MAG negative | IgM IgG, IgA IgM | 8–37% in MGUS 5–10% in WM | Slowly progressive gait ataxia, tremor, loss of joint position, Romberg sign
Chronic, symmetric, progressive, distal sensory or sensorimotor (CIDP-like) CIDP-like |
Sensory > motor axonal
| Cryoglobulinemia
Treatment-related | IgG (or IgM) n/a | ∼70% 40–75% | Painful, progressive, symmetric distal sensorimotor, ± multiple mononeuritis Primarily sensory neuropathy |
Motor > sensory demyelinating | MM POEMS | IgG (or IgM) IgG, IgAp | 5–50% >90% | CIDP-like Proximal and distal sensorimotor, loss of vibration and proprioception |
Sensory > motor ± autonomic dysfunction | Amyloidosis | IgG, IgA | 15–20% | Painful, progressive, symmetric distal sensorimotor; autonomic dysfunction with orthostatic hypotension, bladder or bowel dysfunction |
Like MGUS, POEMS syndrome is characterized by its peripheral neuropathy, which is typically symmetric, distal, ascending, and demyelinating, with predominate motor nerve involvement. To be more specific, the acronym “POEMS” stands for Polyneuropathy, Organomegaly, Endocrinopathy, M protein, and Skin changes. POEMS syndrome is classified as a paraneoplastic process due to an underlying plasma cell disorder.
Unlike MGUS, POEMS has been associated with central nervous system (CNS) involvement, including a reported 5-year stroke risk of 13.4%.16 While the pathophysiologic mechanism of stroke in POEMS syndrome is not well understood, management must incorporate treatment of the underlying condition, which could include local (eg, irradiation) or systemic (eg, corticosteroids, alkylating agents, stem cell transplantation) therapies.17
CNS involvement in multiple myeloma is both more varied and more common than in POEMS syndrome. Spinal cord compression (affecting 20% of patients) may result from vertebral body collapse due to myeloma bone involvement or extension of plasmacytomas into the epidural space. Leptomeningeal infiltration is rare (1% of cases) but portends a grave prognosis (median survival of 1.5–3 months). Intracranial plasmacytomas have been described in the pituitary gland, orbit, and base of the skull with resultant neurologic compromise directly related to anatomic location. Similarly, encephalopathy and cranial nerve palsies in multiple myeloma may be due to known metabolic derangements, such as hypercalcemia or uremia. Hyperviscosity is reported to occur in 2–6% of cases.15,18
Affecting 10–30% of cases, hyperviscosity syndrome (eg, neurologic symptoms, vision changes, and mucosal bleeding) due to IgM hypergammaglobulinemia found in Waldenströ m macroglobulinemia has a much higher frequency than the typical hyper-IgG or hyper-IgA states of multiple myeloma. Treatment is aimed at rapidly reducing the IgM level through interventions such as intravenous hydration and plasmapheresis.15
Among the myeloproliferative disorders, chronic myelogenous leukemia (CML) can be associated with exceptionally high levels of circulating white blood cells, particularly in the chronic phase (before the accelerated and blast phases occur). Fortunately, despite white blood cell counts greater than 100,000/µ L in more than 50% of patients at presentation, symptomatic hyperviscosity is rare in CML.8
Although rare, a hyperviscosity triad of neurologic symptoms, visual changes, and mucosal bleeding similar to that described in Waldenström’s macroglobulinemia can occur in CML. When present, white blood cell hyperviscosity—referred to as hyperleukocytosis or leukostasis—has a mortality of 20–40%, a least partially attributable to pulmonary complications of the syndrome. Fever, almost universally present in patients presenting with hyperleukocytosis, can be an important clue to the presence of the syndrome. Prompt intravenous hydration and cytoreduction interventions, such as leukapheresis, are warranted to reduce the risk of complications.8
Hemorrhagic complications occur in 20% of acute leukemia cases. Leukemic cell infiltration from hyperleukocytosis is an important cause of intracranial hemorrhage in acute leukemia but typically occurs only in the setting of marked leukocytosis where white blood cell counts exceed 300,000 leukemic cells/µL. Other common causes of intracranial hemorrhage in acute leukemia include DIC, disseminated aspergillosis or mucormycosis, and thrombocytopenia.19
Leukemic parenchymal tumors, known as chloromas, have been reported, although these solid tumors consisting of leukemic blasts more often result in epidural spinal cord compression due to their predilection for bone or dural attachment. Encephalopathy and meningitis are additional complications that can arise from direct leukemic infiltration, as a result of malignant immunosuppression, or even as an adverse effect of treatment of acute leukemia.19
Fortunately, not all leukemic cells behave aggressively. The small white blood cells in chronic lymphocytic leukemia, although predicting a median survival of 6 years, rarely progress to a more malignant phenotype.
The hyperviscosity syndrome triad of neurologic symptoms, visual changes, and mucosal bleeding can occur in white blood cell disorders.
Plasma cell disorders, particularly multiple myeloma, have an extensive array of neurologic manifestations, both in the peripheral nervous system and in the central nervous system (CNS).
As in other disorders of excessive production, acute management is typically focused on cytoreductive therapies to improve circulation.
