Chordomas are infiltrative tumors located within the intricate anatomy of the central skull base. It is well established that radical resections with no gross residual is associated with better prognosis. However, the invasive growth pattern of chordomas within the bone and their undetected indolent course until they reach considerable sizes creates a complicated clinical picture. 1,2,3 Tracking the tumor for a gross total removal is oftentimes beyond the limits of exposure of one single approach, and unexpected and undetected tumor remnants are frequently found at postoperative imaging. 4 Similarly, aggressive surgeries also put neighboring neurovascular structures in jeopardy. This is where intraoperative assistive technologies come into use: intraoperative assistive technologies aid the surgeon in (1) localizing the tumor, (2) reaching anatomical margins of the tumor, and (3) protecting neighboring vital neurovascular structures. Among the many technologies that have been applied in chordoma surgery, four are more prominent: neuronavigation, intraoperative magnetic resonance imaging (MRI), neuroendoscopy, and intraoperative neuro monitoring. These techniques will be discussed in this chapter individually.
23.2 Impact of the Extent of Resection Disease Course and Tumor Biology in Chordomas
All of these intraoperative assistive technologies were developed to increase the extent of surgical resection and to increase operative safety. One would certainly ask whether this effort to increase the extent of resection is justified and whether a more conservative approach based on a simple biopsy would be more sufficient. As chordomas are rare tumors, prospective clinical trials are exceptionally difficult to make. Despite this fact, compelling evidence has accumulated through the years indicating that patients benefit from surgery and to its extent. This efficiency is observed for chordomas in the skull base, in the spine, and in the sacrum. This finding was not unexpected, as it is well established that surgical excision can significantly alter the clinical course of slow-growing neoplasms such as meningiomas and low-grade gliomas. This effect remains the main justification of skull base surgery for benign tumors such as meningiomas and schwannomas. The positive effect of surgery on patient survival has also been demonstrated for other primarily intraosseous neoplasms of the skull base, spine, and sacrum, including chondrosarcomas, Ewing’s sarcomas, and osteosarcomas. 5,6 Apart from the efficiency of surgery, the inefficiency of other techniques also augments the importance of surgical treatment. Several studies have shown that unless chordomas are very small in size, radiotherapy and radiosurgery are of limited effect and that chemotherapy has no effect on chordomas. These findings indicated that surgery is the only effective form of initial treatment for large chordomas. Since the popularization of skull base surgery in the 1980s, case series have persistently shown that surgery has a positive impact on patient survival. Early studies have demonstrated in case series the feasibility, safety, and efficiency of extensive resections for skull base chordomas. 4,7,8,9,10,11,12,13,14,15,16,17,18,19,20,21,22,23,24,25,26 The observation in these studies were also regularly reviewed and critically analyzed. 23,27,28,29,30 However, due to the rarity of the disease, all of these studies report cohorts of less than 100 patients. To overcome the limitations related to small patient numbers, different groups used alternative strategies such as performing meta-analyses or reviewing public registries and databases, and all of these analyses have resulted in the same conclusion that the surgery and its extent have a significant impact on survival in patients with chordoma. Di Maio et al 27 performed a meta-analysis of 807 patients in 23 studies that have met the inclusion criteria (among studies reported between 1999 and 2011). The authors concluded that when compared with totally resected cases, subtotally resected chordoma patients were 3.83 times more likely to experience a recurrence event (95% confidence interval [CI]: 1.63–9.00) and 5.85 times more likely to die of progressive disease (95% CI: 1.40–24.5) at 5 years. 27 The same study also compared 5-year progression-free survival in 304 patients with complete resections and 222 patients with incomplete resections using a random-effects model and concluded that 5-year progression-free survival was 20.74% higher in patients with complete resections. The same significant difference in 5-year progression-free survival was observed using a Kaplan–Meier analysis. 27 Similar results have also been found in analysis of chordoma tumor registries. Independent groups analyzed the outcomes of patients with chordoma using the Surveillance, Epidemiology, and End Results (SEER) database and concluded that patients undergoing surgical resection survived significantly longer than those that did not, independent of other treatments that the patient might have received. 2,3,6,31
