8 Intervertebral Disc Whole Organ Cultures
Marianna Peroglio, Zhen Li, Lorin Michael Benneker, Mauro Alini, and Sibylle Grad
Abstract
Whole intervertebral disc (IVD) organ cultures bridge the gap between in vitro and in vivo assays by offering a controlled environment where cells are kept in their native tissue. Applications of whole organ cultures include testing of tissue responses to novel surgical techniques and repair strategies for both the nucleus pulposus (NP) and the annulus fibrosus (AF). Importantly, whole organ cultures can be instrumental in deciphering the role of stem cells and therapeutic agents in a relevant and controlled environment. In this chapter, the historical perspective of whole organ cultures is presented and both unloaded whole organ cultures and loaded cultures implying the use of bioreactors are highlighted. This section is followed by evidence-based results obtained from whole organ cultures, which range from the evaluation of new surgical techniques and the damage and repair of both the AF and the NP, to the role of stem cells in IVD regeneration. Advantages and limitations of whole organ culture models are discussed. Finally, foreseen future applications are presented. Overall, whole organ cultures represent an alternative to preclinical models and help replace, or at least reduce, the need for animal research in line with the 3 R (replacement, reduction, and refinement) principles. Whole organ cultures promote a fast translation of new therapies from bench to bedside.
Keywords: 3 R principles, bioreactor, intervertebral disc, loading, whole organ culture
8.1 Introduction
The healthy state of an intervertebral disc (IVD) is ensured by a fine balance of biological and mechanical cues (see Chapter 1). During disc degeneration, there is a shift toward catabolism of the extracellular matrix (ECM) associated with structural changes that can finally lead to failure of the IVD (see Chapter 2).
Whole organ cultures can provide answers with respect to how changes in biological or mechanical signals can affect the biological and mechanical response of the IVD as a whole organ, as recently reviewed.1,2 For instance, effects of needle puncture, discectomy, biomaterials, and biological therapies on whole IVD response have been investigated.3,4,5,6
Biomechanical questions can be answered by using thawed discs from a small- or large-animal frozen specimen collection (see Chapter 4). However, fresh tissue is required for biological evaluation and discs from large animal models are more representative of the human IVD as they involve high diffusional distances and, in some species, lack notochordal cells (see Chapter 5).
Clinicians can take advantage of whole organ cultures to evaluate new surgical approaches,7 implants, and biological therapies (see Chapters 9–15), thereby collecting useful data for preclinical testing and for further applications in clinical trials. In this respect, whole organ cultures can accelerate the translation from bench to bedside.8
Industry can also benefit from whole organ cultures given they provide a representative model of the targeted tissue that can be used for testing new therapies, compounds, and implants (see also Chapter 20). There is growing interest in the development of culture systems that better represent the in vivo situation. Indeed, there is a global shift from two-dimensional (2D) to three-dimensional (3D) cultures.9 3D cultures (e.g., alginate and agarose hydrogels) have been used for many years and have demonstrated a better maintenance of disc cell phenotype compared with 2D cultures on tissue-culture plastic. However, the hydrogel matrix surrounding the cells is very different from the native IVD tissue. In this respect, whole IVD organ cultures offer several advantages (summarized in ▶ Table 8.1); thus, there is an interest in whole organ culture to become an alternative method for testing new developments, provided that the method is standardized. Such standardization will allow more robust comparison of results among different institutions. Bioreactor-based culture systems represent a recent progress of 3D cultures that allow for reproduction of physiological processes such as perfusion or loading.9
Table 8.1 Advantages and limitations of whole organ cultures compared to in vitro and in vivo models
Advantages | Disadvantages |
