Diagnosis and Medical and Endovascular Treatment of Intracranial Atherosclerotic Disease Objectives: Upon completion of this chapter, the reader should be able to identify the indications for and technical steps involved in treating intracranial atherosclerotic disease with angioplasty, stenting, or some combination. Accreditation: The AANS* is accredited by the Accreditation Council for Continuing Medical Education (ACCME) to sponsor continuing medical education for physicians. Credit: The AANS designates this educational activity for a maximum of 15 credits in Category 1 credit toward the AMA Physician’s Recognition Award. Each physician should claim only those hours of credit that he/she spent in the educational activity. The Home Study Examination is online on the AANS Web site at: http://www.aans.org/education/books/controversy.asp * The acronym AANS refers to both the American Association of Neurological Surgeons and the American Association of Neurosurgeons. The treatment of intracranial atherosclerotic disease has centered on a symptomatic patient population. Patients presenting with transient ischemic attack (TIA) or stroke without cervical carotid disease have increasingly been studied by means of magnetic resonance (MR) angiography. This new trend has been responsible for uncovering an increasing number of patients with intracranial stenosis. When the stenosis correlates with the neurological signs and symptoms, treatment should be initiated. Antiplatelet (aspirin) and anticoagulant (sodium warfarin) therapies have been the focus of medical management. A prospective randomized trial (Warfarin-Aspirin Symptomatic Intracranial Disease [WASID]) to determine whether aspirin or warfarin is more effective for the treatment of symptomatic intracranial atherosclerotic lesions has recently been completed, and the results demonstrated a significantly increased hemorrhage and death rate in the warfarin group (compared with the aspirin group) without any benefit with warfarin for stroke prevention in patients with symptomatic intracranial stenosis.1,2 Technological advancements in angioplasty catheters and stents have driven the application of these devices for revascularization of the intracranial circulation. To date, only small patient series have been reported in the literature. In this chapter, we will review the current literature concerning the natural history of intracranial atherosclerotic disease and primary angioplasty, primary stenting, and provisional stenting procedures for the treatment of symptomatic intracranial atherosclerotic lesions. Methods of diagnosis and patient selection for endoluminal revascularization will be reviewed. Selected case presentations will be made. Finally, complication avoidance and management will be discussed. The management of intracranial atherosclerosis remains perplexing. Unlike for atherosclerosis of the extracranial vasculature, only one prospective randomized trial has influenced therapeutic approaches for intracranial disease. The Extracranial-to-Intracranial (EC-IC) Cooperative Bypass Study demonstrated the inefficacy of bypass surgery to prevent stroke recurrence.3 This study and other smaller prospective studies have allowed us to define the natural history of intracranial arterial stenosis. There are several studies, including the EC-IC bypass study, in which a subgroup of patients was treated with aspirin alone. In the bypass study, 714 patients with intracranial internal carotid artery or middle cerebral artery (MCA) stenosis who received 1300 mg of aspirin on a daily basis were observed. The ipsilateral annual stroke rate was 7%, with an overall stroke rate of 10%. In prospective nonrandomized studies conducted by Craig et al (58 patients) and Marzewski et al (66 patients), distal ICA stenosis carried a 3.2 to 7.6% annual stroke rate ipsilateral to the stenosis, with an overall stroke rate of 4 to 11.6%.4,5 The retrospective arm of the WASID study examined the efficacy of warfarin compared with aspirin for the prevention of major vascular events (stroke, myocardial infarction, or sudden death).1 Among 63 patients treated with aspirin, the rate of a major vascular event was 18.1 per 100 patient-years. The associated stroke rate of 10.4 per 100 patient-years corroborates well with that of other studies. Among patients receiving warfarin, however, the major vascular event rate decreased to 8.4 per 100 patientyears in 88 patients. The stroke rate was 3.6 per 100 patient years. Three patients (3%) had major hemorrhagic complications, with two being fatal. The rate of hemorrhagic complications for the warfarin-treatment group was 7.8 per 100 patient-years, compared with 1.4 per 100 patientyears for the aspirin-treatment group.1 In addition, a European trial, Stroke Prevention in Reversible Ischemia Trial (SPIRIT), has demonstrated a high hemorrhagic complication rate associated with warfarin therapy in patients who have experienced previous cerebral ischemic events, adding to the complexity of medication choices.6 The mechanism by which warfarin eliminates strokes is unclear. In the WASID trial, warfarin treatment resulted in near-elimination of strokes in territories outside the significant stenosis.1 Among six patients who had strokes, five strokes were in the territory of the compromised vessel. With aspirin, 15 strokes occurred, of which 9 were in the territory of the stenotic artery. This suggests that warfarin may