Neurovascular surgery is a broad and challenging, yet exciting field within neurologic surgery. The neurovascular surgeon must be meticulous; because the brain and spinal cord are unforgiving to ischemic insults. Along with the pressures of this demanding subspecialty comes the potential to help patients recover from potentially devastating pathology to go on and lead normal, healthy lives. Several intraoperative imaging modalities are available to help maximize treatment success while reducing risk. This article reviews each of these modalities, including digital subtraction angiography, fluorescence angiography, Doppler ultrasonography, laser Doppler, laser speckle contrast imaging, neuronavigation, and neuroendoscopy.
Key points
- •
Intraoperative digital subtraction angiography is typically selected to assess for complete resection of arteriovenous malformations and obliteration of dural arteriovenous fistulas.
- •
Intraoperative indocyanine green dye video angiography frequently provides the greatest benefit in assessing vessel patency after aneurysm clipping, assessing bypass graft patency, and identifying/obliterating dural arteriovenous fistulas.
- •
The selection of adjunctive imaging modalities is performed on a case-by-case basis because no one modality is superior in every regard.
- •
New technologies are in development and the neurovascular surgeon needs to stay abreast of these technologies to provide optimal patient care.
Introduction
Ischemic events during neurovascular surgery are a frequent cause of new postoperative neurologic deficits. Therefore, maintenance of central nervous system tissue perfusion is of utmost importance. Intraoperative imaging techniques can reduce the risk of ischemic events, as well as the need for additional surgery or intervention in the setting of incompletely treated pathology. Several intraoperative imaging modalities exist; however, no one approach is superior in every aspect. The careful selection and use of intraoperative imaging techniques is associated with improved outcomes. Therefore, the neurovascular surgeon must be familiar with, and understand the strengths and weaknesses of each of the imaging modalities. Although the operative microscope helps to visually inspect vessels from the outside, vessel patency and residual aneurysm filling cannot be discerned from visual inspection alone. Herein we review several modalities for adjunctive intraoperative imaging in open neurovascular surgery.
Introduction
Ischemic events during neurovascular surgery are a frequent cause of new postoperative neurologic deficits. Therefore, maintenance of central nervous system tissue perfusion is of utmost importance. Intraoperative imaging techniques can reduce the risk of ischemic events, as well as the need for additional surgery or intervention in the setting of incompletely treated pathology. Several intraoperative imaging modalities exist; however, no one approach is superior in every aspect. The careful selection and use of intraoperative imaging techniques is associated with improved outcomes. Therefore, the neurovascular surgeon must be familiar with, and understand the strengths and weaknesses of each of the imaging modalities. Although the operative microscope helps to visually inspect vessels from the outside, vessel patency and residual aneurysm filling cannot be discerned from visual inspection alone. Herein we review several modalities for adjunctive intraoperative imaging in open neurovascular surgery.
Digital subtraction angiography
Intraoperative digital subtraction angiography (DSA) was first introduced in 1974. It was further developed for routine use in the 1990s. DSA has become the gold standard for assessing intracranial vasculature. It allows for the visualization of vessels when there are calcifications, atherosclerotic plaques with thick walls, partially or completely thrombosed aneurysms, or giant aneurysms. Intraoperative DSA has proven useful in the assessment of aneurysm, extracranial–intracranial (EC–IC) and intracranial–intracranial (IC–IC) bypass, arteriovenous malformation (AVM), and dural arteriovenous fistula (DAVF).
Aneurysm Surgery
The objective in aneurysm clip ligation is to occlude the aneurysm neck while maintaining patency of parent, branching, and perforating vessels. Postoperative DSA has shown approximately a 2% to 8% incidence of residual aneurysm filling, along with a 4% to 12% incidence of occlusion of parent or branching vessel, depending on the difficulty of the aneurysm. A residual aneurysm neck, if left untreated, may result in subsequent aneurysm growth and rupture. Thus, this often necessitates an additional surgery or procedure to prevent deleterious consequences. Occlusion of a parent or branching vessel typically results in stroke by the time the postoperative DSA is completed.