•Maintain the cells in their native niche and offer an ideal three-dimensional culture system. •Represent a more robust setup compared with static two-dimensional cell cultures. •Structural changes due to tissue damage or tissue repair are taken into account. •Better control of the culture conditions compared with in vivo studies (e.g., control of loading and nutrition parameters, reproducible degenerative models). •In line with the 3 R principles of animal models, no animal needs to be sacrificed for research purposes if tissue is obtained from slaughterhouses. •Do not require ethical approval when tissue is obtained from slaughterhouses because no animals are specifically sacrificed for research purposes. •Have low costs when using ovine or bovine coccygeal discs, given these can be obtained from slaughterhouses. | •Do not reproduce the complex response (e.g., immune response) of a living organism. •Do not allow biocompatibility tests. •Reaction of the structures surrounding the IVD is not taken into account (these include bone vertebrae, muscles, ligaments, spinal cord, and nerves). •Being more complex than traditional in vitro systems, whole organ cultures are less reproducible. •Application of multiple loading patterns (e.g., flexion/extension, lateral bending, axial rotation) not yet implemented. |
Abbreviations: 3 R principles, replacement, reduction, and refinement; IVD, intervertebral disc. |
8.2 Historical perspective
8.2.1 Culture of Whole Intervertebral Discs without Loading
Since the 1970s, disc slices have been cultured in aqueous solutions. However, if no precautions are taken, such slices swell and lose proteoglycans. Urban and Maroudas demonstrated the prevention of swelling of disc slices cut from frozen human lumbar IVDs by adding polyethylene glycol to the culture medium, thus creating culture conditions iso-osmotic to the IVD environment.10 Recently, Van Dijk et al applied a similar technique to maintain bovine NP explants viable for 42 days, although a downregulation of matrix protein expression was observed.11
Culture of whole IVDs was first performed by Chiba and colleagues using rabbit IVDs.12 They found that embedding IVDs in alginate induced a higher proteoglycan synthesis rate and maintained higher matrix content compared with discs cultured without alginate during the 1-month time frame of the study. Fifteen years later, Risbud et al cultured whole rat IVDs using hyperosmotic medium supplemented with transforming growth factor β (TGF-β) and found that the vitality of the NP cells could be maintained for 1 week.13 In another study, Le Maitre et al found that human IVD tissue structure and cell function could be maintained when the tissue was constrained in a plastic ring during the 3-week culture, whereas this was not the case in free-swelling culture conditions.14
Long-term cultures (49 days) were first reported by Haschtmann et al for rabbit IVDs comprising bony EPs. Cell viability was maintained, although a reduced metabolic activity and a degenerative gene expression profile was found.15 Gawri et al improved the preparation of IVDs for whole organ culture by carefully removing the vertebral bone and only leaving the cartilage EPs.16 This allowed whole human IVDs with intact cartilage EPs to be cultured under free-swelling conditions with preservation of cell viability and ECM homeostasis for 1 month. This was not the case when the IVDs were cultured without cartilage EPs or when vertebral bone was left. Using this technique, culture time was extended to 4 months under freeswelling conditions, with cell viability above 95% in both NP and AF tissues, independent of the concentration of glucose or fetal calf serum in the culture medium.
A model to study IVD regeneration was first introduced by Roberts and colleagues, who used bovine coccygeal discs to mimic disc degeneration by enzymatic digestion with trypsin or papain.17 The enzymatic treatment produced a cavity (~12% of the volume of the disc), which could be used to test injectable biomaterials or biological therapies. One week after trypsin injection, Jim et al found an aggrecan loss of 60%, whereas cell viability was preserved.18
Recently, MSC migration into the disc in response to the chemoattractant stromal cell derived factor-1 (SDF-1) was investigated by Pereira et al using free-swelling whole IVD short-term cultures.19 In this study, a nucleotomy was performed through the EP and the cavity was filled with SDF-1 in saline solution or in a thermoreversible hyaluronic acid grafted with poly(N-iso-propylacrylamide) (HA-pNIPAM) hydrogel carrier. Intact discs, nucleotomized discs solely filled with the hydrogel, and empty nucleotomized discs were used as controls. It was found that the nucleotomy itself induced MSC migration and that this phenomenon was enhanced by the HA-pNIPAM hydrogel. The use of SDF-1 in combination with the HA-pNIPAM hydrogel had a synergic effect on MSC migration.19
Overall, these studies indicate that it is possible to maintain large whole IVDs that are viable for months and that these discs can be used to investigate both degenerative and regenerative processes. Key features for the success of such culture models include the prevention of excessive swelling by hyperosmotic medium, mechanical confinement, or preservation of the cartilage EP; maintenance of the EP represents the condition that is closest to the physiological situation. However, care needs to be taken to conserve the permeability of the EP to allow diffusion of nutrients and waste products into and from the central parts of the IVD during the entire culture period.
