The goal of both intracranial and scalp electroencephalography (EEG) within the epilepsy surgery evaluation is to identify the brain region critical for seizure generation (epileptogenic zone) so that the patient may be rendered seizure-free by the removal of this region. For each, a focal ictal onset is sought, but spatial sampling issues of intracranial electrodes introduce a complexity to clinical decision making that scalp EEG does not have. This includes deciding the type of intracranial electrode and the placement locations. Depth EEG is the surgical placement of intraparenchymal electrode contacts that are along a thin, flexible, plastic tube. Grid and strip electrode contacts exist within a plastic sheet that is laid across the cerebral convexity and are discussed in Chapters 32 and 33.
Both depth and grid/strip EEG techniques are indicated for patients who are considering epilepsy surgery due to medication refractory seizures, whose noninvasive evaluation has not led to an adequate localization of the epileptogenic zone, yet provides sufficient evidence to produce a plausible hypothesis for the epileptogenic zone’s location. The decision whether to use depth or subdural electrodes depends on which method best tests the hypothesis and provides the most useful information regarding the location and extent of the epileptogenic zone. In general, depth electrodes are more helpful when the potentially epileptogenic region is not across the lateral cerebral surface, extends across much of a hemisphere, or includes regions of both hemispheres. Regions that are not on the lateral cerebral surface include the medial surfaces and three-dimensionally complex abnormalities, such as schizencephalies. Subdural electrodes are usually better suited for evaluations of the cerebral surface across one or two lobes and when the evaluation must include an extraoperative mapping of cerebral function, as is possible by stimulating through the subdural electrodes. However, the simultaneous use of both techniques has become increasingly common.
When interpreting all intracranial EEG studies, the spatial sampling limitations always must be considered. Intracranial electrodes record from only immediately adjacent tissue, and ictal onsets outside the coverage region are manifested only when the epileptic rhythm propagates to include tissue adjacent to electrodes. Alternatively stated, the ictal onset depicted with intracranial EEG always shows an ictal onset zone, but the accuracy of this localization is entirely dependent on the accuracy of the electrode placement decision. Therefore, it is critical that intracranial electrodes are used only when the epilepsy surgery hypothesis is clear and the electrode anatomical coverage has the potential to confirm or reject an epileptogenic region’s location and extent.
In general, the appearance of brain activity recorded by scalp EEG differs from that recorded by depth EEG in several ways.1,2 First, the scalp and skull preferentially filter high frequencies, and intracranial EEG depicts these frequencies clearly, which makes the background and occasional transients appear more sharply contoured. Second, scalp EEG records electrical activity that originates at a distance from recording electrodes spaced farther apart from one another, resulting in spatial averaging of electrical activity from a larger volume of tissue. Therefore, the familiar rhythms and discharges on scalp EEG are not present. Third, depth EEG allows recording of distant activity that is simply not accessible with scalp EEG because of the intervening tissue and a decrease in electric field amplitude that is proportional to the square of the distance.
The most extensive comparison between scalp and depth electrode recordings has been in patients with mesial temporal lobe epilepsy (MTLE). Ictal localization of temporal lobe seizures by scalp/sphenoidal EEG is very good but not as sensitive as depth electrode recordings. Surface electrodes tend to detect clinical seizures reliably but are less likely to demonstrate ictal rhythms of an aura or subclinical seizure noted on depth recordings.3 Unilateral localized temporal/sphenoidal ictal patterns on scalp/sphenoidal EEG correctly predicts localization of depth electrode ictal activity in 82 to 94% of cases.4 Because the localizing and lateralizing information provided by scalp/sphenoidal EEG is not perfectly reliable, it cannot be used in isolation for definite ictal localization. Therefore, information from noninvasive studies, such as scalp/sphenoidal interictal EEG, magnetic resonance imaging (MRI), positron emission tomography (PET), lateralization of memory deficit on a Wada test, and single-photon emission computed tomography (SPECT), must also be considered.2 If there is a lack of concordance of data from these noninvasive tests (i.e., tests demonstrate evidence of conflicting lateralization or localization), then intracranial EEG monitoring is necessary. For evaluation of MTLE, depth electrodes are an excellent method.