CASE 51-2
A 66-year-old woman presents to the emergency department (ED) with one month of mid-back pain and progressive weakness of her right leg. Examination reveals point tenderness over the thoracic spine and diffusely diminished strength and increased muscle stretch reflexes of the right lower extremity. CT of the thoracic spine confirms the presence of a vertebral mass compressing the spinal cord (see Figure 51-2). Upon further examination, a 5-cm, firm, fixed mass is palpated in the upper outer quadrant of the right breast. There is associated axillary adenopathy.
According to the World Health Organization (WHO), breast cancer is the most common malignancy in women, as well as the leading cause of cancer death in women, encompassing an estimated 16% of all malignancies in females. Identified risk factors for the development of breast cancer include increased age, dense breast tissue, family history of breast or ovarian cancer, and gene mutations, such as BRCA1 and BRCA2.20,21
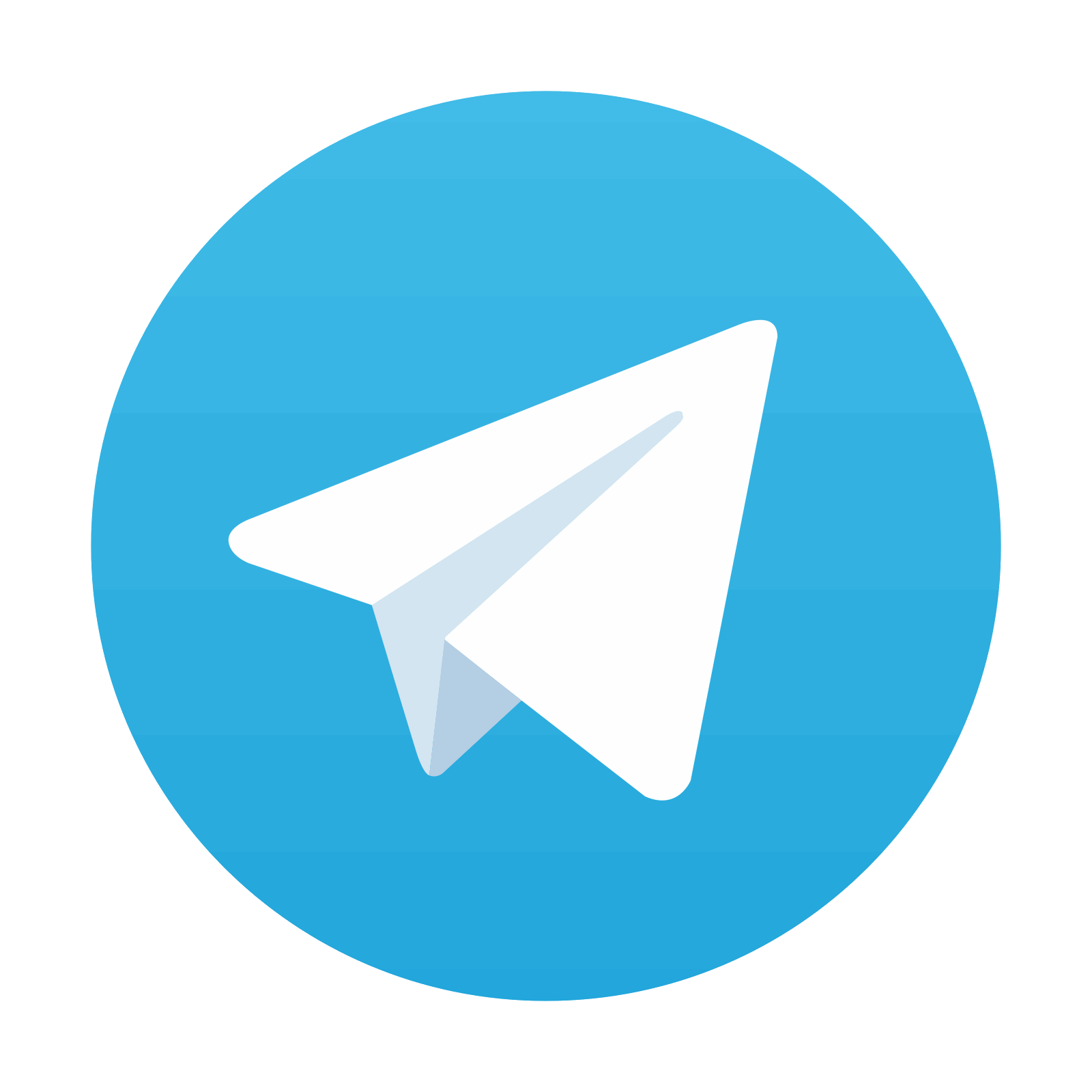
Stay updated, free articles. Join our Telegram channel

Full access? Get Clinical Tree
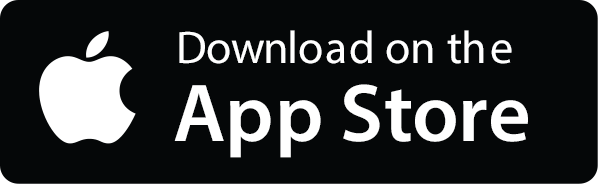
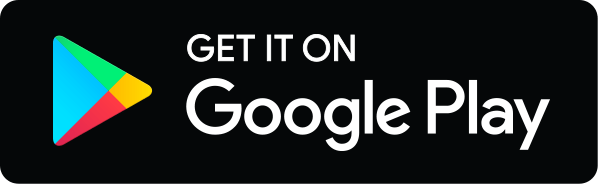