It must be kept in mind that surgery decreases the total disease volume in the patient but that it does not impact the growth rate of the tumor. Therefore, the postsurgical residual tumor volume is a more important determinant rather than the extent of resection. In turn, the postoperative residual tumor volume is a function of the initial tumor volume, the invasion pattern, and the surgical efficiency (extent of resection) and has a direct influence on patient survival. 32 Initial reports of common treatment failures with fractionated radiation has created a nihilism and reluctance in the surgical community to administer radiotherapy to residual chordomas. But further advances in chordoma biology has taught us that chordomas are in fact radiosensitive but require high energy to achieve this goal, which can be efficiently provided by photon or hadron radiosurgery as long as the tumor does not exceed a critical volume. Eid et al 33 compared the outcome of a cohort of 30 patients treated with “wide resection,” “subtotal resection,” or “nonsurgical techniques” and concluded that with the addition of radiosurgery, similarly good results can be achieved with wide or subtotal resection. Combination of extensive surgery and application of high-dose focal radiation for small tumor residuals has largely been accepted by the surgical and radiation therapy communities. But residual tumor volume is very critical and is the key to success in chordoma surgery. Today, the aim of the surgical treatment is either to eliminate the disease completely (in small tumors) or to transform it into a more localized form to make it treatable by high-dose radiation therapy. Studies analyzing the effect of radiotherapy, radiosurgery, or charged-particle radiation as adjuvant therapies after surgical resection of chordomas have consistently emphasized that the volume at the start of these treatments determines their success. 34,35,36,37,38,39,40,41,42,43,44,45,46,47 Local treatment failure is significantly more common in larger tumors independent of the form of radiation treatment, be it fractionated radiotherapy, intensity-modulated radiation therapy (IMRT), radiosurgery, or charged-particle radiation therapy. High marginal doses (minimum of 15–16 Gy) are required for local control of chordomas using radiosurgery, and the main limitations of the prescribed dose are large tumor volume and proximity to vital and radiosensitive neurovascular structures. 34,37,38 Reports of fractionated radiotherapy (including IMRT) also cite that the postoperative residual tumor volume is a major determinant of the local tumor control. 48,49,50,51 Potluri et al 50 analyzed 19 cases that were treated by postoperative radiotherapy and concluded that the postoperative residual volume was the most important determinant of outcome and that small residuals could be effectively controlled by fractionated radiotherapy.
However, extensive surgical resection of chordomas is not easy. Chordomas usually grow for extended periods without coming to medical attention and during this process widely invade the intricate anatomy of the central skull base. This initial tumor volume as well as the invasion pattern significantly impacts the surgical outcome and survival. The invasion significantly impacts surgical resectability and in selected cases such as those invading the cavernous sinus precludes a gross total resection. 52 Al-Mefty and Borba 4 classified chordomas into three categories according to their size and anatomical location to predict surgical resectability. Unfortunately, most chordomas present to clinical attention when they are considerably large. Most prominent clinical studies have reported gross total resection rates of 0 to 80%. 12,13,15,16,18,19,20,21,40,53 This large variability indicates that gross total surgical resection of chordomas cannot be achieved universally. Perhaps more importantly, the reported surgical success does not appear to be improving over time. Di Maio et al 11 compared the outcome of 56 patients treated between 1988 and 1999 and 39 patients from 2000 to 2011 and concluded that the gross total resection rates have not changed significantly (68% vs. 74%) but that only the complication rates have come down. Based on these findings, various intraoperative assistive technologies are utilized to achieve this aim of safely reducing the tumor volume to the absolute minimum possible.