Depth electrodes are superior to scalp/sphenoidal EEG in localizing and lateralizing ictal onsets in temporal lobe epilepsy. In 1981, Spencer reviewed the available literature comparing scalp and depth EEG and specifically asked how often depth electrodes changed patient management.5 For more than 50% of patients, depth EEG had an influence on surgical plans because (1) scalp localization differed from depth localization; (2) scalp was not localized or multifocal, whereas depth was well localized; or (3) scalp was localized, whereas depth was not localized or multifocal. Eight years later, So et al. described a series of 44 patients with bitemporal independent epileptiform abnormalities on scalp EEG for whom the epileptogenic zone could not be localized or lateralized by scalp EEG and other noninvasive tests and so underwent evaluation with depth electrodes.6 (So et al., 1989). A majority (77%) had seizures originating exclusively or with a strong predominance in one temporal lobe only. There have been many other similar examples of the ability of depth EEG to localize seizure onset when scalp EEG was unable to do so.7
Several case series have identified a specific patient type with unilateral temporal lesions on imaging whose scalp EEG ictal studies have falsely lateralized ictal onset to the contralateral temporal lobe, as identified by subsequent depth electrode studies. Mintzer and colleagues reported five patients with unilateral hippocampal atrophy and surface ictal recordings in which the majority of seizures appeared to initiate in the opposite temporal lobe.8 Depth electrode recordings in four of the five patients demonstrated clear ictal onset in the mesial temporal lobe ipsilateral to the hippocampal atrophy (contralateral to apparent scalp onset). One patient had an unusual bitemporal onset pattern that nevertheless suggested onset in the atrophic hippocampus. All patients underwent resection of the structurally abnormal temporal lobe, and after at least 2 years of follow-up, 80% were seizure-free. Others have likewise reported depth electrode–confirmed false lateralization of temporal lobe seizure onset by surface EEG in patients with temporal lobe lesions on neuroimaging who become seizure-free after removal of the structurally abnormal tissue.9
In patients whose scalp EEG shows well-localized MTLE, depth electrode studies have identified other localizations for epileptogenic foci or multifocal ictal onsets. Sites of seizure onset that are commonly found on depth electrode studies of these patients are the ipsilateral insular lobe, posterior temporal region (location beyond the typical boundaries of an anterior temporal resection), and frontal, unlocalized, or multifocal.7,10–12
The most extensive comparison between depth and subdural electrode recordings has been in patients with MTLE. In this population, depth electrodes have advantages over subdural electrodes because they may be placed within specific anatomical targets, such as the hippocampus. When used in this situation, subdural strip coverage is through a blind insertion under the temporal lobe through a burr hole. However, studies of patients who had both subdural and depth electrode EEG either simultaneously or sequentially showed that in most patients subdural and depth electrode EEG provided similar information about ictal onsets.13–16 However, subdural recordings can give misleading information in this patient population because ictal activity in mesial temporal structures appears earlier in the depth electrodes. Therefore, subdural electrodes can miss a sustained focal ictal rhythm from a brief aura or subclinical seizure.13,14 Subdural electrodes also can suggest unilateral temporal onset, whereas depth EEG shows a nonlocalized pattern, and seizures that are clearly lateralized on depth electrodes can appear nonlateralized on subdural recordings.13 In patients studied with both depth and subdural electrodes, subdural recordings occasionally falsely lateralize a subclinical or clinical seizure.13,16,17 Compared with subdural electrodes, depth electrodes also identify interictal epileptiform activity in MTLE more accurately.18
The varied propagation patterns of mesial temporal lobe seizures accounts for the occasional false lateralization by subdural grid recordings. As discussed in greater detail below, most hippocampal onset seizures propagate first to the ipsilateral neocortex. Therefore, subdural electrodes over the ipsilateral neocortex are usually able to lateralize correctly. However, 23% of hippocampal onset seizures spread initially to the contralateral hippocampus,14 likely via the hippocampal commissure or frontal limbic pathways.15,19 It is hypothesized that the occasional false lateralization seen with subdural electrodes is due to this spread with involvement of contralateral neocortex before spread to the ipsilateral neocortex.17
In patients with suspected neocortical epilepsy, the use of subdural strips and grids is generally preferred because of the larger, contiguous area of cortex that can be studied and the capacity for extraoperative functional mapping through stimulation. However, neocortical epileptic lesions are highly individualized, and depth EEG occasionally is preferred over subdural electrodes. This is demonstrated in a case report of a patient whose epileptogenic focal cortical dysplasia was detected on depth but not subdural electrodes.20In this patient, the resected tissue revealed a focal cortical dysplasia 2.0 to 2.5 cm beneath the surface of the sensory cortex.
