Alan David Kaye and Scott Francis Davis (eds.)Principles of Neurophysiological Assessment, Mapping, and Monitoring201410.1007/978-1-4614-8942-9_18
© Springer Science+Business Media New York 2014
18. Intraoperative Cortical Mapping: Basic Concepts, Indications, and Anesthesia Considerations
Art Saus1 , Charles Fox1 , Harish Bangalore Siddaiah1 , Alan David Kaye2, 3 , Scott Francis Davis4, 5 and Bharat Guthikonda6
(1)
Department of Anesthesiology, Louisiana State University Health Sciences Center Shreveport, Shreveport, LA, USA
(2)
Departments of Anesthesiology and Pharmacology, Louisiana State University Health Sciences Center, New Orleans, LA 70112, USA
(3)
Interim Louisiana State University, Hospital and Ochsner Kenner Hospital, New Orleans, LA, USA
(4)
Department of Anesthesiology, Louisiana State University Health Sciences Center, New Orleans, LA, USA
(5)
Department of Anesthesiology, Tulane University School of Medicine, New Orleans, LA, USA
(6)
Department of Anesthesiology, Louisiana State University Health, Shreveport, LA, USA
Abstract
The eloquent area of the brain is responsible for written and verbal communication. Functional neuroimaging indicates that interindividual variation exists with the anatomical location of the eloquent area of the brain. Some patients have shown significant contribution from areas located near, but outside of, the traditionally recognized eloquent area. Classically, these areas adjacent to or near the eloquent area were thought to have little impact on written or oral language skills, and many neurosurgeons, in the past, underestimated the impact of operating in these areas. Now, each patient is known to have a unique eloquent area. This necessitates intraoperative cortical mapping to more accurately identify functioning before removing brain tissues in patients undergoing epilepsy or brain tumor surgery in areas near this region of the brain. The goal of intraoperative cortical mapping is to maximize surgical resection in the eloquent area while minimizing the incidence of permanent disabilities. This chapter is intended to provide you with the concepts, indications, and anesthetic considerations important to intraoperative cortical mapping and to prepare you for further reading of more advanced texts and primary literature on this topic.
Introduction
The eloquent area of the brain is responsible for written and verbal communication. Functional neuroimaging indicates that interindividual variation exists with the anatomical location of the eloquent area of the brain. Some patients have shown significant contribution from areas located near, but outside of, the traditionally recognized eloquent area. Classically, these areas adjacent to or near the eloquent area were thought to have little impact on written or oral language skills, and many neurosurgeons, in the past, underestimated the impact of operating in these areas. Now, each patient is known to have a unique eloquent area. This necessitates intraoperative cortical mapping to more accurately identify functioning before removing brain tissues in patients undergoing epilepsy or brain tumor surgery in areas near this region of the brain. The goal of intraoperative cortical mapping is to maximize surgical resection in the eloquent area while minimizing the incidence of permanent disabilities. This chapter is intended to provide you with the concepts, indications, and anesthetic considerations important to intraoperative cortical mapping and to prepare you for further reading of more advanced texts and primary literature on this topic.
Concepts
Cortical Mapping: Historical Perspective
Dr. Robert Bartholow performed the first electrical stimulation of the cortex in 1874 on a patient named Mary Rafferty, a 30-year-old Irish woman who had been employed as a domestic servant. She presented with an infected scalp ulcer, which was diagnosed as cancerous. The physicians attempted to treat this surgically, leaving a 2-in. diameter hole in her skull with exposed dura. Apparently after determining that nothing could be done to save her life, Dr. Bartholow proceeded to experiment on the exposed brain, reportedly with the patient’s consent. By inserting needle electrodes into the exposed brain tissue and by applying small electrical currents to various areas, he noticed it caused movements in various parts of her body, and did not cause pain, following the patient’s initial complaints of neck pain with needle insertion. He also noted that application of larger currents resulted in seizure activity and what seems to be what we now recognize as a transient post-ictal state. Although it is reported that she returned to consciousness 20 min later, she complained of weakness and vertigo. As her condition worsened, her physicians did not do any further experiments, and she died a few days later. The conclusions from her autopsy were that although parts of her brain had been damaged from the electrodes, her death was due to her cancer and not to this experiment. Despite this “contribution to medical science,” both British and American physicians severely criticized Dr. Bartholow and his “reckless use of living human beings,” and the American Medical Association condemned his experiments, calling them “so in conflict with the spirit of our profession, and opposed to our feelings of humanity that we cannot allow’ them to pass unnoticed” [1].