8.2.2 Culture of Intervertebral Discs under Loading
It is well-known that IVDs have a tendency to swell when immersed in aqueous solutions. The idea of using mechanical loading to avoid swelling of IVD tissue was introduced in 1981 by Urban and Maroudas.10 By employing a pressure of 0.65 MPa, they could prevent swelling of IVD slices. Static load application to whole bovine coccygeal IVDs was reported by Ohshima et al in 1995.20 They found that when static loads were applied for 8 hours, the matrix synthesis rate was highest under a pressure of 0.13 to 0.26 MPa, whereas both low static pressure (0.01 MPa) and high static pressure (0.4 MPa) decreased the matrix synthesis rate. With the goal to improve nutrient diffusion into the IVD, Lee et al compared IVD cultures with and without vertebral EPs under a static load of 0.25 MPa and medium perfusion for 1 week.21 When IVDs were cultured without vertebral EPs, cell viability and biosynthetic responsiveness could be preserved.
Shifts between two static loads, simulating a diurnal load and a nocturnal rest, represent a progress toward physiological loading. Gantenbein et al used ovine coccygeal discs (systemically anticoagulated before death) perfused with culture medium and maintained them under static load (0.2 MPa) or “diurnal” load (0.8 MPa for 16 hours followed by 0.2 MPa for 8 hours) for 4 days.22 It was found that solutes could diffuse deeper into the IVD when diurnal load was applied.
A more sophisticated development was represented by the introduction of air-controlled systems and the automated application of simulated physiological compressive loading cycles in medium-perfused chambers (▶ Fig. 8.1).23 The simulated physiological loading comprised two periods of sinusoidal loading between 0.4 and 0.8 MPa at a frequency of 0.2 Hz separated by 2 hours of static load at 0.6 MPa, followed by a resting static load at 0.2 MPa for 14 hours. To mimic limited nutrition in the IVD, which is an important factor in disc degeneration, discs were cultured under simulated physiological loading in medium with either limited or sufficient glucose concentration for 21 days. Limited nutrition induced a cell viability decrease of ~50% within a few days and the surviving cells could not compensate matrix production during the time frame of the study. In a follow-up study, Illien-Jünger et al applied this loading pattern with a cyclic load at either 0.2 Hz or 10 Hz to IVDs cultured in medium with limited or sufficient glucose concentration for 1 week.24 It was found that both combinations of limited glucose with low frequency loading and of high frequency loading with high glucose concentration resulted in cell death. Culture in limited glucose and under high frequency loading had an additive effect on cell death and induced gene expression upregulation of the matrix degrading enzyme matrix metalloproteinase 13 (MMP-13). This bioreactor system was also used to induce degeneration in a controlled manner in bovine coccygeal discs and to study regenerative therapies based on MSC homing.25
Fig. 8.1 Specimens and equipment for loaded whole organ culture of ovine or bovine discs. Images from a bovine model: (a) a whole tail, (b) a motion segment, (c) an intervertebral disc (IVD) with endplates (EPs), (d) a sagittal section of the IVD with bony EPs, (e) an IVD bioreactor, (f) a bioreactor chamber for large discs, (g) and a bioreactor chamber for small discs.
Such IVD bioreactor systems generating temporally controlled loading regimes opened the way to the development of other bioreactors capable of applying simulated physiological compressive loading patterns in bovine26 and caprine IVDs.27 Haglund et al optimized the loading regime for coccygeal bovine IVDs and found that disc height loss occurred mainly during preconditioning (48 hours at 0.1 MPa static load) and during the first week of dynamic loading (0.1–0.3 MPa at 0.1 Hz).26 Moreover, when comparing low (0.1–0.3 MPa), medium (0.1–0.6 MPa), and high (0.1–1.2 MPa) load at 1 Hz, cell viability was better preserved under low loading conditions for this type of discs. In another study, Paul et al cultured lumbar caprine discs without load, under low dynamic load (0.1–0.2 MPa, 1 Hz) or under simulated-physiological load (alternating every 30 minutes between 0.1–0.2 MPa at 1 Hz and 0.1–0.6 MPa at 1 Hz for 16 hours, followed by 8 hours of low dynamic load). They found that cell viability, density, and gene expression were best preserved when simulated physiological loading was applied. However, none of the loading regimes had an effect on the ECM content during the 21 days of culture.27In a follow-up study, it was found that both dynamic and static overloading induced pathological changes. Both types of loading induced an upregulation of catabolic and pro-inflammatory genes, as well as water and proteoglycan loss. Dynamic overloading caused cell death in all IVD regions, whereas static overloading mainly affected the outer AF.28
Recent advancements in the design of bioreactors allow the simultaneous loading of several human lumbar discs.29 Walter et al cultured human lumbar discs under compressive loading (0.1 MPa for 12 hours followed by 0.2 MPa for 12 hours) for 21 days and reported a cell viability of ~ 80% for both the NP and AF regions.