Overall, both subdural electrodes and depth electrodes have individual advantages and disadvantages. This partly explains the increasing simultaneous use of both methods in the epilepsy surgery evaluation. Given the unique nature of every patient with epilepsy, the approach and method used to evaluate a patient are individualized based on data from noninvasive studies.
A clear advantage of intracranial electrodes is lack of muscle artifact.2 Because frontalis and temporalis muscle contraction, chewing automatisms, and various head and body movements often greatly increase at seizure onset, it is not uncommon for muscle and movement artifact to significantly obscure scalp EEG ictal recordings. Therefore, intracranial recordings can be much easier to interpret. However, depth EEG is not without occasional technical issues. When large movements affect the leads and amplifiers, some movement artifact may result. In addition, a 20 to 40 Hz respiration-linked vibration artifact has been recorded from limbic depth electrodes.21 Such artifact is due to palatal vibration during partial airway obstruction and can also be simultaneously recorded from nasopharyngeal and sphenoidal electrodes.21,22 Depth EEG electrodes also are much more challenging to replace or repair when they malfunction compared with scalp electrodes.2
Interictal background activity is similar to scalp EEG.1,2 However, the normal transients present within background activity have sharper contours and may appear to be epileptiform sharps or spikes due to the greater abundance of higher-frequency activity1,2 (Figure 31-1). Within the first 48 hours of implantation, transient slowing secondary to the trauma of implantation can be seen.23 This is most commonly present at contacts sampling from the neocortex. Persistent asymmetric or focal slowing on depth electrodes can be a clue as to the spatial extent of abnormal tissue1,2,23 (Figure 31-2). Asymmetric or focal background attenuation and/or slowing can also indicate an issue related to electrode implantation, such as a progressive bleed, infection, or fluid collection.23
Figure 31-1.
Interictal bursts of epileptiform fast activity involve most contacts of two right parietal electrodes. Coverage includes bilateral temporal and parietal lobes. The patient had complex partial seizures and normal MRI. On scalp EEG, interictal epileptiform discharges were right temporal, parietal, and occipital. Ictal onset on both scalp and intracranial EEG was right parietal. A right parietal resection produced seizure freedom (1 sec vertical line interval).

Figure 31-2.
Pathologic rhythmic slowing is present broadly across left temporal electrodes. Coverage includes bilateral temporal lobes. The patient had complex partial seizures and normal magnetic resonance imaging (MRI). Right posterior hypometabolism was present on positron emission tomography (PET). On scalp electroencephalography (EEG), interictal epileptiform discharges were present independently over bilateral temporal lobes, and ictal onset was bitemporal. A right posterior temporal resection produced seizure freedom and identified a cortical dysplasia (1 sec vertical line interval).

The location of interictal epileptiform abnormalities on depth electrode studies is always assessed and considered in the overall clinical context but is generally not considered reliable for indicating the extent of the epileptogenic region.2,23 This is generally true for all intracranial studies, as well as for intraoperative electrocorticography. The most frequent interictal epileptiform activity is typically within the ictal onset zone, but this is not always the case. Interictal discharges within the ictal onset zone may have more complex forms or longer durations when they occur in brief runs. Overall, interictal epileptiform activity is useful but can lead to an incorrect localization of the epileptogenic region (Figures 31-3 and 31-4).
Figure 31-3.
Epileptiform spikes present as runs across the left temporal lobe and are within broadly distributed pathologic slowing. Coverage includes bilateral temporal lobes and orbitofrontal regions with temporal and central scalp electrodes. The greater sensitivity of depth electrodes for epileptiform activity is evident by the absence of corresponding abnormality in the scalp electrode channels. Although the pathologic slowing is unilateral, neuropsychological testing demonstrated bilateral mesial temporal dysfunction (1 sec vertical line interval).