Intraoperative DSA first gained popularity for use in correcting imperfectly positioned aneurysm clips with improvement in surgical outcomes. The rate of aneurysm clip readjustment after intraoperative DSA ranges from 8% to 34%. Intraoperative DSA is typically reserved for the treatment of complex aneurysms; however, the preoperative angiogram may not be a good predictor of need for intraoperative DSA. DSA along with 3-dimensional rotational angiography allows for even a small aneurysm neck remnant to be visualized. Intraoperative DSA may also assist in locating the correct vessel for sacrifice when an aneurysm is located on a distal segment of a small-caliber vessel ( Fig. 1 ). Intraoperative DSA may demonstrate vasospasm occurring from vessel manipulation, which can be treated immediately. Intraoperative DSA has limitations in aneurysm surgery in that it does not provide information about the integrity of perforating vessels. In addition, even with an experienced team, intraoperative DSA still typically requires a minimum of 20 minutes to perform. Many neurosurgeons use a time limit of 8 to 10 minutes before considering an ischemic event to be irreversible. The delay between clip placement and clip readjustment is often too great to avoid ischemic complications. Batjer and colleagues reported that clip readjustment for occluded vessels after intraoperative DSA was associated with a 33% stroke rate.
Extracranial–Intracranial and Intracranial–Intracranial Bypass
EC–IC and IC–IC bypass have proven useful in treating complex intracranial aneurysms, moyamoya, and atherosclerosis. EC–IC bypass is well-suited for augmenting blood flow, whereas IC–IC bypass is often used in the treatment of complex and/or giant aneurysms not amenable to straightforward clipping or endovascular management. In patients suffering hemodynamic insufficiency secondary to internal carotid stenosis or occlusion, or middle cerebral artery stenosis, the goal is to augment cerebral blood flow (CBF) and cerebrovascular reserve capacity to prevent future ischemic events. In aneurysm treatment, bypass allows for flow reduction through the diseased segment or trapping of the diseased vessel segment and removing it from the circulation. The postoperative bypass patency rate is reported to be between 90% and 96%. Hence, a primary goal in cerebral bypass is to avoid graft occlusion or stenosis at the anastomosis site(s), which can lead to downstream ischemia. Intraoperative and postoperative DSA remains the gold standard for assessing bypass graft patency. It allows for a dynamic bypass assessment, providing information about the extent of intracranial perfusion through the bypass. However, indocyanine green dye video angiography (ICG-VA) has largely replaced intraoperative DSA for assessment of bypass graft patency.
Arteriovenous Malformation Resection
In AVM surgery, the objective is to completely resect the nidus. Hemorrhage, however, may compress the AVM, making portions of the nidus not visible on preoperative imaging. Partial resection of the AVM nidus does not seem to improve on the natural history risk of rupture, and may in fact increase the risk of rupture, likely secondary to venous hypertension. DSA remains the gold standard in evaluating a patient with an AVM. There is no standard protocol for assessing the achievement of resection with intraoperative or postoperative DSA. Postoperative DSA revealing residual nidus or early draining veins requires reoperation for further resection, which can frequently be performed with satisfactory results. Intraoperative DSA has proven useful for identifying small AVMs, and locating and excising unexpected residual AVM, thus avoiding need for a second operation. Unfortunately, intraoperative DSA is often of lesser quality, which can lead to more false-positive and false negative results when assessing for residual AVM. False-negative results may be secondary to compression of the residual nidus by hematoma or cerebral edema, transient spasm, or thrombosis of feeding arteries, or technical factors. False positives may result from hyperperfusion in normal vessels surrounding the resection cavity; therefore, residual nidus should only be diagnosed when there is associated arteriovenous shunting. As a result, some surgeons prefer to perform an immediate postoperative DSA, as an alternative to, or in addition to, intraoperative DSA.
Dural Arteriovenous Fistula Obliteration
Most intracranial DAVFs are treated using endovascular techniques using a transarterial, a transvenous, or a combined approach; however, this is not always feasible. In DAVF surgery, the aim is to completely resect and/or obliterate the fistula. One case series reported that intraoperative DSA identified unexpected residual DAVF in 18.8% of cases. Furthermore, intraoperative DSA is instrumental in identifying the fistula, and allows assessment of obliteration.
Spinal DAVFs are frequently treated with an open surgical approach. The spinal cord often lacks adequate collateral arterial supply, dramatically increasing the risk of neurologic injury associated with endovascular treatment approaches. Intraoperative DSA has proven to be an asset in locating and obliterating spinal DAVFs.
Additional Limitations of Digital Subtraction Angiography
Intraoperative DSA increases radiation exposure for the patient and the entire operative team. It also carries with it a complication rate of 1% to 3%, often in the form of thromboembolic events, arterial dissection, and/or groin hematoma. Many institutions do not have a combined or hybrid operative suite, increasing the difficulty of obtaining high-quality intraoperative DSA. Intraoperative DSA obtained using a C-arm fluoroscope does not carry the same image quality as those images obtained in a dedicated biplane cerebral angiography or hybrid suite. Furthermore, a hybrid operative suite requires a highly specialized team, increasing resources and cost. Because of these downsides to intraoperative DSA, many surgeons do not use this adjunctive imaging modality routinely and reserve it for select cases.