The simulation of torsional movements has attracted interest from athletes who actively participate in sports involving torsional movement of the spine and are frequently diagnosed with disc prolapse. Therefore, bioreactors capable of applying torsion were developed by Chan et al.30 Using bovine coccygeal discs, it was found that torsion (1 hour/day at 0.1 Hz during 4 days) at low amplitude (2 degrees) can improve cell viability compared with statically loaded discs, whereas high magnitude torsion (5 degrees) can induce a significant increase in cell apoptosis in the NP. Moreover, cell metabolic activity in the NP decreased with increasing torsion amplitude.
Simulation of complex loading patterns is one of the latest developments of IVD bioreactors. Walter et al studied the cellular and structural response of bovine coccygeal discs to low-magnitude asymmetric compressive loading (15-degree angle).31 They found that asymmetric compression induced cell death, upregulation of catabolic and pro-inflammatory cytokines, and structural disruption. In another study, Chan et al applied compression (0.4–0.8 MPa at 0.2 Hz) and/or torsion (−2 degrees to 2 degrees at 0.2 Hz) to bovine coccygeal discs for 8 hours/day, followed by 16 hours of free swelling for a period of 15 days.32 The effect of combined cyclic compression and torsion was region-specific, with a higher cell death in the NP compared with simple torsion or simple compression, whereas the AF responded with active matrix remodeling.
Taken together, bioreactors represent an ideal setup to study the link between loading and nutrition, two of the main factors considered to be implicated in IVD degeneration.
8.3 Evidence-based Results: Current Applications of Whole Organ Cultures
8.3.1 New Surgical Techniques
Whole organ cultures can provide useful insight when evaluating new surgical techniques. For instance, Vadalà et al recently developed a transpedicular approach to perform nucleotomies. Whereas the feasibility of the technique could be tested on cadaveric spines,7 a whole organ culture model was required to assess the effect of this approach on the viability of the surrounding tissue (▶ Fig. 8.2a).4 By using lactate dehydrogenase staining on cryosections, it was possible to identify which nucleotomy method (purely mechanic, purely enzymatic, or combined mechanic and enzymatic) yielded a reproducible defect while preserving the viability of the surrounding tissue.
Fig. 8.2 Examples of applications of whole organ cultures: (a) X-ray image of the transpedicular approach; (b) whole intervertebral disc (IVD) with annulus fibrosus (AF) defect with 4-point suture; (c) whole IVD with end plate (EP) defect and bone cement sealing; (d) transversal section of an IVD with a 2% w/v agarose hydrogel (stained with methylene blue for better visualization) as nucleus pulposus (NP) filler; (e) fluorescence image of sagittal section of a degenerated IVD (scale bar = 1,000 μm) on which PKH26-labelled mesenchymal stem cells (MSCs) were applied to study cell homing; (f) magnified view of the region marked in image (e), with PKH26-labelled MSCs (red fluorescence) migrating through the EP and in the inner AF indicated with arrows (scale bar = 200 μm).