Figure 31-4.
Spike and slow wave discharges occur bilaterally, simultaneously in the premotor areas. Coverage includes bilateral frontal and temporal lobes. Scalp electroencephalography (EEG) demonstrated bilateral spike and slow wave discharges ictally and interictally. Pathologic left sided slowing also was present interictally. MRI and PET were normal. Depth EEG identified a left lateral frontal ictal onset, and a cortical dysplasia was diagnosed after resection of the ictal onset zone (1 sec vertical line interval).

More recently, sampling depth electrode EEG at higher frequencies (2000 Hz) has revealed the presence of interictal high-frequency oscillations. High-frequency oscillations of 100 to 200 Hz (also known as “ripples”) have been demonstrated in the human hippocampus and entorhinal cortex.24 The occurrence of ripples does not clearly differentiate between seizure onset zones and remote areas.25 However, the presence of higher-frequency oscillations of 250 to 500 Hz (termed “fast ripples”) has been strongly associated with the epileptogenic hippocampal and entorhinal cortex, as well as with well-defined neocortical epileptogenic tissue.26,27 Limited data suggest the possibility that the absence of fast ripples is associated with poor localization of epileptogenic tissue.27 This is an area of research that requires further investigation before broad clinical application. Such high-frequency oscillations are currently not recordable using most standard clinical data acquisition systems, which have sampling rates of 200 to 400 Hz.
Ictal Behavior, Interictal EEG, and Ictal EEG in Epilepsy Syndromes Commonly Studied with Depth Electrodes
A clinical seizure or scalp EEG suggestive of a mesial temporal lobe onset also may occur with seizures originating from other structures and then propagating into the mesial temporal region. Such seizures may originate in the temporal neocortex, orbital frontal cortex, medial frontal regions (e.g., anterior cingulate), and occipital cortex because of the preferential propagation from these regions into the mesial temporal structures.28,29 Although they may have signs or symptoms unique to the brain region of seizure origin before evolving into a seizure more typical of MTLE, they also may first manifest with mesial temporal features. Most often, imaging provides the insight that the origin is not mesial temporal. Regardless, intracranial EEG is the most commonly preferred method for determining whether the onset truly is mesial temporal. It is important to keep in mind that not all signs and symptoms typical of mesial temporal origin are exclusive to seizures in this region. For example, oroalimentary automatisms are a common feature of MTLE but have also been associated with seizures of extratemporal origin.30,31
Seizures of mesial temporal origin are typically complex with partial preceding auras in the large majority (~90%). Examples of typical auras include epigastric rising sensation, psychic sensations (commonly fear), déjà vu sensations, jamais vu sensations, somatosensory experiences (e.g., tingling/paresthesias that are bilaterally diffuse, perioral, or at the bridge of the nose), and olfactory or gustatory hallucinations.29,30,32 Sensory distortions may also occur.29 Chapter 8 includes more detail. The aura is usually stereotyped within an individual. Often, patients with MTLE have auras in isolation, meaning that some auras are not followed by a complex partial seizure. The complex partial seizure is typically characterized by impaired consciousness, a fixed stare, and dilated pupils. Automatisms are common during complex partial seizures. Oral-alimentary automatisms such as lip smacking, chewing, and swallowing often occur early in the seizure. Reactive automatisms commonly occur, characterized by a patient continuing with ongoing motor activities in a semipurposeful manner (e.g., continuing to eat groom). Stereotyped automatisms are also common and consist of repetitive movements (e.g., repetitive wiping of the mouth with the right hand) that are the same for each seizure within a patient.29 Automatisms may end with the EEG seizure or continue during some of the postictal period.