Indocyanine green dye video angiography
ICG is currently the most frequently used adjunct imaging technique in open neurovascular surgery. ICG was approved by the US Food and Drug Administration in 1956 to assess cardiocirculatory and liver function, and in 1975 for ophthalmic angiography. In 1995, ICG was first used to study cerebral hemodynamics. Eight years later, in 2003, intraoperative microscope-based ICG-VA was first described for use in intracranial aneurysm clipping.
ICG is a water-soluble, near-infrared fluorescent tricarbocyanine dye. ICG binds to plasma globulins in about 1 to 2 seconds after intravenous injection. It is metabolized almost entirely by the hepatic parenchymal cells and is secreted into bile. It has minimal uptake in other organs and in peripheral tissues. Caution must be exercised when considering use in patients with history of iodide allergy because the dye preparation may contain up to 5% of sodium iodide. It is typically well-tolerated and has an estimated adverse reaction of 0.05% to 0.20%. It is a pregnancy category C drug; therefore, its use in pregnant women should only be considered if the benefits clearly outweigh the risks.
The recommended ICG dose for videoangiography is 0.2 to 0.5 mg/kg. Many institutions use a standard bolus of 25 mg dissolved in 2 to 5 mL of normal saline. Several doses can be given throughout an operation. The half-life is 2.5 to 3.0 minutes; therefore, waiting 5 to 10 minutes before redosing is recommended. The daily dose should not exceed 5 mg/kg.
ICG-VA gained widespread popularity among neurovascular surgeons owing to its ease of use, with the setup time taking 1 to 3 minutes, and an interpretation time of 30 to 40 seconds. As opposed to intraoperative DSA, ICG-VA does not take an experienced team to perform. Additionally, ICG-VA provides high spatial resolution. The uses for ICG-VA are broad and include aneurysm surgery, EC–IC bypass, IC–IC bypass, cerebral and spinal AVM, intracranial and spinal DAVF, extracranial vertebral artery surgery, carotid endarterectomy, and cortical perfusion assessment.
Aneurysm Surgery
ICG-VA is used routinely in aneurysm surgery, and allows for evaluation of flow in large vessels, perforating vessels, as well as the aneurysm. ICG-VA can be used to visualize small vessels (<0.5 mm) not visible on DSA. Raabe and colleagues reported that ICG-VA corresponded with intraoperative or postoperative DSA in 90% of aneurysm clipping cases. Others reported a correspondence as high as 97%. Compared with intraoperative or postoperative DSA, ICG-VA decreases time between clip placement and clip repositioning, reducing the probability of ischemia. The use of ICG-VA in aneurysm clipping is not without limitations. The view is restricted to that provided by the operative microscope, and image quality is reduced when working in deep surgical fields. In addition, vessels covered by clot, aneurysm, brain tissue, or other vessels cannot be visualized. Similarly, vessel wall calcification or intraluminal thrombus may obscure the view. In a series where ICG-VA was performed routinely after aneurysm clipping, Dashti and colleagues reported an unexpected aneurysm residual neck rate of approximately 6%, and 64% of these were deep aneurysms. In this series and others, ICG-VA missed slow residual aneurysm filling. Raabe and colleagues found that ICG-VA missed hemodynamically irrelevant stenosis in 7.3% of cases and hemodynamically relevant stenosis in 0.8% of cases; in these cases, the stenosis was revealed on DSA. Multiple injections of ICG with short intervals between may cause retrograde filling of vessels distal to the aneurysm, misleading the surgeon into thinking a parent or branching vessel is patent. To minimize this risk, care must be taken to expose all vasculature in close proximity to the aneurysm before assessment with ICG-VA. Also, the ICG-VA can be replayed to assess for retrograde flow of ICG. ICG-VA allows for assessment of collateral CBF before sacrifice of distal cerebral arteries required in treatment of complex distal aneurysms.
Extracranial–Intracranial and Intracranial–Intracranial Bypass
In cerebral bypass surgery, ICG-VA is used routinely to assess the patency of the bypass ( Figs. 2 and 3 ). ICG-VA results have shown to correlate well with postoperative DSA and computed tomography angiography. In addition, ICG-VA has not only proven useful in revealing occlusion of the graft, but also stenosis at the anastomotic site, thus improving the patency rate. In a large series, Woitzik and colleagues reported an initial bypass patency rate of 87% when evaluated with ICG-VA. After subsequent revision, they reported an early postoperative bypass patency rate of 100%.