Despite this criticism, and the fact that this hardly could be considered “cortical mapping” in any sense of our current use of the term, this provided to beginnings of understanding that electricity could be applied to different areas of the brain and that regional somatic activity would result. Research on electrical stimulation of human and animal brains continued, and in 1888 Dr. Nancrede mapped the motor cortex by the use of a battery-operated bipolar stimulator probe. Neurologists David Ferrier and Victor Horsley used cortical stimulation mapping techniques to research the function of the precentral gyrus and the postcentral gyrus in the late 1800s. In the early 1900s Charles Sherrington used monopolar stimulation to elicit responses and was able to determine that the precentral gyrus elicited a motor response and that the postcentral gyrus was a sensory cortex. Dr. Harvey Cushing confirmed these findings and was the individual primarily responsible for moving cortical mapping from an experiment into an accepted neurosurgical technique.
Prior to going into the techniques used in the process of cortical mapping and analysis of those determinations, we should first have some understanding of what a cortical map is. Most physicians and medical students have seen the diagram of the homunculus, as described in the 1930s by a Canadian neurosurgeon, Dr. Wilder Penfield, and probably remember an image of a human body displayed across a drawing of a human cortex, although in a somewhat disjointed manner, with a large elongated face in the lateral one-third, while the hand is in the next approximately one-third, and the rest of the body is in the next one-third, toward the most central part, then with the foot on the medial portion of each hemisphere (see Chap. 2). Most physicians will probably also remember that the sensory functions are shown as existing in the postcentral gyrus of the parietal lobe, just posterior to the central (or Rolandic) sulcus, while the motor function in the cortex is described as primarily in the precentral gyrus of the anterior lobe, just anterior to the central (Rolandic) sulcus (with a very similar homunculus image). In effect, this displays the most basic concept of a cortical map. Unfortunately, however, this homunculus diagram is a grossly inaccurate oversimplification. The anatomical view of the brain tissue does not always precisely correlate to localizing the functions as suggested by this diagram. Sensory and motor areas of the cortex can now be mapped much more precisely by electrical stimulation and recording of “evoked” responses.
Anatomic and Physiologic Basis
The cortex of the human brain is 2–4 mm thick and in most parts of the cerebrum contains six layers which can rather easily be demonstrated on microscopic examination. In general, sensory cortex is thinner and motor cortex is thicker [2]. Within the brain cortex, very small areas (minicolumns) can be identified that perform a specific information processing function. Minicolumns grow from progenitor cells within the embryo and contain neurons within multiple layers (2–6) of the cortex [3]. A cortical minicolumn is a vertical column through the cortical layers of the brain, comprising approximately 80–120 neurons, except in the primate primary visual cortex, where there are typically more than double this number. There are about 200,000,000 minicolumns in the human cortex. Many sources support the existence of minicolumns, especially Mountcastle [4] with strong evidence reviewed by Buxhoeveden and Casanova [5] who conclude “… the minicolumn must be considered a strong model for cortical organization” and that the minicolumn is “the most basic and consistent template by which the neocortex organizes its neurones, pathways, and intrinsic circuits.” It appears that this minicolumn structure is the primary means of organization in the cerebral cortex not only of humans but of other animals as well.
From multiple examinations and calculations, various researchers have estimated the diameter of a human minicolumn is about 28–60 μm. These minicolumns also contain downward projecting axons that are approximately 10 μm in diameter, with periodicity and density similar to those within the cortex, but not necessarily coincident. The probable estimated size of a minicolumn can also be calculated by area considerations: if the surface area of a human cortex (both hemispheres) is 1.27 × 1011 μm2 and if there are 2 × 108 minicolumns in the cortex, then the cortical surface area of each minicolumn is 635 μm2, giving an average diameter of 28 μm (but even if the cortex area were doubled to the commonly quoted value of 2.5 × 1011 μm2, this would rise to 40 μm). Johansson and Lansner do a similar calculation and arrive at an estimated minicolumn size of 36 μm.
There is also evidence from studies published in 2000 by two separate researchers, Buxhoeveden and Buldyrev, that spacing of 50–80 μm exists between adjacent columns. All cells in a single minicolumn have the same receptive field; adjacent minicolumns may have very different fields. Thus, a stimulus applied to a specific sensory nerve elicits a response within specific cortical minicolumns and does not necessarily elicit responses in immediately adjacent minicolumns in the cortex. This columnar arrangement forms the anatomic basis for the ability to perform cortical mapping. However, electrodes which are used for cortical mapping currently have a diameter of 2–3 mm, so we cannot electrically stimulate each discrete minicolumn but instead electrically stimulate a field containing hundreds of minicolumns with (hopefully) common functionality.