23.3 Technical Challenges in Chordoma Resection
The greatest challenges in a chordoma surgery are anatomical localization of the tumor, localization of tumor remnants, and localization and protection of vital neurovascular structures. Intraoperative imaging technologies such as fluoroscopy, computed tomography (CT), and intraoperative MRI (ioMRI) are complementary to visualization technologies such as endoscopy and image guidance systems such as neuronavigation, and in combination these technologies increase both the safety and efficiency of chordoma operations. 17,54,55,56,57
Localization of the tumor within the bony clivus is the first challenge during chordoma surgery. In some cases, this is easily achieved using simple fluoroscopy, but in other cases neuronavigation and in extreme cases ioMRI can aid during drilling within the clivus. All of these technologies augment the safety of the operation, as slight deviations from the planned entry trajectory may lead the surgeon to close vicinity of vital neurovascular structures such as the petrous or cavernous carotid artery.
As described above, identification and localization of unexpected tumor remnants is also a challenging problem in chordoma surgery. The surgeon in most cases is limited by the visualization techniques (be it microscopy or endoscopy) and/or the reluctance to continue resection in proximity to vital neurovascular structures. An ioMRI update in many cases helps the surgeon to safely continue the resection. Similarly, endoscopy, neuronavigation, as well as the ioMRI all provide complementary information both to localize vital neurovascular structures as well as tumor remnants. The anatomical sites where unexpected tumor remnants are discovered are not random: some sites are recurrent. Most of these locations can be checked during the operation using endoscopic assistance and ioMRI. In their analysis of 50 skull base chordomas, Jahangiri et al 7 have shown that chordomas were less likely to occur in the lower third of the clivus but also that tumor remnants were significantly more likely to be located in the lower third of the clivus (33, 38, and 63% in upper, middle, and lower thirds of the clivus, respectively). Similarly, bony invasion of chordomas are difficult to detect in the upper third of the clivus behind the sella turcica. This small retrosellar area of the clivus is difficult to visualize with almost all anterior approaches but can be sought after by endoscopy or by ioMRI. Studies in invasive pituitary adenomas has clearly shown that ioMRI is highly reliable in identifying retrosellar tumor remnants. 54,58,59 Lateral extension of the tumor also becomes an important concern when those tumors are attacked using anterior approaches, as most anterior approaches are limited in their lateral extent. The use of the endoscope and ioMRI both help in identifying such lateral extensions. 60,61 Last but not least, the tumoral architecture of chordomas is an inherent factor that predisposes to residual tumor. In most cases, chordomas are relatively avascular tumors of soft consistency that are amenable to removal by suction. But it is also very common for chordomas to invade the surrounding bone in an irregular pattern. Unlike meningiomas that grow in a concentric fashion from a single epicenter, chordomas grow by infiltrating surrounding bone tissue in an irregular and unpredictable pattern. 28 This growth pattern results in a heterogenous tumor with occasional sequestrated bone islands within a soft tumor, which is commonly surrounded by healthy-looking bone in the periphery, which only separates another tumor island from the main bulk. Therefore, although wide bony drilling at tumor borders is recommended, it is also practical to use ioMRI to obtain negative borders. 4,17
Another major challenge in chordoma surgery is safe identification of vital neurovascular structures surrounding widely invasive skull base chordomas. Although they grow within the clivus, chordomas usually are located anterior and medial to vital neurovascular structures such as the carotid arteries, the basilar artery, and the cranial nerves. During their growth, large chordomas displace the basilar artery as well as the dura and the brainstem posteriorly and laterally. Due to this pathoanatomy, anterior midline approaches provide the most direct route to the tumor. Very frequently the dura is also eroded by the tumor. Therefore, during resection of the tumor, these vital anatomical structures (such as the basilar artery and the anterior brainstem) are located just behind the tumor, making their protection difficult. Both neuronavigation and ioMRI are very useful in demonstrating this proximity during the operation and facilitate the protection of these vital anatomical structures.
