9 Intraoperative Imaging for Arteriovenous Malformations and Dural Arteriovenous Fistulas
Abstract
The routine use of intraoperative imaging during the surgical treatment of cerebral arteriovenous malformations (AVMs) and dural arteriovenous fistulas (dAVFs) may identify unsuspected residual arteriovenous shunting (AVS) and maximize the odds of complete treatment of the target lesion. The most commonly performing imaging modalities include (1) indocyanine green (ICG) videoangiography, (2) intraoperative digital subtraction angiography (DSA), (3) intraoperative ultrasound, (4) intraoperative flat panel rotational angiography, and (5) intraoperative magnetic resonance imaging (MRI). These different intraoperative imaging modalities are discussed in this chapter.
Keywords: intraoperative angiography, intraoperative magnetic resonance imaging, intraoperative computed tomography, intraoperative ICG, intraoperative ultrasound, arteriovenous malformation, dural arteriovenous fistula
Key Points
- Intraoperative imaging during surgical treatment of arteriovenous malformation (AVM) and dural arteriovenous fistula (dAVF) helps verify complete treatment of the lesion and reduces the subsequent risk of intracranial hemorrhage and additional surgery.
- Intraoperative digital subtraction angiography (DSA) offers the greatest sensitivity for residual arteriovenous shunting after surgical treatment and is the gold standard for intraoperative imaging during cerebrovascular surgery.
- Intraoperative indocyanine green (ICG) videoangiography, ultrasound, flat panel rotational angiography, and magnetic resonance imaging (MRI) may also be used during the surgical treatment of AVMs and dAVFs.
9.1 Introduction
9.1.1 Cerebral Vascular Lesions with Abnormal Arteriovenous Shunting
Cerebral Arteriovenous Malformations
Cerebral arteriovenous malformations (AVMs) are uncommon vascular lesions characterized by abnormal arteriovenous shunting (AVS) between cerebral arteries and veins. The angioarchitecture of AVMs is characterized by the absence of a normal capillary bed between cerebral arteries and veins and the presence of a nidus, which is a complex of channels connecting arteries and veins within the AVM.1,2 AVMs are thought to develop secondary to errors in vascular development and morphogenesis, and these lesions are typically identified in children or young adults.1,2 Due to the high risk of cerebral AVM rupture, significant effort is employed to characterize the AVM angioarchitecture, which in turn guides AVM treatment.3,4,5
The imaging evaluation of cerebral AVMs seeks to identify the arterial supply to the AVM, the size and location of the AVM nidus, the venous drainage of the AVM, the presence of a flow-related or nidal aneurysm, and the presence of a stenosis in the venous outflow of the AVM, all of which have treatment implications. Currently, digital subtraction angiography (DSA) remains the gold standard in the imaging evaluation of cerebral AVMs given its excellent spatial and temporal resolution with respect to these features. The identification of abnormal AVS is the most sensitive imaging feature for the presence of a cerebral AVM. AVS is therefore the most important clue as to the presence of an otherwise imaging-occult AVM, and the intraoperative imaging of AVMs seeks to identify AVS with a high sensitivity.
Computed tomography angiography (CTA) and magnetic resonance imaging (MRI) with magnetic resonance angiography (MRA) may also be used to evaluate cerebral AVMs. However, these studies lack the temporal resolution of DSA, which limits their sensitivity for AVS. Advanced MRI sequences, such as arterial spin labeling (ASL) and susceptibility-weighted imaging (SWI), may offer a high sensitivity for AVS, although these technique have not been deployed for intraoperative imaging to date.6,7 Nevertheless, CTA and MRI are important adjuncts in the surgical treatment of cerebral AVMs, as they demonstrate the relationship of the AVM to the brain anatomy and may be used for intraoperative navigation.