affect other pathophysiological mechanisms of stroke besides large artery occlusive disease (e.g., mechanisms such as small vessel arteriopathy or cardioembolic events). Cardioembolic sources of stroke may well be prevalent in this population, and warfarin may be a more effective treatment for these embolic sources than aspirin. The WASID study also provides interesting data on posterior circulation events, with respect to daily aspirin use.1 For posterior circulation stenosis, stroke rates approached those for symptomatic extracranial carotid stenosis exceeding 70%. For intracranial vertebral artery stenosis, a 7.8% annual stroke rate was found, whereas basilar artery stenosis was associated with a 10.7% annual stroke rate. Further studies have implicated a very high recurrence of neurological symptoms once antithrombotic therapy has failed.7 Recurrent ischemic events typically occur within 1 month after failure of therapy.7 There is, therefore, some urgency to change the form of treatment once failure has occurred. In addition, most patients with intracranial disease are more likely to present with a major event (such as a catastrophic stroke) than with TIAs or minor stroke.3 This further complicates medical decision-making, as the question arises whether medical management should even be attempted initially. There is considerable debate about the mechanism responsible for large vessel intracranial stenosis that results in stroke. Unlike the extracranial carotid where artery-to-artery embolism is the predominant mechanism, increasing evidence in the literature supports a hemodynamic cause for large vessel infarctions. Certainly, a subset of patients has demonstrated a higher rate of stroke recurrence associated with hemodynamic failure. 8–10 More likely, there is an ongoing interaction between the stenosis providing an embolic source and reduced blood flow resulting in diminished ability to clear the emboli. The ischemic events are thus a shift in the balance between embolic load and blood flow.11 Another medical concern regarding stroke prevention is hypertension. Blood pressure control is the most important factor in preventing recurrent stroke even in patients with evidence of impaired cerebrovascular reserve.12 Hypertension is the most important risk factor for small vessel disease in penetrating arteries and large vessel atherosclerosis, yet aggressive control of blood pressure may precipitate ischemia in a territory with diminished cerebrovascular reserve. Therefore, a difficult medical decision must be made as to the optimal blood pressure for patients with stenosis and functional tests that suggest relative hemodynamic insufficiency. Bypass surgery limitations probably relate to the reasons mentioned above. Bypass surgery can improve cerebral blood flow and reduce hemodynamic risk of future strokes, but it has little ability to reduce artery-to-artery embolism. The EC-IC bypass study failed to demonstrate a reduction in stroke frequency, partly because the patient population could not be selected to a hemodynamic failure arm.3 In addition, the EC-IC bypass procedure would occasionally precipitate MCA occlusion at the site of the stenotic segment (usually the M1 segment). 13,14 This thrombosis likely causes perforator occlusion, resulting in deep brain infarction. The Carotid Occlusion Surgery Study (COSS) is evaluating the rate of stroke reduction after the performance of EC-IC bypass procedure in patients with symptomatic carotid occlusion and an increased oxygen extraction fraction, as measured by positron emission tomography.15 In summary, aspirin has demonstrated only mild efficacy for stroke prevention. Warfarin was associated with significantly higher rates of intracranial hemorrhage and provided no benefit over aspirin in the WASID prospective trial.2 Endoluminal revascularization, therefore, may have a role in the treatment of both embolic and hemodynamic sources of infarction from the stenotic vessel. A brief explanation of the angiographic appearance of intracranial atherosclerosis is a necessary prelude to a discussion of endovascular techniques for the treatment of this condition. Intracranial stenosis is a dynamic process whereby repeat imaging can reveal sometimes dramatically different degrees of arterial blockage. In a retrospective series of serial angiographic studies obtained to determine the natural history of intracranial stenosis, 40% of stenoses progressed and 20% regressed.16 The distal internal carotid artery did not seem as predisposed to disease progression as did the more distal branches (MCA, anterior cerebral artery, posterior cerebral artery). In three patients, regression of the intracranial stenosis was impressive, suggesting that a thrombus was present within the already diseased vessel. More recently, significant angiographic improvement of a stenotic vertebrobasilar artery segment was seen after the administration of highdose atorvastatin, a potent HMG-CoA reductase inhibitor, for a 2-week period.17 This improvement may have been from resolution of thrombus as statins promote endogenous fibrinolysis and plaque remodeling. The three methods for achievement of endoluminal revascularization are primary angioplasty, primary stenting, or provisional stenting. Primary angioplasty means that the physician is placing an angioplasty balloon to expand the stenotic segment and does not intend to place a stent. Primary stenting means that