Arteriovenous Malformation Resection
In AVMs with superficial venous drainage, ICG-VA is effective in revealing residual arteriovenous shunting. ICG-VA has consistently proven valuable in the identification of feeding and nidal arteries. In a series of 32 patients undergoing resection of intracranial AVMs, Bilbao and colleagues reported that ICG-VA helped to identify AVM vessels in 26 patients. However, ICG-VA missed 3 of 4 unexpected residual AVMs, and intraoperative DSA missed 1 of 4 unexpected residual AVMs. In this series and others, ICG-VA proved less valuable in deep and high-grade AVMs.
The goals in resection of intramedullary spinal AVMs are different from cerebral AVMs. In resection of spinal AVMs, often the pia plane is preserved, and the surgeon carefully coagulates the feeding arteries and draining veins along the surface, devascularizing the AVM while leaving the intramedullary nidus behind. ICG-VA has proven itself as an advantageous adjunct in resection of spinal AVMs, and it is typically used several times during the operation to identify feeding arteries and draining veins.
Although ICG-VA is a beneficial adjunctive imaging modality for use in resection of AVMs, secondary to its limitations it does not replace the need for intraoperative and/or postoperative DSA.
Dural Arteriovenous Fistula Obliteration
In both intracranial and spinal DAVF surgery, ICG-VA allows for the identification of the anomalous arteriovenous connection allowing for obliteration. In a retrospective review of intracranial DAVFs, Thind and colleagues reported that ICG-VA identified the fistulous point in 96% of cases; it also demonstrated satisfactory obliteration of the fistulas, with comparable results to intraoperative and postoperative DSA in 91% of cases. In this series, the false-negative rate of 8.7% associated with ICG-VA was similar to the false-negative rate of 10.5% associated with intraoperative DSA.
In spinal DAVF surgery, the use of ICG-VA has been associated with excellent outcomes. In addition, ICG-VA is integral in identification and treatment of angiographically occult spinal DAVFs. Some argue that it may negate the need for routine postoperative DSA.
Dual-Image Videoangiography
Standard ICG-VA shows vessels as white on a black background; other tissue cannot be visualized. Dual image videoangiography was developed in 2015 to allow for simultaneous visualization of both the microscope image (within the visual light spectrum) as well as the near-infrared fluorescence images from the ICG-VA. This technology allows for a better understanding of the vascular anatomy observed on ICG-VA with relation to surrounding anatomic structures. It has already proven useful in aneurysm clip ligation, cerebral bypass, and AVM and DAVF resection at our institution and others.
FLOW 800
One of the limitations to conventional ICG-VA is that a precise quantitative analysis of CBF cannot yet be determined. To date, no imaging modality exists that can provide routine intraoperative quantitative assessment of CBF with high temporospatial resolution. The FLOW 800 system (Zeiss, Oberkochen, Germany) was introduced recently as a microscope-integrated tool that provides a color-coded qualitative assessment of perfusion in preselected regions of interest after injection of ICG. Qualitative analysis is provided by the measurement of relative changes in fluorescence intensity. The software has the capability to measure several parameters, including maximum intensity, time to peak intensity, time to half-maximal fluorescence, rise time, blood flow index, and microvascular transit time. Prinz and colleagues studied the use of FLOW 800 for EC–IC bypass. Although the technology did provide a visual qualitative assessment CBF, these investigators found that it lacked correlation with quantitative Doppler flow and semiquantitative laser speckle contrast imaging (LSCI). In carotid endarterectomy, FLOW 800 has been used to help identify the region of the arterial plaque. In AVM resection, the system was used to help distinguish between normal en passage arteries and veins, and nidus vessels and draining veins. However, the FLOW 800 system does not seem to change the surgical strategy or outcomes associated with AVM surgery. Because the FLOW 800 does not calculate vessel diameter, true blood flow rate calculations are not possible. The data obtained depend on the ICG injection rate and dose, and systemic hemodynamic parameters; therefore, these variables must be standardized. Differences in blood volume, cardiac output, and angioarchitecture may prevent future establishment of interindividual comparison of data. In addition, the observable territory is restricted to the view provided by the microscope. The role of this technology in neurovascular surgery is still being established.