Dural Arteriovenous Fistulas
Dural arteriovenous fistulas (dAVFs) are characterized by abnormal AVS between arteries and veins within the dura and account for approximately 10% of intracranial vascular lesions.8,9,10 In contrast to AVMs, the AVS in dAVF most commonly arises from branches of the external carotid arteries and meningeal arteries. These arteries directly connect to the dural sinuses or cerebral veins without an intervening capillary bed, which results in a fistula with associated abnormal AVS.11 The pathogenesis of dAVFs is poorly understood, but these lesions are thought to be acquired following trauma or venous sinus thrombosis and are most commonly identified in adults older than 50 years.10,11 Presenting symptoms of dAVFs include intracranial hemorrhage, tinnitus, headache, or symptoms of increased intracranial pressure.10,11,12,13 Intracranial hemorrhage occurs in up to 18% of patients with dAVF, and the pattern of venous drainage is the most important predictor of hemorrhage risk.10,14
The imaging evaluation of dAVFs must identify the fistula location, the arteries supplying the fistula, the direction of venous drainage into a dural sinus, and retrograde filling of cortical veins. The identification of a direct connection to a cortical vein or reflux from a dural sinus into a cortical vein secondary to high pressure induced by the dAVF is critical because these features best predict the risk of dAVF rupture.10,14 Similar to cerebral AVMs, DSA is the gold standard in the imaging evaluation of dAVFs, given its high spatial and temporal resolution and superior ability to detect retrograde blood flow into a cortical vein.11,15
CTA and MRI with MRA are increasingly used for noninvasive imaging of dAVFs. These studies may demonstrate arterial prominence near the fistula site, engorgement of cortical veins, associated venous sinus thrombosis, and the sequela of dAVFs, including cerebral edema, hydrocephalus, flattening of the optic discs, or proptosis. In addition, there are emerging data regarding the utility of advanced MRI sequences, such as ASL and SWI, and time-resolved MRA and CTA in the diagnosis and characterization of dAVFs.5,6,7,16,17,18 Although these techniques are currently largely investigational, they are expected to play an increasing role in the imaging evaluation of dAVFs in the near future.
9.1.2 Surgical Treatment of Cerebral AVMs and dAVFs
Surgical Treatment of Cerebral AVMs: Intraoperative Imaging Principals
Surgical resection of cerebral AVMs is effective, offers the possibility of an immediate cure of the lesion, and has a low complication rate.19,20,21 For these reasons, surgical resection of cerebral AVMs is often considered the most optimal treatment when anatomically and technically feasible.19 The goal of surgical treatment of cerebral AVMs is complete resection of the AVM nidus with preservation of the normal arterial and venous anatomy and brain parenchyma.22 If the AVM nidus can be resected in its entirety, then the risk of AVM-related intracranial hemorrhage or AVM recurrence is very low. However, incomplete resection of a cerebral AVM does not reduce the subsequent risk of hemorrhage, and it may increase this risk.23
Intraoperative imaging may be performed during cerebral AVM resection using a variety of imaging modalities, such as indocyanine green (ICG) videoangiography, DSA, ultrasound, flat panel rotational angiography, MRI, or a combination of these modalities. The goals of intraoperative imaging are to determine the presence of any residual AVM nidus, to localize a suspected residual AVM nidus, and to assess for an operative complication. Intraoperative imaging may demonstrate unsuspected residual AVM nidus after resection in 10 to 20% of patients.24,25,26,27,28,29 Therefore, the routine use of imaging to identify residual AVM nidus may increase the likelihood of a complete resection in a single operative session and reduce the need for repeat procedures.
Intraoperative imaging during cerebral AVM resection must have a high sensitivity for residual AVS, which is the most sensitive feature for the presence of a residual nidus. Depending on the imaging modality used in the operating room, demonstration of a residual nidus may be challenging. The most commonly used intraoperative imaging modalities are described below.
Surgical Treatment of Cerebral dAVFs and Intraoperative Imaging Principals
Endovascular embolization is the most common treatment for dAVFs. However, a subset of dAVFs are not amenable to endovascular embolization because of difficult vascular access or arterial supply arising from an anatomically important artery that cannot be embolized safely.30,31,32 In such situations, surgical treatment of dAVFs may be performed.