the physician intends to place a stent and may or may not dilate the lesion with an angioplasty balloon before stent placement. If no previous dilation is required, the procedure is referred to as direct stenting. Initial patient series presented the feasibility and demonstrated the limitations of primary angioplasty alone.13,18–20 More recent series have documented the decreased incidence of complications, demonstrating a considerable learning curve for these techniques.21–24 The periprocedural neurological event rate in these four series, which comprise more than 10 patients each, is less than 10%,21–24 whereas the event rate in the four initial studies, comprising more than 10 patients each, ranged from 8 to 33%.18–20,25 The results of these numerous reports are mirrored in the angioplasty series presented by Connors and Wojak.21 These authors divided their experience from 1989 to 1998 into three periods. A higher rate of complications was encountered in 17 patients treated during the early and middle periods from 1989 to 1993, including dissection, 82%; neurological events, 6%; and death, 6%. Subsequent to 1993, the rates among 41 patients were dissection, 14%; neurological events, 8% (of which 4% were TIAs); and death, 2%. Connors and Wojak attribute the improvements to decreasing the balloon diameter to restore the vessel lumen, very slow inflation of the balloon (over 2 to 5 minutes), and the routine use of glycoprotein IIb/IIIa receptor inhibitors, such as abciximab, during angioplasty. Two hemorrhages (included in the neurological events rate) occurred during this period. They also avoid crossing a lesion more than once with the angioplasty balloon because that maneuver is likely to raise an intimal flap and cause the vessel to become occluded. One intrinsic advantage that endovascular approaches have over surgery is the ability to re-angioplasty. A stenotic vessel that has been suboptimally dilated initially can be further dilated on subsequent interventions. Another “pearl” discovered by these authors is the use of shorter angioplasty balloons to prevent straightening of the intracranial vessels after balloon inflation, making an injury or dissection less likely. Mori et al demonstrated in several published case series the effectiveness of angioplasty in patients with short (≤5 mm), mildly eccentric or concentric (type A) lesions.23 In their hands, these lesions had a periprocedural complication rate of 8% (1 stroke in 12). During the 2-year follow-up period, no ipsilateral stroke, neurological event, or angiographic stenosis occurred, and no bypass surgery or repeat angioplasty was needed. In angiographic lesions that were longer and more eccentric or chronically occluded, the procedure yielded less effective results. For lesions that were 5 to 10 mm in length or totally occluded and were less than 3 months from initial angioplasty (type B), the success rate was 86%. Angiographic restenosis occurred in 33% of the lesions within the 2-year follow-up period. Angioplasty attempts were unsuccessful in 2 of 21 patients. Patients with chronically occluded lesions that were 3 months or older or highly angular or long (>10 mm in length; type C) fared the worst. Angioplasty was associated with an initial success rate of 33% (3 of 9 patients) and a restenosis rate of 100% at 1 year. These results suggest that angiographic characteristics may help determine feasibility and periprocedural risks. One of nine patients with type C lesions experienced a stroke from abrupt closure of the stenotic vessel, suggesting that vessels harboring these lesions are extremely tenuous. The cumulative risk of ipsilateral stroke was 12% for type B lesions and 56% for type C lesions. Of note, the natural history of these lesions was not delineated according to lesion type in either the WASID study or the EC-IC bypass study. The studies by Mori et al26,27 suggest that type C and possibly type B lesions as well should not be treated by angioplasty alone but rather may benefit from another endovascular technique or surgery. In another series, 20 patients with intracranial vertebrobasilar stenosis were treated with angioplasty alone.24 One dissection resulting in a stroke and one TIA occurred, for a 10% complication rate. Restenosis occurred in 14% during an average follow-up period of 8 months. The two cases of restenosis were in patients in whom residual stenosis of 20 to 30% remained at the end of the revascularization procedure. Marks et al report a low periprocedural risk of 5% in their intracranial angioplasty series.22 These authors also undersize the balloon and allow for residual stenosis. They frequently include anticoagulation (warfarin) therapy in their postprocedural regimen (prescribed for 18 of 23 patients), particularly if there was significant (>50%) residual stenosis or dissection. Two complications occurred in the immediate postprocedural period among this group of 23 patients. A vessel ruptured, which resulted in death, and an angioplasty site became occluded by a thrombus. The clot was successfully lysed with intraarterial tissue plasminogen activator. Two strokes occurred during the respective follow-up periods of 37 and 32 months. Only one of the strokes involved the territory supplied by the treated vessel. This stroke occurred in a vessel with 50% residual stenosis. Including the vessel rupture, the annual rate of stroke in the territory of the previously treated vessel