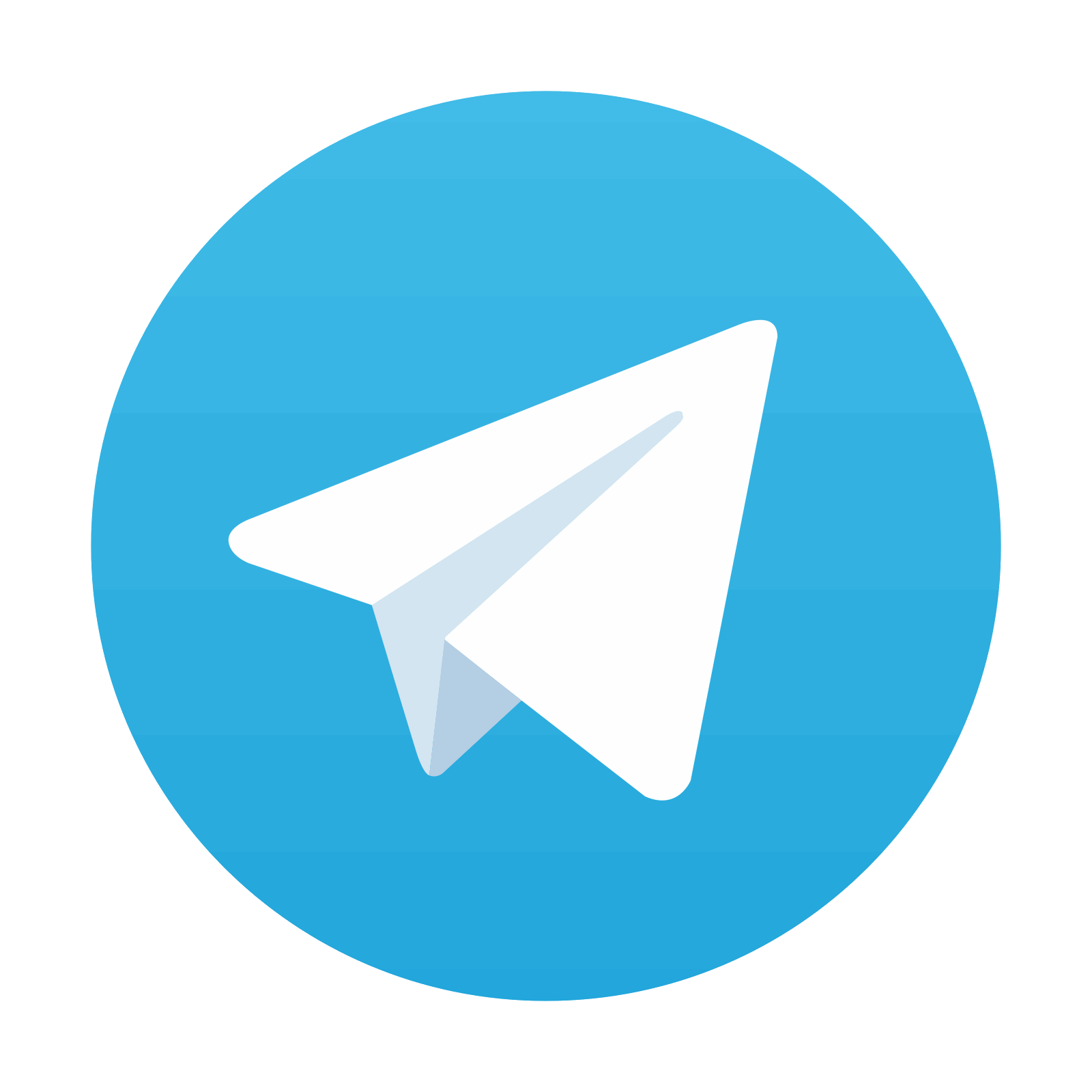
Stay updated, free articles. Join our Telegram channel

Full access? Get Clinical Tree
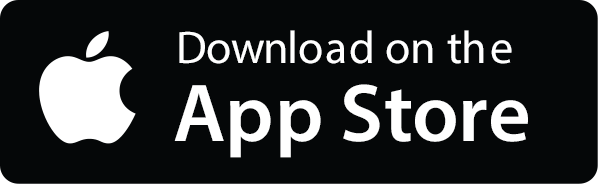
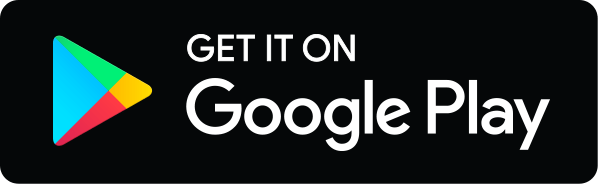