In contrast to cerebral AVMs, dAVFs lack a compact vascular nidus and are composed of fistulas between arteries and a dural venous sinus or cortical vein. A dAVF may comprise a single fistula, but more commonly numerous small fistulas exist between multiple small arteries that supply the dAVF and the venous sinus or vein that drains the fistula. These anatomic differences make the surgical treatment of dAVFs different from that of AVMs. dAVFs may be treated by a combination of techniques, including surgical packing of the affected sinus, cauterization or surgical ligation of arteries feeding the dAVFs, skeletonization of the affected sinus, and cauterization or surgical ligation of cortical veins draining the dAVFs.33,34,35 More recently, surgical disconnection of the draining veins from the dAVFs has been shown to be an effective treatment for dAVFs with a low complication rate.36,37
Intraoperative imaging during surgical treatment of dAVFs is less well described than in the treatment of cerebral AVMs.24,38,39 Intraoperative imaging during dAVF treatment must identify residual AVS and retrograde filling of a cortical vein with a high sensitivity. Residual AVS and retrograde filling of a cortical vein indicate incomplete surgical treatment of the dAVF, which continues to place the patient at risk for intracranial hemorrhage. The imaging modalities used during intraoperative dAVF imaging are described in detail in the following section.39,40,41,42,43
9.2 Materials and Methods
PubMed searches were performed for intraoperative imaging, cerebral arteriovenous malformation, dural arteriovenous fistula, magnetic resonance imaging, computed tomography, digital subtraction angiography, indocyanine green, and ultrasound. Pertinent articles were reviewed. Additional articles were identified from the reviewed articles in some circumstances.
9.3 Results
9.3.1 Intraoperative Imaging Techniques
The most commonly used intraoperative imaging modalities during surgical treatment of cerebral AVMs and dAVFs include ICG videoangiography, DSA, ultrasound, flat panel rotational angiography, and MRI. Intraoperative MRI and flat panel rotational angiography have been described during cerebral AVM resection, but these techniques have not yet been reported during dAVF surgery, to our knowledge. In this section, we describe these imaging modalities during surgical treatment of cerebral AVMs and dAVFs.
Intraoperative Indocyanine Green
ICG is a cyanine dye that binds with a high affinity to bloodborne proteins, and it may therefore be used as a blood pool imaging agent. ICG is administered by intravenous injection and imaged within cerebral blood vessels with a high temporal and spatial resolution using near-infrared spectroscopy that is integrated with the operative microscope. Early identification of ICG fluorescence within a vein draining an AVM or dAVF indicates AVS, and the neurosurgeon may use this information to guide AVM (► Fig. 9.1) or dAVF (► Fig. 9.2) resection. For these reasons, ICG videoangiography has become a common tool in cerebrovascular surgery.
Fig. 9.1 ICG videoangiography during ruptured AVM resection. A young patient presented with intraparenchymal hemorrhage (a) and was taken to the operating room for hematoma evacuation. Intraoperative ICG angiography demonstrates an AVM nidus (arrows) in the frontal opercula in the early (b) and late (c) arterial phase (b). An early draining vein (arrowhead) is present in the early parenchymal phase (d). (These images are provided courtesy of Johnny Wong, MD, PhD, Sydney, Australia.)
Fig. 9.2 ICG videoangiography during dAVF ligation. Intraoperative ICG angiography during treatment of a superior petrosal sinus dAVF demonstrates engorged cortical veins (a, arrow and arrowhead) with associated venous reflux in the early arterial phase following ICG injection (b, arrow and arrowhead). (These images are provided courtesy of Johnny Wong, MD, PhD, Sydney, Australia.)
ICG videoangiography has several distinct advantages during AVM and dAVF surgery. It is easy to use multiple times during a surgery due to its short half-life of 3 to 4minutes, and it provides real-time identification of AVS within the cerebral vasculature.44 ICG videoangiography also minimizes interruptions during the surgery by allowing the surgical microscope to be maintained in position with continuous visualization of the operative bed. In most instances, ICG videoangiography may be performed in 5 minutes or fewer, which may translate into a significant cost savings with respect to procedural times compared to other intraoperative imaging studies.40 However, these advantages of ICG videoangiography must be balanced with several limitations of the technique.