was 3.2%, and the overall rate of stroke during the average 35.4 months of follow-up was 4.8%. This dramatically low frequency of strokes should be acknowledged because it occurred while warfarin therapy was often used in conjunction with revascularization therapy. This combination of therapies may best reduce the risk of strokes from hemodynamic, embolic, and small vessel arteriopathy sources. In summary, angioplasty remains a new modality of therapy for intracranial atherosclerotic disease. Although no prospective randomized trials are available for review, numerous retrospective series suggest that this approach has promise. In all of the series, numerous dissections occurred, and mortality was usually a result of vessel rupture or wire perforation. Strokes occurred rarely during the procedure, but on occasion, emboli were generated; and the vessels at greatest risk for restenosis or further strokes were those with residual stenosis. Furthermore, angioplasty eliminates one of the factors confounding optimal blood pressure management. With aggressive blood pressure control, no ischemia should be precipitated. Procedural improvements are ongoing. New developments in wire technology and the introduction of catheters with soft hydrophilic coatings have made perforations rare. Undersizing the balloon makes rupture unlikely. Embolic phenomena can now be treated with medications such as glycoprotein IIb/IIIa receptor inhibitors. A caveat is that the use of IIb/IIIa inhibitors may increase the hemorrhagic risk of these procedures. In the future, protection devices may be available to collect plaque debris during angioplasty. This technology, whether it involves a filter or a balloon aspiration device placed distal to the angioplasty site, is on the horizon and is currently being used in the extracranial carotid distribution. Dissections can be prevented by use of either slower inflation techniques or shorter balloons (of note, the balloon length still must span the entire stenotic segment). If dissection does occur, intracranial stents can be placed to tack the intimal layer down. Stents have been used to improve the results of coronary angioplasty by restoring vessel luminal diameter and preventing restenosis. This strategy should apply to the intracranial circulation. Self-expanding stent characteristics of less radial force and enhanced navigational flexibility represent the technology that is advancing to become the appropriate tool for the treatment of intracranial stenosis. Unfortunately, stent navigation in tortuous anatomy can be hazardous, sometimes leading to complications. There are two series of at least 10 patients each who underwent intracranial primary stenting for atherosclerotic lesions.28,29 Both report excellent results with minimal residual stenosis and no neurological complications. Mori et al were unable to deliver a stent in two of 12 lesions.29 They were able to successfully angioplasty these lesions. Improvements in trackability and flexibility will make stents easier to deliver. In the series reported by Mori et al, two lesions were type C, and eight lesions were type B lesions.29 One type C lesion could not be accessed for stent delivery, which again demonstrates the difficulty in treating this kind of lesion. No restenosis or neurological events have been reported in the early postprocedural (>4 months) period. Gomez et al reported that three of their 12 patients had headaches and two had cranial nerve deficits, all of which resolved in 8 to 12 weeks.28 The cranial nerve deficits were likely related to nerve injury resulting from basilar artery movement during stent placement. Ramee et al proposed a combined approach.30 They used primary angioplasty for revascularization. If the lesion was complex or long, primary stenting was attempted. If the results of primary angioplasty were suboptimal because of either dissection or vessel recoil with residual stenosis, stenting was used. The combined approach yielded an excellent short-term outcome with a 93% success rate and a 53% “unexpected benefit” rate in that 8 of 15 patients had reversal of what was initially thought to be a permanent deficit from a prior stroke. This method is described in the coronary literature as provisional stenting; it has become popular for coronary revascularization. Levy et al presented a series of 10 patients who underwent stent placement for medically refractory, symptomatic atherosclerotic disease of the basilar artery at our center.31 Four patients were treated with direct stent placement, three with a staged procedure (angioplasty followed ≥1 month later by stent placement with or without repeated angioplasty), and three with conventional stent placement. In the group treated with direct stent placement, a dense quadriparesis developed in two patients after the procedure, with infarction of the ventral pons in these patients. In the staged stent placement group, no permanent neurological complications occurred after the procedure, and in the conventional stent placement group, one of three patients experienced a neurological complication involving homonymous hemianopsia. Based on these data, direct stent placement in the basilar artery is associated with a relatively high complication rate, compared with a staged procedure. Complications may result from an embolic shower following disruption of atheromatous plaque debris by high-profile devices such as stents, as demonstrated