23.4 Neuronavigation
Neuronavigation systems are descendants of earlier efforts of stereotactic surgery. Earlier technologies such as the neuroarm have paved the way for more sophisticated systems of today. 62 In essence, these systems rely on registering a Cartesian coordinate system to neuroradiologic imaging, which may be acquired preoperatively or intraoperatively. Although these systems suffer significantly from brain shift during cerebral surgery, they are much more reliable in navigating the bony skull base and are ideal for use in chordoma surgery. 60,63,64,65,66 Surgical instruments, including the endoscope, can be registered to navigation systems. Navigation can be updated during surgery using ioMRI to reflect the most up-to-date situation, and the quality and spatial distortion of these images are nearly identical to preoperative MRI images. During the surgery, neuronavigation can either be tracked using computer screens in the operating room or the navigation information can be fed to operating-microscope units for frameless image-guided navigation systems. Such augmented reality imaging systems incorporate both the stereotactic information and imaging findings into the visualization units such as the operating microscope or the endoscope. 67 In their simple or the sophisticated forms, neuronavigation systems have become an essential part of chordoma surgery. 17,64,68,69
23.5 Intraoperative MRI
ioMRI provides an immediate intraoperative quality control in the resection of chordomas, giving information on the extent of resection and specific anatomical localization of possible tumor residuals in relation to the landmarks in the surgical approach. This is especially important for widely infiltrative tumors such as chordomas.
The main drive behind the concept of ioMRI technology was to increase the extent of resection in neuro-oncology, and two of the most common indications were low-grade gliomas and transsphenoidal surgery. The technology has proven feasible, practical, and useful for these indications. More recent studies also indicate that the impact of ioMRI significantly changes the disease course.
The first ioMRI unit was installed at Brigham and Women’s hospital in Boston in 1994. This initial scanner was developed by Professors Ferenc Jolesz and Peter Black of Harvard University in collaboration with General Electric Company and featured a double-donut design with 0.5 T magnet strength (SIGNA SP, General Electric Medical Systems, Milwaukee, WI). 70,71 This initial design had several unique characteristics, including a real-time neuronavigation system, which was not adopted in newer designs. 71 The main limitations of this system were the small operating space (as the operating field was located within the gantry) and the need for expensive nonferromagnetic instrumentation. 71 The first ioMRI-guided stereotactic biopsy was performed in June 1995 and the first ioMRI-guided tumor resection was performed in June 1996 at Brigham and Women’s Hospital in Boston. 71,72,73
Several other systems were quickly introduced worldwide, including the Erlangen 74 and Toronto Siemens low-field systems (Magnetom OPEN; Siemens Medical Systems, Erlangen, Germany) and the Hitachi 75 system (Fonar, Melville, NY; Hitachi Medical, Twinsburg, OH). After these initial systems had proven the feasibility and practicality of the ioMRI concept, a second generation of ioMRI systems were introduced, which featured higher magnet strengths, higher signal-to-noise ratios, improved image quality, and advanced imaging modalities such as diffusion-weighted imaging (DWI), diffusion tensor imaging (DTI), magnetic resonance spectroscopy (MRS), and functional MRI. Among all these improvements, the most significant was the improvement in T2-weighted image quality: Because intraoperative use of contrast materials poses technical problems, such high-resolution T2-weighted images are used by many centers as the standard ioMRI sequence. Such high-field systems were developed by several different companies and were installed at various academic and private institutions worldwide, including the Philips system at University of Minesota, 76 Siemens systems at University of Erlangen 77 and University of California, and the IMRIS system at Calgary, Canada. 78 Soon thereafter, scientific reports have documented the feasibility, practicality, and usefulness of the high-field systems. 78,79
An alternative approach to the MRI design was taken by others with an aim of decreasing the scanner size to fit a regular operating room. Magnet strength was reduced to as low as 0.12 T with permanent magnets of 40 cm diameter placed 25 cm apart from each other (Polestar N20 system; Medtronic Navigation, Louisville, CO). 80 After the 1.5-T high-field units, 3-T ultra-high-field systems were introduced to the operating room, which further increased the signal-to-noise ratio and the image quality. The first few examples of these systems included the 3-T Philips system at University of Minnesota (USA), 76 the 3-T Siemens system at Acibadem University (Turkey), 81 and the Philips system at Cliniques Universitaires St-Luc, Université Catholique de Louvain (Belgium). 82 3-T ioMRI systems have been successfully used in chordoma surgery, but no systematically analyzed case series has been reported yet. 81,83,84