The field of view during ICG videoangiography is confined to what may be seen through the surgical microscope (see ► Fig. 9.1 and ► Fig. 9.2), which may therefore limit the detection of AVS outside of the operative field. The limited visualization of ICG is exacerbated when the neurosurgeon is working deeply within the brain or in an anatomically confined operative bed.45 For these reasons, ICG videoangiography may fail to identify residual AVS during AVM resection in up to 20 to 75% of procedures.29,45 However, other studies have demonstrated a more favorable sensitivity of ICG videoangiography with false-negative rate of 9% or less during surgical treatment of cerebral AVMs and dAVFs.40,42
Although intraoperative ICG videoangiography has been more widely used during cerebral AVM resection, there are relatively few papers describing this technique during the surgical treatment of dAVFs (► Fig. 9.2).40,43 However, the largest series describing the use of ICG videoangiography during surgical treatment of dAVFs in 47 procedures noted a 8.7% false-negative rate, which is similar to the reported false-negative rate of intraoperative DSA.40 To date, there has not been a prospectively designed study to compare intraoperative ICG videoangiography and intraoperative DSA, but such a study would be of interest to undertake.
Intraoperative Digital Subtraction Angiography
Intraoperative angiography was first described in the 1960s, and today intraoperative DSA remains the gold standard for intraoperative cerebral vascular imaging.46,47 Intraoperative DSA offers excellent temporal and spatial resolution, which allows for sensitive detection of AVS, reflux into small cortical veins, and the presence of a small residual nidus after partial AVM or dAVF resection.24,25,26,27,28,39,48 Furthermore, intraoperative DSA performed during parenchymal hematoma after AVM rupture may identify an occult cause of hemorrhage, such as a nidal or perinidal aneurysm that was previously compressed by the hematoma.49
Intraoperative DSA may be performed in a standard operating suite with a portable C-arm fluoroscopic unit or in a hybrid endovascular-surgical suite with biplane angiography equipment. Typically, the patient is placed under general anesthesia, and then arterial access in the common femoral artery, radial artery, or brachial artery is obtained with or without ultrasound guidance. The sheath is then secured to the patient with a suture and connected to a continuous heparinized saline flush to present thrombus forming within the sheath or near the sheath tip. The patient is then placed in position for the surgery and placed in a radiolucent Mayfield skull clamp to facilitate subsequent DSA.
Intraoperative DSA may be performed before the surgical procedure if localization of the cerebral AVM or dAVF is required. More typically, however, the location of the AVM or dAVF has been determined based on preoperative conventional angiography, MRI, or CTA, as this information is useful in determining patient positioning in the operative suite. Furthermore, a preoperative high-quality DSA is important to understand completely the vascular anatomy of the lesion being treated. After the neurosurgeon has completed what he or she feels is a complete resection of the AVM (► Fig. 9.3) or obliteration of the dAVF (► Fig. 9.4), intraoperative DSA may be performed to exclude unsuspected AVS. The operative microscope is removed, and a portable C-arm fluoroscopic unit or a biplane fluoroscopy unit is brought into position. The neurointerventionalist then introduces a catheter through the sheath and selects the arteries known to supply the AVM or dAVF using fluoroscopic guidance. A DSA is then performed following contrast injection through the catheter. Multiple cervical vessels may be selected to ensure complete interrogation of all vessels previously shown to supply the AVM or dAVF based on the information from the preoperative DSA. The images are carefully reviewed by the neurointerventionalist and the neurosurgeon to assess for any remaining AVS with a draining vein or dural sinus or evidence of a residual nidus.
Fig. 9.3 Digital subtraction angiography during AVM resection. Preoperative angiography of a patient with a right occipital lobe AVM demonstrates arterial supply from the right middle cerebral artery (arrow, a) and the right posterior cerebral artery (arrow, d) with superficial venous drainage. Intraoperative (b,e) and postoperative (c,f) angiography demonstrates no residual AVM nidus or early draining vein (arrowheads, b,c,e,f) after injection of the right internal carotid artery (b,c) and the right vertebral artery (e,f), consistent with successful resection.