by the postprocedural imaging appearance of acute pontine infarctions. Additionally, displacement of debris by the stent into the ostia (snowplowing) of small brainstem perforating vessels may be responsible for the complications noted. We use endoluminal revascularization for only symptomatic patients with high-grade intracranial stenosis because of the intrinsic risks of these procedures. A screening MR angiogram is performed that documents the target lesion. Direct correlation between the neurological deficit and the lesion location must be made prior to treatment. Dizziness alone, without a corresponding neurological deficit, is not adequate motivation for revascularization of the anterior circulation. Initially, we treat all patients with antiplatelet therapy using aspirin and clopidogrel before consideration of the patient for revascularization. Patients in whom pharmacologic treatment has failed are scheduled for revascularization. We recently modified our treatment regimen after a few patients, each with posterior circulation atherosclerosis, suffered devastating strokes while receiving pharmacologic therapy. At present, patients with severe stenotic symptomatic lesions are considered for revascularization primarily. Cerebral blood flow measurements have played an increasingly important role in the treatment of symptomatic atherosclerotic lesions. Single-photon emission CT (SPECT) scans are used to study vascular perfusion and reserve.8,12 Patients with symptomatic high-grade lesions and evidence of severe hypoperfusion ipsilateral to the stenosis are good candidates for revascularization. Patients with poor vascular reserve, as evidenced by acetazolamide-challenge SPECT, are also good candidates for revascularization. Computerized tomography (CT) perfusion techniques are being evaluated and analyzed to determine their correlation with actual vascular perfusion.32–34 At our center, perfusion CT scans have gained acceptance as a means of studying vascular perfusion and reserve. Patients with recent infarctions are considered for treatment within 2 to 4 weeks of the ictus. The volume of the infarction governs the timing from ictus until treatment. Large infarctions are permitted to stabilize as a means of reducing the risk of reperfusion injury. In addition, neurological signs and symptoms fluctuate in the immediate period after the onset of stroke, which makes interpretation of the periprocedural examination difficult. It is crucial that outcomes be monitored carefully during our early experience with device-based intracranial revascularization. Before patients are considered for intracranial revascularization, it is essential that the cervical carotid artery, carotid artery origin, vertebral artery, and vertebral artery origin be free of significant atherosclerotic disease. Cervical carotid disease can be easily imaged by use of duplex ultrasonography. Vertebral artery or carotid origin disease cannot be adequately imaged by use of this technology. MR angiography can provide adequate images of the aortic arch and vertebral artery, especially if gadolinium bolus techniques are used. We have found that digital subtraction angiography is the “gold standard” for uncovering lesions in these locations. If significant stenosis within the proximal vasculature is discovered, consideration should be given to a staged revascularization. The proximal lesion can be treated first. The intracranial lesion can be treated within 6 to 8 weeks after the proximal lesion has healed. Sometimes, the flow limitation created by the proximal lesion will make the distal lesion appear more severe on the angiographic image. Patients undergo routine preprocedural testing that includes blood cell count, electrolytes, EKG, chest X-ray, and coagulation profile. They are maintained on aspirin and clopidogrel for 72 hours before the procedure. Preprocedural inspection of lower-extremity pulses and distal perfusion are helpful as a means of avoiding periprocedural lower extremity ischemia. The procedure is performed after midazolam and fentanyl have been administered to induce mild neuroleptic analgesia. Oxygen saturation, heart rate, blood pressure, urine output, and neurological status are monitored throughout the procedure. A 6-French sheath is placed into the femoral artery. This sheath is perfused with heparin saline flush (4 U heparin per cc of normal saline solution). A 5-French Sim II catheter (Terumo Medical, Somerset, New Jersey) is used for access to the brachio-cephalic vessel. A three- or four-vessel, diagnostic contrast X-ray angiogram is performed to facilitate lesion characterization and to assess collateral circulation. Measurements are made of the target lesion. Proximal and distal vessel diameters and lesion length are measured. The tortuosity of the intracranial vascular pathway should be carefully assessed. In some cases, tight curves or bends in the artery that are proximal and distal to the lesion may prevent delivery of devices to the target vessel. Anticoagulation Anticoagulation is initiated by use of an intravenous or intraarterial bolus of heparin in the range of 70 U/kg. All saline flush bags are infused with heparin as previously mentioned. Activated coagulation time (ACT) is measured and maintained within the range of 250 to 300 seconds. Numerous practitioners have suggested the use of intravenous IIb/IIIa inhibitors as a means of preventing acute or subacute