Introduction
Glioblastoma (GBM) is one of the deadliest forms of cancer, with a median survival of approximately 15 months. Standard of care treatment of GBM includes maximal safe surgical resection, fractionated radiotherapy, temozolomide chemotherapy, and, most recently, tumor treatment fields. Despite this combination treatment regimen, tumor progression or recurrence occurs after a median of 7 months. Overall survival of patients with GBM is determined by multiple factors, including the patient’s performance status (Karnofsky performance status [KPS]), age, tumor DNA repair status, and extent of surgical resection.
Despite advances in surgical techniques and radiographic imaging, maximal resection has been shown to be achieved in only 35% of cases. Intraoperative brain shift, poorly demarcated tumor margins, and proximity to eloquent brain all affect the surgeon’s ability to achieve the maximal extent of resection (EOR). Therefore, there is renewed focus on improving the intraoperative imaging and visualization of GBM to overcome these inherent limitations during surgery. This chapter investigates the various surgical adjuncts available to aid in the surgical resection of GBM, namely neuronavigation with MRI, diffusion tensor imaging (DTI), functional MRI (fMRI), intraoperative MRI (iMRI), and intraoperative tumor visualization for fluorescence-guided surgery (FGS).
MRI neuronavigation
Frameless stereotactic navigation devices have been an indispensable tool for tumor removal by neurosurgeons since they were described by Barnett and colleagues in 1993. At present, 2 commonly used devices are the StealthStation S7 (Medtronic, Minneapolis, MN) and the Brainlab Curve (Brainlab AG, Germany) ( Fig. 14.1 ). Patients undergo high-resolution MRI before surgery. Typical sequences used are postcontrast three-dimensional, T1-weighted gradient-echo (magnetization-prepared rapid gradient-echo) and T1-weighted spin echo. These preoperative MRI scans are subsequently uploaded to the device and registration is performed after the patient’s head is immobilized by 3-pin fixation. Registration is accomplished through the use of skin fiducials (paired-point method) or laser surface registration. The surgeon uses a metal pointer on the scalp to assist with planning for the craniotomy and incision. Intraoperative guidance is performed by using a sterile metal pointer in the surgical field to identify anatomic structures and tumor boundaries ( Fig. 14.2 ). When applied in the surgical field, the average radial accuracy has been reported to be 2.4 ± 1.7 mm. Lesions in the frontal lobe have the highest navigation accuracy, whereas infratentorial lesions have the highest rate of error. Periventricular tumors and those with a volume greater than 30 mL also have a higher rate of registration error.


Because frameless stereotactic neuronavigation devices have been present for more than 2 decades at the time of this writing, the main advantages are their time-tested nature and ease of use. Despite these advantages, there are several significant limitations with neuronavigation during GBM resection. First, patient registration can be imprecise, causing delays in operating room workflow. Second, the entry of atmospheric air into the cranial vault, tumor debulking, and the use of hyperosmolar solutions can potentiate brain shift, which exacerbate errors in registration. Navigation errors of a centimeter or more have been reported. These discrepancies can limit the neurosurgeon’s ability to precisely excise the tumor, and increase the frequency of subtotal resections. In addition, the risk of inadvertent violation of eloquent brain is increased with imprecise navigation. Third, there are currently no reliable methods to account for the inaccuracies in navigation caused by brain shift.
With these limitations in mind, tumor neurosurgeons have been progressively examining the use of advanced intraoperative imaging techniques such as DTI, and fMRI (blood oxygen level–dependent fMRI). These advanced magnetic resonance (MR) techniques, which are discussed further in this chapter, have shown promise in improving the EOR and functional outcomes after GBM surgery.
Diffusion tensor imaging
A defining characteristic of GBM is its ability to aggressively infiltrate surrounding white-matter tracts in the brain. Although conventional MR techniques can readily detect the enhancing portions of GBM, they are poor at detecting areas of microscopic invasion at the tumor periphery. These areas of brain invasion at the tumor margin can involve both eloquent and noneloquent white-matter tracts and are not readily visible on conventional T1-weighted/T2-weighted imaging. Because the degree of safe cytoreduction after surgery directly correlates with overall prognosis, the use of DTI has been proposed to address these shortcomings. DTI is a type of diffusion-weighted MR technique that characterizes the molecular diffusion of water to estimate the topographic organization of white matter throughout the brain ( Fig. 14.3 ). This technique provides valuable patient-specific information about subcortical connectivity in relation to the GBM tumor.

The prior characterization of brain tumors with DTI has been well described. Coenen and colleagues first described the intraoperative use of DTI in 2001 in patients with low-grade gliomas and meningiomas. This description was followed by a 2004 study by Berman and colleagues, showing a high correlation between white-matter tracts depicted by DTI and direct cortical stimulation during glioma surgery. In 2007, Wu and colleagues published a randomized controlled trial comparing DTI-guided versus standard frameless stereotactic neuronavigation in patients with gliomas involving corticospinal motor pathways. For high-grade gliomas, the investigators showed improved EOR, decreased incidence of postoperative neurologic deficits (15.3% vs 32.8%), improved 6-month Karnofsky performance score (77 ± 27 vs 53 ± 32), and improved median survival (21.2 months vs 14 months). This study showed class I evidence for the benefit of using DTI versus standard MRI sequences to guide glioma resection involving eloquent cortex. DTI has also shown diagnostic value in differentiating between GBM and metastasis.