Since the facility became operational in 2004, the Acibadem University 3-T ioMRI unit has been used seamlessly in chordoma surgery 81 ( ▶ Fig. 23.1, ▶ Fig. 23.2). This unit is designed using a twin-room concept where the patient is transferred to the MRI gantry during the procedure using a floating transfer table. The twin-room design enables the use of all standard ferromagnetic instrumentation and equipment such as the microscope, neuronavigation, endoscope, drill systems, Doppler, ultrasonography, and fluoroscopy, all of which are essential tools in chordoma surgery. MRI can be repeated as necessary, but in the majority of cases only one or two sessions are required. ioMRI provides information on the extent of surgery, possible unexpected tumor remnants, the location of vital neurovascular structures in relation to the resection cavity, and any possible complications during surgery such as hemorrhage and direct neural or neurovascular injury. Most cases of clival chordomas are treated by anterior approaches, and modified transsphenoidal approaches make up the majority of these case. Transsphenoidal surgery is an important indication for the use of ioMRI. Several centers have reported the usefulness of ioMRI for the surgery of invasive pituitary macroadenomas and craniopharyngiomas, and the documented experience is large. 54,58,59,73,81,84,85,86,87,88,89,90,91,92,93,94,95,96,97,98,99 High-field ioMRI systems are capable of clearly demonstrating unexpected tumor remnants and therefore positively impacting on transsphenoidal adenoma surgery.
Fig. 23.1 ioMRI is effective in identifying tumor remnants during surgery, which does lead to higher rates of resection during surgery. Demonstrated here is a midclival chordoma with significant brainstem compression in a 31-year-old male patient (a, b). The tumor was resected using an extended transsphenoidal approach. ioMRI documented near-complete resection (c). Follow-up MRI 7 years after the operation excluded and recurrences (d, e).
Fig. 23.2 A large clival chordoma that occupied the upper two thirds of the clivus and was compressing the brainstem was detected in a 70-year-old male patient (a, b, c). The tumor was resected using an extended transsphenoidal approach. ioMRI done during the procedure documented the resection of the tumor and alleviation of the mass effect on brainstem (d, e, f).
23.6 Neuroendoscopy
Endoscopy has become a very important part of the armamentarium for skull base surgery. Bringing both the point of vision and illumination to the immediate vicinity of the tumor and the possibility of changing the viewing angle by using angled optics greatly facilitate detailed visualization of the tumor within the intricate skull base anatomy, which in turn increases operative success. Endoscopy can be seamlessly combined with neuronavigation or microscopic techniques and become a part of the augmented reality environment to support skull base surgery. 17,54,55,56,57,65,100,101 The instrumentation and the technique have been significantly popularized.
23.7 Intraoperative Neuromonitoring
As discussed above, chordomas grow at the central skull base, frequently displacing important neurovascular structures such as the internal carotid arteries, cavernous sinus, basilar artery, cranial nerves, and brainstem. This is also the case in spinal and sacral chordomas where the tumor displaces the spinal cord, spinal roots, and their vasculature. This close proximity to vital neurovascular structures makes chordoma operations high-risk procedures with high surgical morbidity. 102,103 Ascending and descending pathways, cranial nerves and nuclei, respiratory and cardiovascular centers, and neural networks controlling basic functions such as swallowing, coughing, and eye movements are under risk during such skull base or spinal operations. Long tracts as well as motor roots and sphincter functions are under risk and must be protected during spine surgery. With recent developments in neurophysiology, various mapping and monitoring techniques have been established to improve surgical outcomes and reduce the risk of permanent postoperative neurologic deficits. 104,105,106 Intraoperative neuromonitoring (IONM) consists of two separate techniques: mapping techniques and monitoring techniques. The mapping techniques are used for the proper identification of specific neural structures (motor cortex, cranial nerves, nuclei, corticospinal tract) that may be distorted, displaced, infiltrated, and thereby hardly recognizable. 105,107 Mapping is a guide to the surgeon and helps to find safe entry zones, and continue the resection with a safe distance to important neural structures. On the other hand, monitoring techniques evaluate the “real-time” functional integrity of the nervous system. In order to minimize false-positive and more dangerously false-negative results during IONM, a patient-based, customized protocol should be created making use of both mapping and monitoring techniques.