thrombosis in instrumented plaque. Although this has been widely accepted in cardiology, judicious application of this pharmacologic therapy in neurointerventional procedures is warranted. Revascularization of a chronically ischemic vascular bed can result in reperfusion hemorrhage. Bleeding in the face of platelet-receptor blockade can result in uncontrolled intracranial hemorrhage and death. Careful blood pressure monitoring and aggressive blood pressure control are tantamount to safe use of IIb/IIIa inhibitors in this patient population. If IIb/IIIa inhibitors are to be used, a smaller bolus of heparin (40 or 50 U/kg) is given with an ACT objective of 225 seconds. Surgical Technique The procedure is performed by use of a biplane digital subtraction angiography (DSA) fluoroscopy unit (Toshiba Medical Systems, Tustin, California). After the diagnostic catheter is positioned in the target vessel and the ACT has been adequately adjusted with heparin, an exchange-length glidewire (Terumo Medical, Somerset, New Jersey) is placed through the diagnostic catheter into the target vessel. The diagnostic catheter is then removed. A 6-French straight Envoy catheter (Cordis Neurovascular, Miami Lakes, Florida) is placed over the wire and into the internal carotid or vertebral artery. The position of the catheter is critical. If a stent is to be used, the catheter tip should be placed as distal in the target vessel as possible. Positioning of the guide catheter can lead to spasm, vessel telescoping, and dissection. Roadmap fluoroscopy can be very useful to avoid vessel injury. Once the guide catheter is in position, a micro-catheter (Prowler14, Cordis Neurovascular) and microwire (Transcend Wire; Boston Scientific Scimed, Natick, Massachusetts) are used to cross the lesion. The microcatheter is advanced through the lesion into the distal target vessel. The microwire is removed, and a 300-cm exchange length 0.014-inch wire (light- or medium-weight balance, Guidant/ACS, Temecula, California) is placed through the microcatheter. The microcatheter is then removed. Great care must be taken to ensure that the position of the distal wire does not change during device exchange. Revascularization Strategies The strategy governing the choice of devices for revascularization is fueled with controversy. Initially, the use of intracranial stents was reserved for patients who had failed previous angioplasty because of significant vessel recoil or dissection. This strategy is supported by the work of Connors and Wojak, who advocate angioplasty with a low-profile coronary balloon.21 They routinely avoid oversizing the balloon relative to the vessel diameter. They perform slow inflation and deflation of the balloon at low pressures, typically below 6 atm. In this way, the plaque is stretched and not cracked. They postulate that this prevents dissections and limits vessel recoil. Connors and Wojak have not seen a need for intracranial stent placement. Their angioplasty series includes more than 50 patients. In Buffalo, we advocate the use of staged angioplasty and stent placement.35 We stent vessels after angioplasty whenever the anatomy of the vessels permits safe delivery of the device. Preliminary results, albeit without long-term angiographic follow-up, indicate a reduced rate of restenosis. To facilitate balloon delivery, the shortest balloon length that covers the lesion should be chosen. We routinely undersize the balloon relative to the vessel diameter; for example, for an MCA lesion, a balloon diameter of 1.5 or 2 mm would be chosen. For short lesions, a 10-mm length balloon would be used. For longer lesions, a 15 or 20 mm balloon can be used. Longer balloons may be more difficult to deliver and may require distal wire purchase. Inflations are kept below 8 atm, and the balloon is slowly inflated and deflated. Stent delivery, placement, and deployment involve a steep learning curve. Our experience with intracranial stents was preceded by a robust animal experience.36 We would suggest practice in models prior to clinical application. As mentioned previously, the decision to perform stent placement should be based on postangioplasty results. Excessive vessel recoil or dissection may mandate stent placement. The stent diameter should be larger than the vessel diameter by ~10 to 20%. The stent length should be chosen so that the entire lesion will be covered with an additional 1 to 2 mm overlap onto the healthy vessel on each side. Stent delivery can be difficult, especially when the radius of curvature of the carotid siphon or vertebral artery near the C1 vertebra is low. It is critical that the wire has a firm purchase in the distal vessel. For a stent to be positioned in the supraclinoid carotid artery, the wire should be positioned in the M2 or M3 segment of the ipsilateral MCA. For a midbasilar stenosis, the wire should be positioned in the P2 or P3 segment of the posterior cerebral artery. During delivery, the position of the distal end of the guide catheter should be carefully monitored. As the stent impacts tight turns in the vasculature, the guide catheter may back out. This movement can result in loss of guide catheter position and distal wire purchase. Great care must be taken to advance the guide catheter distally to ensure adequate support for stent delivery. On occasion, a second wire (VI8 Control; Boston Scientific Scimed, Natick, Massachusetts) is placed in the guide catheter for improved