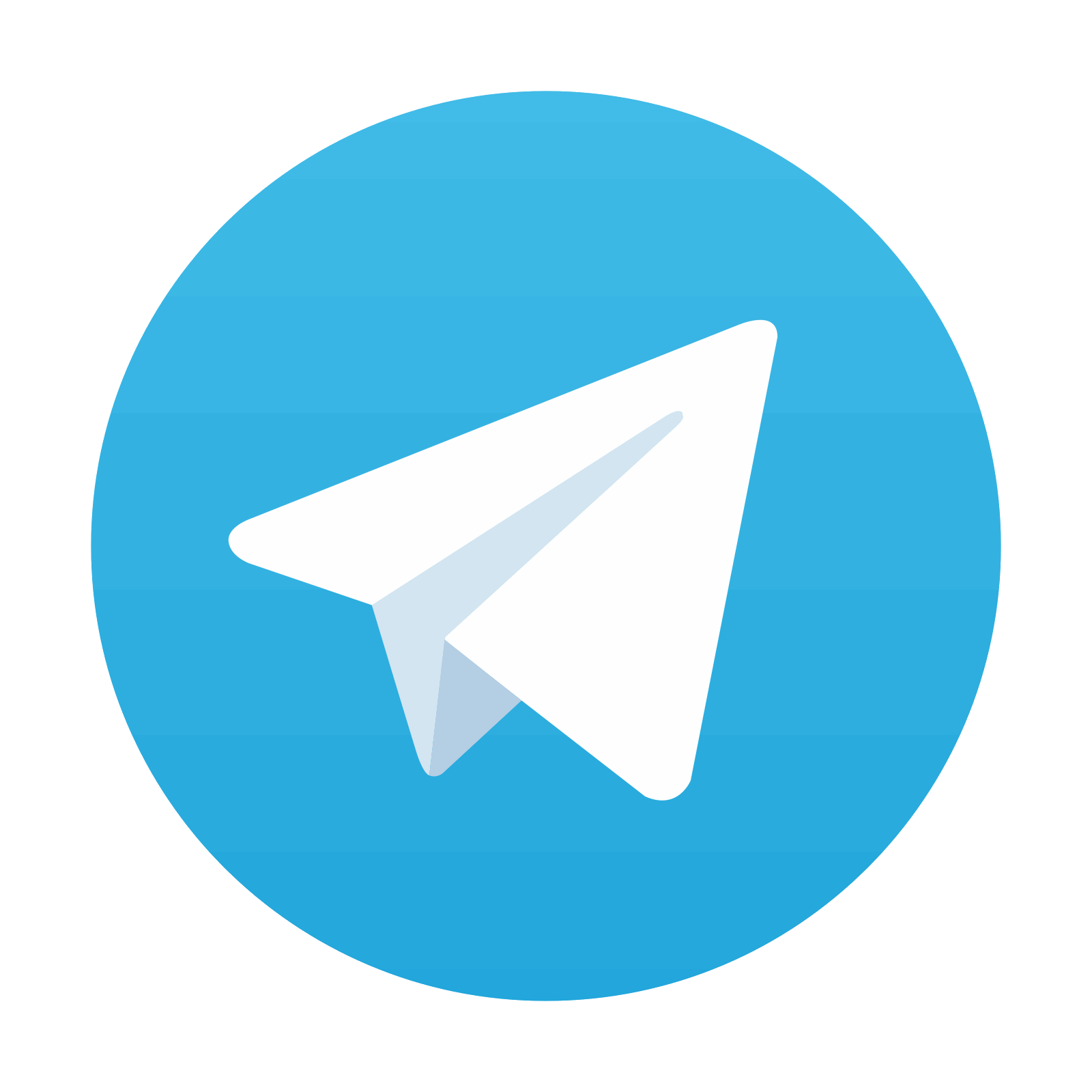
Stay updated, free articles. Join our Telegram channel

Full access? Get Clinical Tree
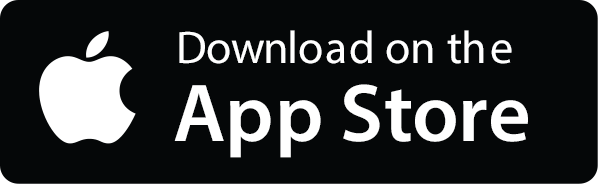
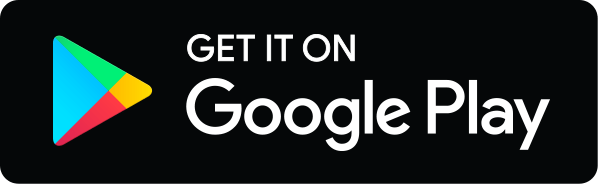