Monitoring and mapping techniques will be discussed separately in this section. Methods available for IONM during skull base and spinal surgeries are listed in ▶ Table 23.1. The patient should be grounded during all procedures. During monitoring, the neurophysiologist may warn the surgeon of either decreasing responses or irritation of cranial/spinal nerves. In the case of a warning, precautions include irrigation with warm saline, application of vasoactive agents, continuing the dissection on another site, waiting, or stopping surgery, decisions depending on the severity of the alarm and the type of the surgery. The goal of IOMN is to assist the surgical team in order to have wide surgical margins while avoiding permanent neurologic deficits. Giving the surgical team more knowledge about the current anatomy and informing about the real-time functional changes in the neural system may lead to changes in the surgical strategies to avoid permanent neural damage.
Monitoring techniques | Mapping techniques |
Spontaneous electromyography (s-EMG)
| Triggered EMG (t-EMG, evoked EMG)
|
Brainstem auditory evoked potentials (BAEPs)
| Corticospinal tract localization |
Somatosensory evoked potentials (SEPs)
| |
Motor evoked potentials (MEPs)
| |
Corticobulbar motor evoked potentials (c-MEPs)
| |
Bulbocavernous reflex
|
For successful IONM, good cooperation between the neurophysiologist, surgeon, and anesthetist is required and a proper patient-based monitoring protocol should be applied to each patient, according to tumor localization, findings of preoperative neurologic examination, and preoperative neurophysiologic studies.
23.7.1 Mapping Techniques
Triggered Electromyography (t-EMG), Evoked Electromyography (e-EMG), Stimulated Electromyography
Stimulation-evoked responses may be useful for identification and therefore preservation of cranial/spinal nerves and nuclei. 106 General principles include electrical stimulation with a hand-held stimulation probe and recording a response from muscles that are innervated by the respective cranial nerves/roots with preferably needle electrodes. 105 The surgeon is guided to begin the surgery from a silent area or continue the resection safely. 105,108,109,110,111
For electrical stimulation, a bipolar or monopolar stimulation electrode may be selected. Bipolar stimulation provides more spatial selectivity and prevents unwanted current spread, whereas monopolar stimulation is preferred for scanning large areas. It is safe to have both available, with monopolar stimulation at the beginning of the operation during searching the surgical field and then switching to bipolar stimulation to find the exact localization of a nearby nerve. If proper stimulation settings (stimulus strength, duration) are used, a compound muscle action potential (CMAP) will be recorded when the nerve is located in the sphere around the tip of the electrode 105,109,112 ( ▶ Fig. 23.3).
Fig. 23.3 Facial nerve CMAP response recorded from orbicularis oculi and orbicularis oris muscles.