stability. Once the stent has crossed the lesion, careful attention must be paid to positioning. Prior to deployment, we routinely perform numerous DSA studies to ensure that the lesion is adequately covered by the stent. Rarely will patients become symptomatic due to flow obstruction caused by the stent before deployment. It is advisable to take time to ensure that the stent is positioned properly prior to deployment. Deployment should be performed slowly and evenly. The balloon should be expanded fully, and the stent should be imbedded into normal vessel proximal and distal to the lesion. A DSA study performed after the procedure should confirm placement, patency, and apposition of the stent to the wall of the vessel. Poststent angioplasty can be performed with a slightly larger balloon if needed. If the stent does not adequately cover the lesion, another stent should be placed. Sluggish flow or evidence of thrombus in the stent may require the administration of IIb/IIIa inhibitors to prevent acute or subacute thrombosis. Runoff in the distal vasculature must be carefully examined to ensure that distal embolization has not occurred. At the conclusion of the procedure, the effect of the heparin is allowed to reverse on its own. The groin sheath can be left in place and removed when the coagulation cascade has normalized, after which pressure can be applied to the groin region to ensure hemostasis. Alternatively, a femoral artery closure device can be used while the patient is receiving anticoagulant or antiplatelet therapy. In the perioperative period, blood pressure is closely monitored and controlled. Clopidogrel is continued for 30 days. Aspirin is continued for the life of the patient. This 73-year-old man presented with unstable angina and prior coronary angiography demonstrating three-vessel coronary disease. He was being prepared for coronary artery bypass grafting and had a 1-year history of right hemisphere TIAs. Diagnostic cerebral angiography revealed a 90% petrous carotid stenosis (Fig. 19-1). A brain SPECT scan using 99 m Tc-bicisate (Neurolite; DuPont Pharmaceutical Co., Wilmington, DE) demonstrated hypoperfusion of the right internal carotid distribution—particularly of the watershed areas in the dorsolateral MCA and between the anterior cerebral and middle cerebral arteries. Petrous carotid revascularization was indicated to reduce his perioperative stroke risk, particularly in the setting of hypotension during bypass (Fig. 19-1B-D). The natural history of intracranial atherosclerosis must be well understood to better make decisions regarding who should undergo endovascular revascularization and when treatment should be performed. Our techniques have evolved such that we perform revascularization in symptomatic patients with high-grade intracranial stenosis and blood-flow assessment revealing hypoperfusion in the threatened territory. We are increasingly using stent-assisted angioplasty for complex plaques as well as when initial angioplasty results are suboptimal. The decision to perform revascularization becomes more difficult when hypoperfusion on SPECT imaging is not demonstrated or the patient has not failed a course of treatment with antiplatelet agents. This group of patients could be candidates for endovascular revascularization. Another challenging group includes asymptomatic patients with magnetic resonance angiography evidence of intracranial stenosis. We do not treat these patients unless SPECT imaging reveals moderate or severe hypoperfusion or lack of reserve in a patient who is to undergo a coronary bypass procedure. Our techniques for endoluminal revascularization of intracranial stenosis have evolved in accordance with the literature. We use short, undersized angioplasty balloons with slower inflations. We continue to administer two different antiplatelet agents for 72 hours before the procedure and may use IIb/IIIa inhibitors during revascularization of longer, ulcerated lesions. We use stent-assisted angioplasty to rescue prior angioplasty dissections or occlusions.21 We use staged angioplasty and stent secondarily to reduce residual stenosis. Given the constant evolution of microcatheters, microwires, and angioplasty balloons and stents, our ability to treat these lesions continues to improve. There appears to be a role for revascularization, particularly in the concentric, short stenosis. FIGURE 19-1 (A) Preoperative right internal carotid arteriography in reverse oblique orientation demonstrates high-grade petrous carotid artery stenosis. (B) A microcatheter (Rapid Transit, Cordis Neurovascular, Miami Lakes, FL) was advanced with an AllStar microguidewire (ACS; Guidant, Santa Clara, CA). After being placed across the lesion, the microcatheter was exchanged for a 2 × 11-mm coronary artery balloon catheter (Chubby; Boston Scientific Schneider, Plymouth, MN). These devices were exchanged after two balloon angioplasty inflations were completed. Improvement in the lumen is seen, but significant stenosis of the vessel and irregularity within the plaque remain. (C) The Chubby balloon catheter is exchanged for a 4 × 12-mm AVE GFX coronary artery stent (Medtronic AVE, Santa Rosa, CA). This is after initial deployment of the stent. There is considerable improvement in the lesion. No significant stenosis remains. (D) Oblique view demonstrates improvement in luminal diameter and a marked improvement in flow through the middle cerebral artery.