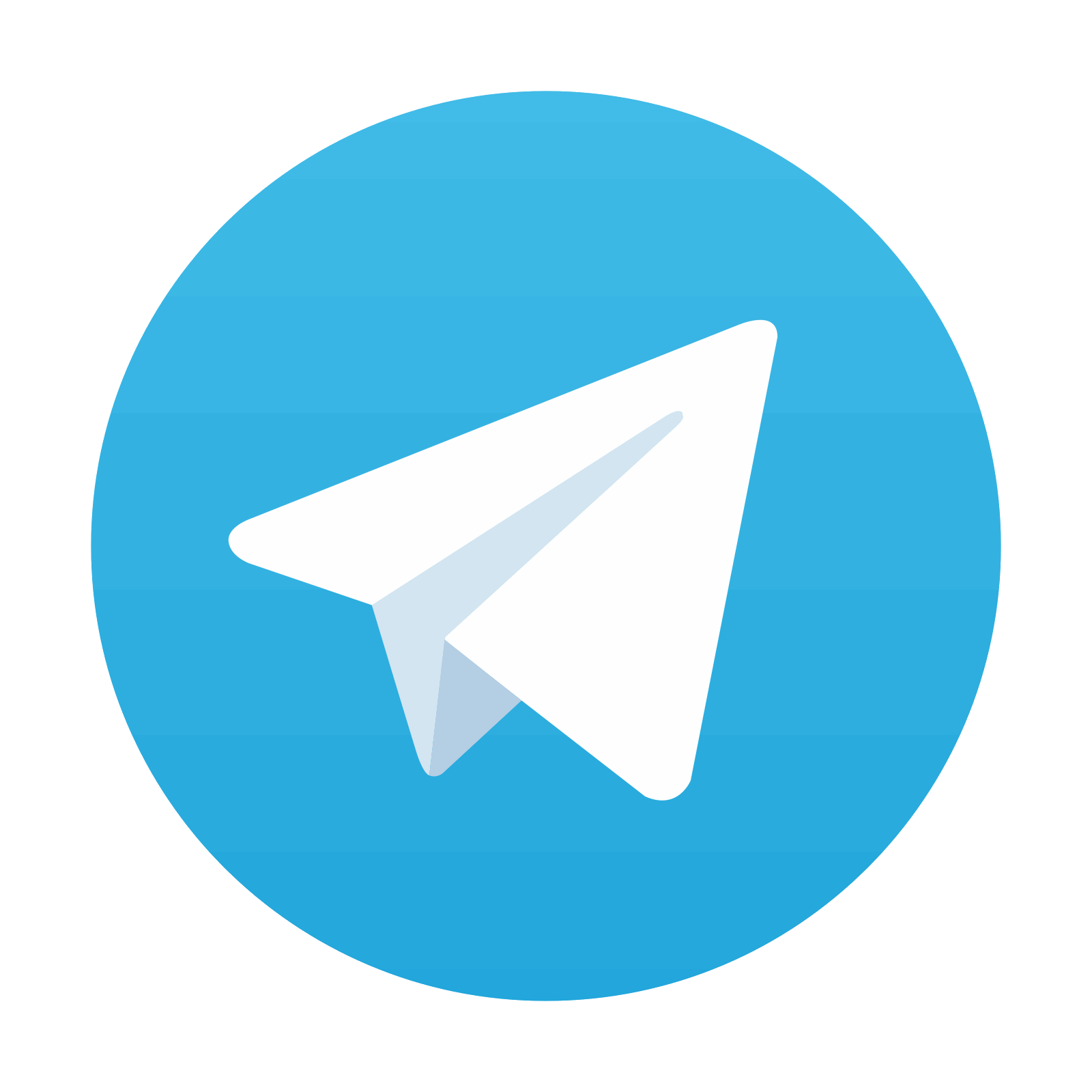
Stay updated, free articles. Join our Telegram channel

Full access? Get Clinical Tree
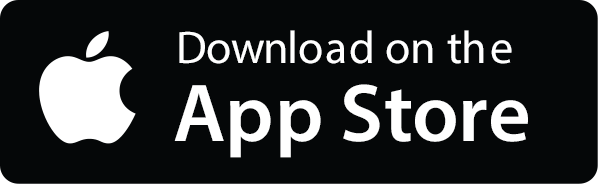
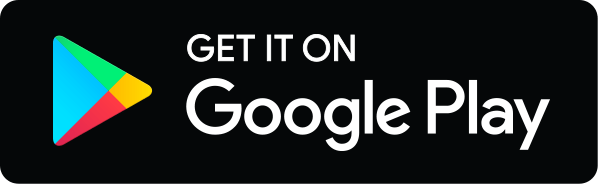