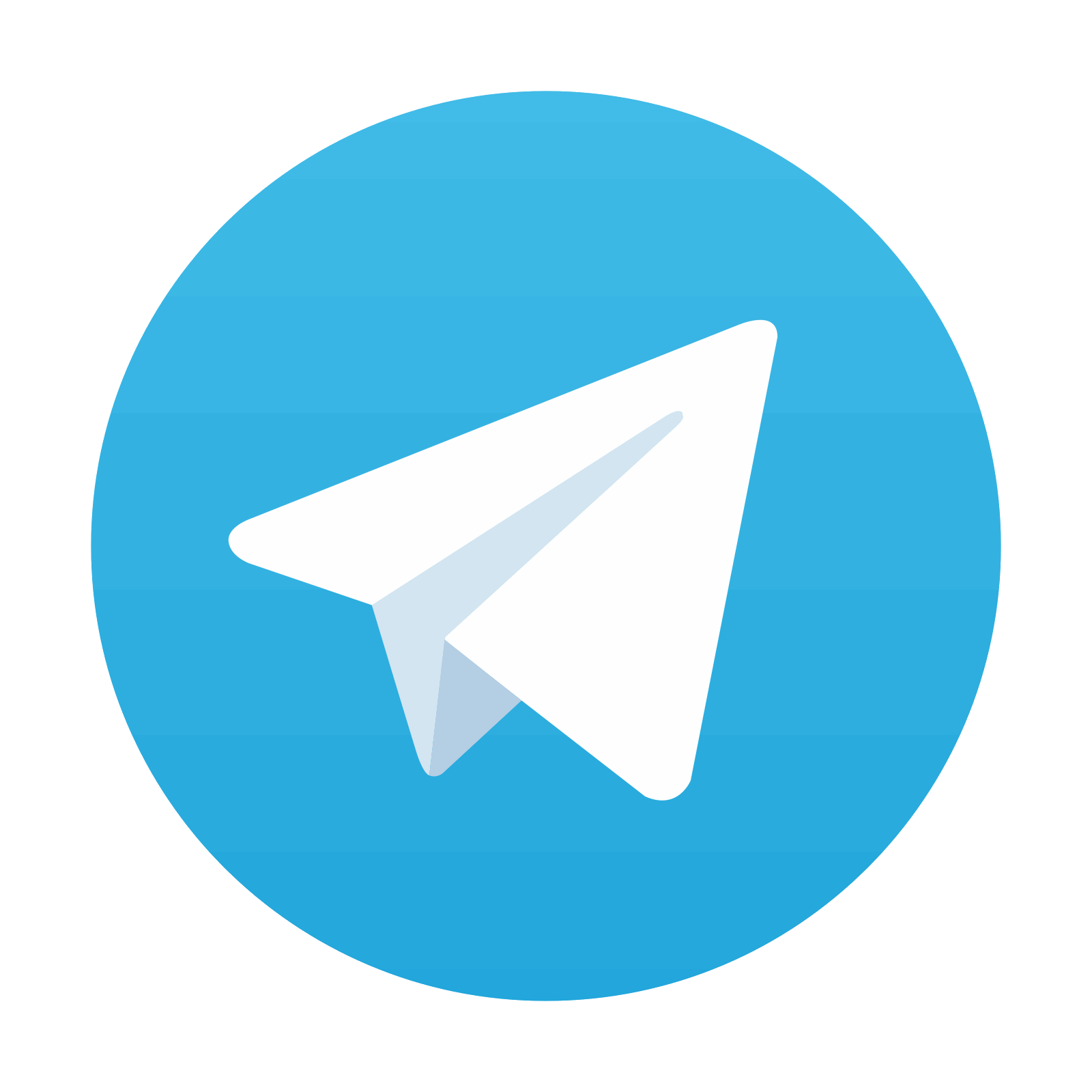
Stay updated, free articles. Join our Telegram channel

Full access? Get Clinical Tree
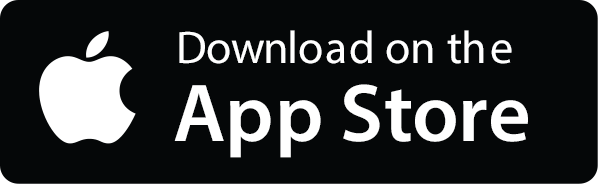
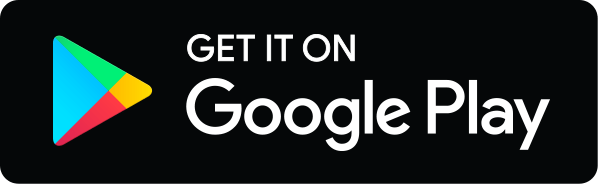