Natural History and Medical Management of Intracranial Stenosis
Pathophysiology of Cerebral Infarction from Arterial Stenosis
Angiographic Progression of Intracranial Atherosclerosis
Endoluminal Revascularization
Patient Selection and Preparation
Pre- and Perioperative Procedure
Case Study
Conclusion
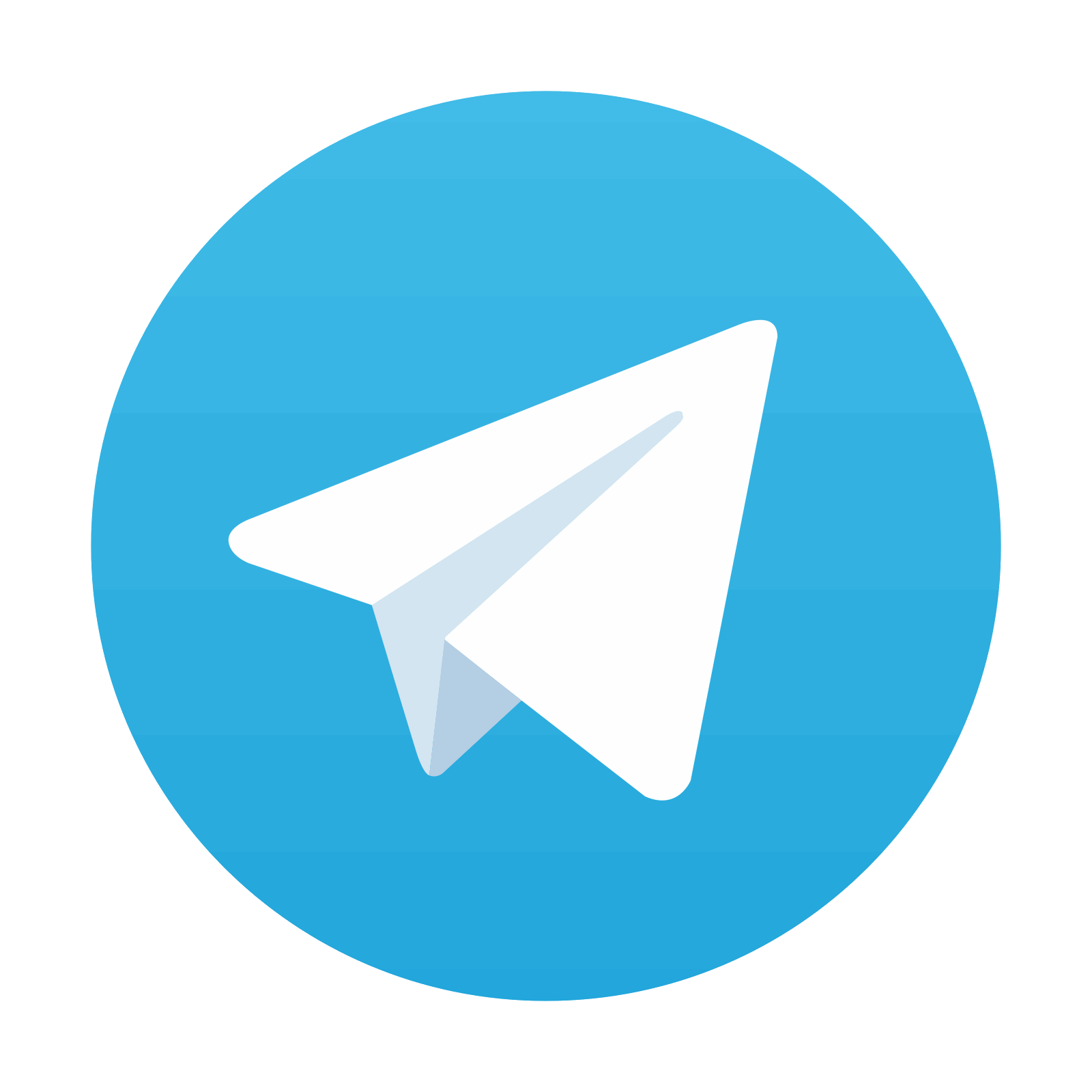