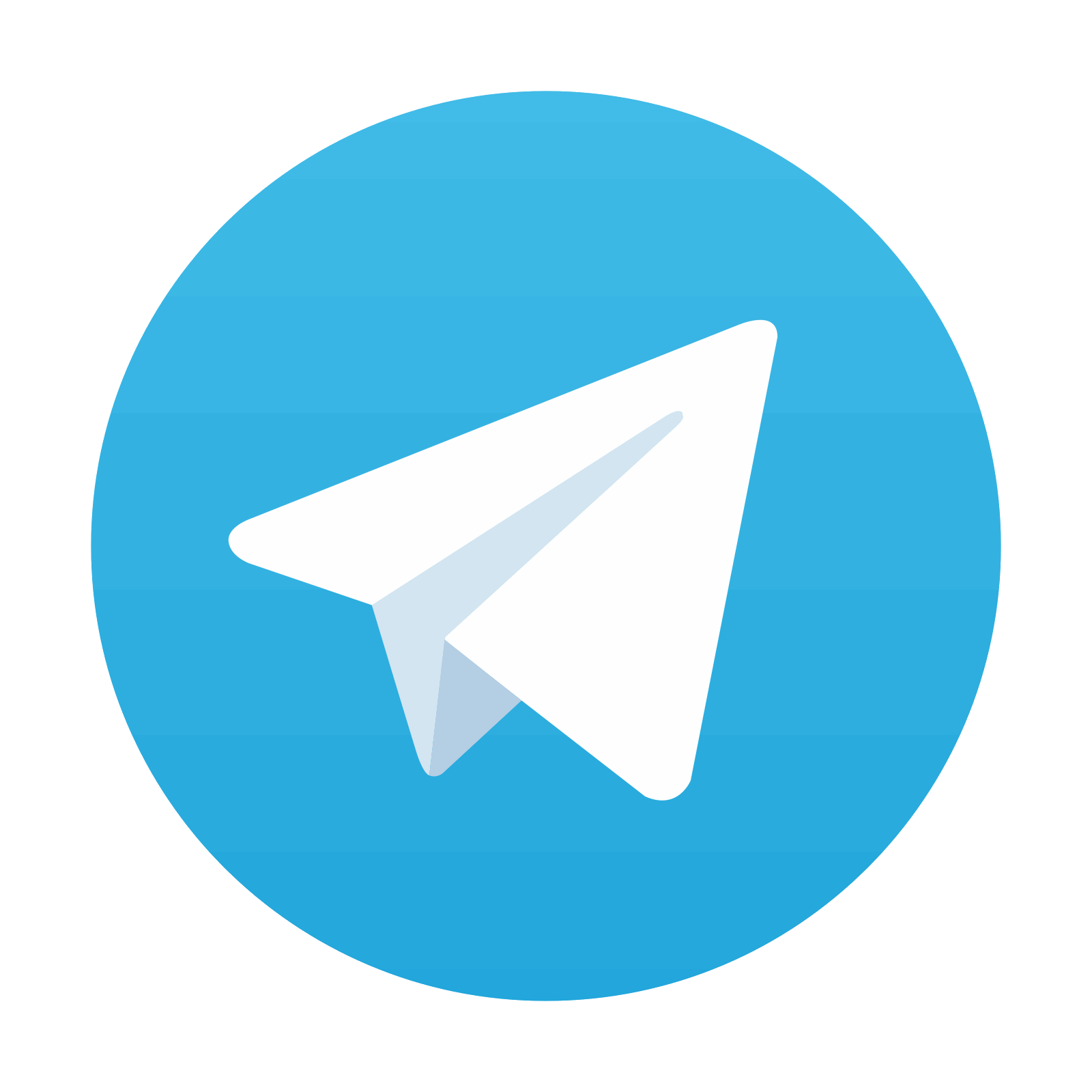
Stay updated, free articles. Join our Telegram channel

Full access? Get Clinical Tree
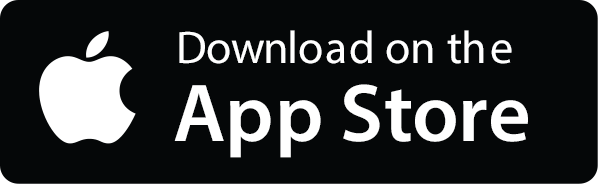
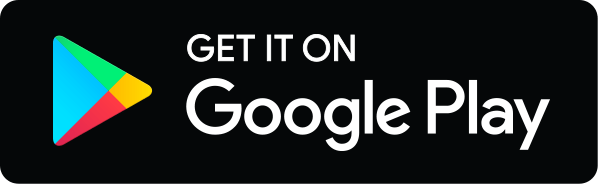