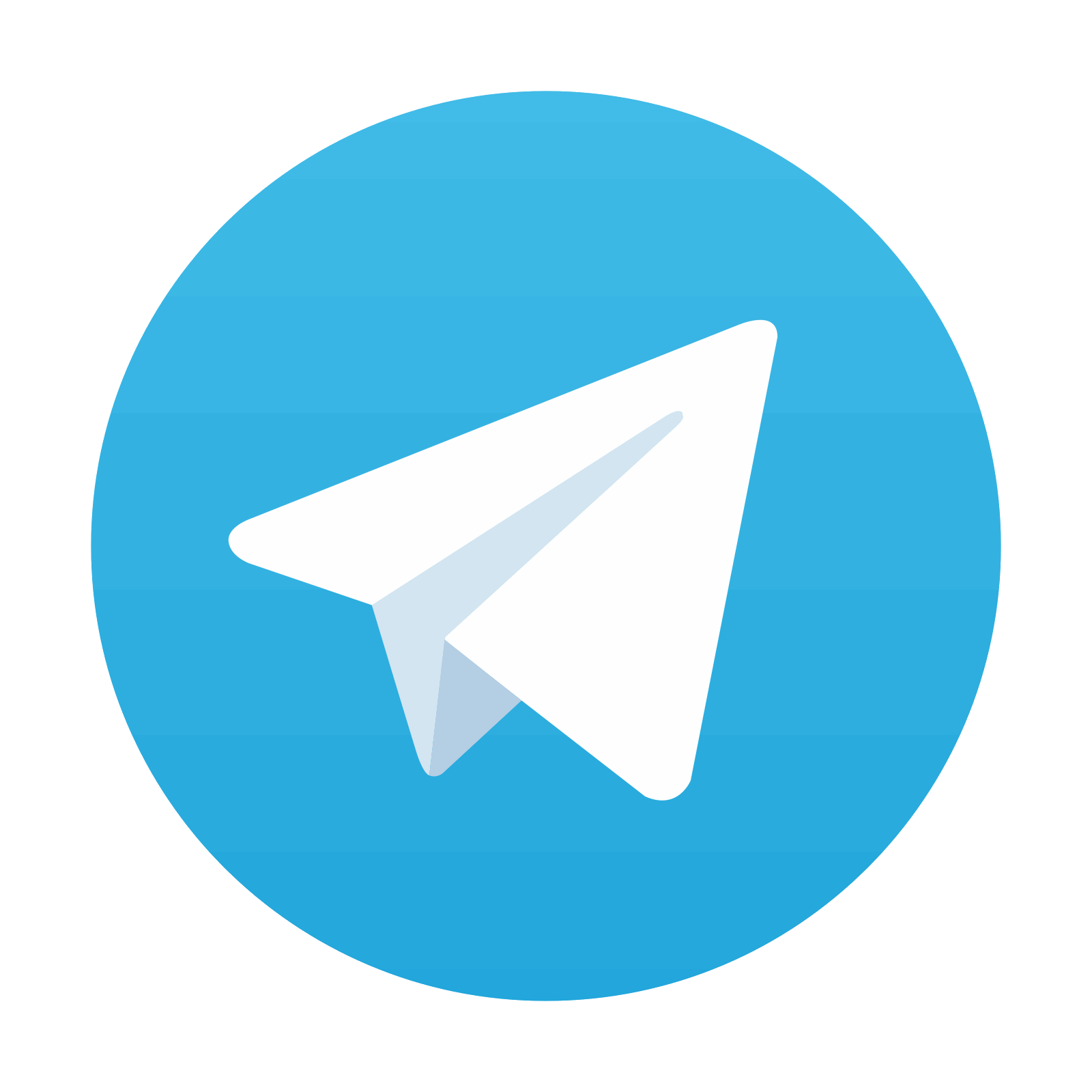
Stay updated, free articles. Join our Telegram channel

Full access? Get Clinical Tree
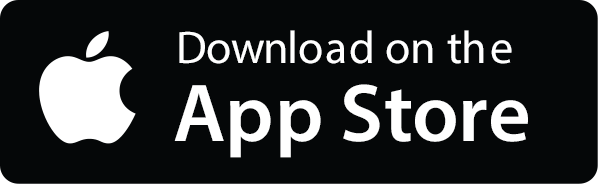
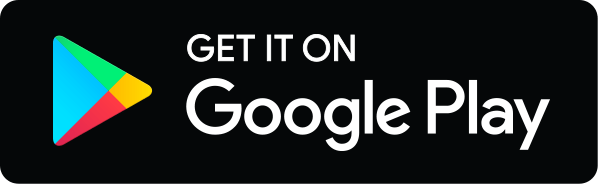