The main advantage of DTI is its ability to accurately depict the true extent of tumor margins and surrounding central nervous system connectivity. With this information, neurosurgeons are better equipped to plan a more patient-tailored approach to tumor resection. One of the major disadvantages of DTI is its reliance on region-of-interest (ROI) selection to reconstruct fiber pathways. ROI analysis can be time consuming and difficult in the setting of brain shift and edema caused by tumor. As these processes become increasingly automated, this cumbersome postacquisition analysis will likely improve. Similar to conventional neuronavigation, another disadvantage of DTI is its inability to account for dynamic changes in the operating room, such as brain shift caused by pneumocephalus. Therefore, there is increased focus in using multimodal techniques such as fluorescence-guided surgery (FGS) in conjunction with advanced imaging techniques to improve outcomes in GBM surgery.
Functional and intraoperative MRI
The use of iMRI has been more widely implemented for GBM resection over the past decade, since its introduction in the late 1990s. The main objectives of iMRI are to update source imaging for neuronavigation use (minimize brain shift) and to identify any remaining contrast-enhancing tissue. Several retrospective studies have been reported in the literature over the past decade that offer the potential advantages of iMRI use. Hirschberg and colleagues provided a retrospective analysis of 32 patients with GBM and showed that the iMRI group had a median survival of 14.5 months compared with 12.1 months for the control group. Lenaburg and colleagues reported on 35 GBM cases in 29 patients in whom the use of iMRI led to further resection in 72% of cases and thereby resulted in a tumor resection of greater than 95% in 27 out of 35 cases. Senft and colleagues published a retrospective report on 41 patients with GBM in which they compared the use of iMRI in 10 patients with conventional neuronavigation in 31 patients. Gross total resection (GTR) was observed in all 10 iMRI cases versus 19 of 31 conventional cases, which translated to a median survival of 74 weeks for the GTR group compared with 46 weeks for the subtotal resection group ( P <.001). This finding in turn led to a median survival of 88 weeks for the iMRI group versus 68 weeks for the conventional group ( P = .07). Napolitano and colleagues reported on 94 GBM resections over a 5-year period. They compared the use of iMRI versus conventional imaging and found that the rate of resection increased by 17.8% up to 73.2% of GTR/near-total resection (NTR). This greater EOR, in turn, led to greater overall survival, with a median survival of 15.26 months for the GTR/NTR group versus 10.26 months for the partial resection group ( P = .049). Another study investigating tumor EOR and iMRI use for 135 patients with GBM showed that iMRI found residual tumor volume in 88 patients, leading to further surgical resection in 19 of those patients and GTR for 9 of those patients. This finding led to a survival benefit in those patients with an EOR greater than or equal to 98% and a median survival of 14 months compared with 9 months for patients with an EOR less than 98% ( P <.0001). Regardless, Kuhnt and colleagues showed the influence of iMRI on EOR, as shown in Table 14.1 .
Resected Tumor Volume (%) | First iMRI (# of Patients) | Final iMRI (# of Patients) |
---|---|---|
100 | 0 | 9 |
99.9–98.0 | 0 | 1 |
97.9–95.0 | 3 | 0 |
94.9–90.0 | 1 | 1 |
<90.0 | 15 | 8 |
A randomized, prospective trial by Senft and colleagues of 58 patients with newly diagnosed GBM underwent resection with either the use of iMRI or conventional computer-assisted neuronavigation. The control group had a 68% GTR rate compared with 96% GTR for the iMRI group on blinded neuroradiological assessment ( P = .023). This study provided level 1 evidence supporting a higher GTR of GBM tumors with the use of iMRI. However, this difference in EOR did not lead to a significant difference in progression-free survival (PFS) at 6 months. It is worth noting a recent study by Zhang and colleagues, in which they combined the use of iMRI and functional neuronavigation with preoperative fMRI and DTI. They studied 198 patients with glioma; 112 underwent surgery combined with iMRI and functional neuronavigation, and 86 patients were in the control group. The study group had a greater EOR (95.5% vs 89.9%; P <.001) and GTR rate (69.6% vs 47.7%; P = .002) in addition to greater language preservation as graded by the aphasia quotient. Moreover, they also showed greater overall survival for their study group (19.6 months vs 13.0 months; P <.001) with PFS double that of the control group (12.5 months vs 6.6 months; P = .003). Therefore, they showed a potential role for this combined preoperative functional neuronavigation assessment with the use of iMRI. Future studies are needed to assess whether factors such as the dose and timing of the contrast agent, the MRI field strength, and the location of the tumor play a role in achieving greater efficacy for iMRI use.
Most recently, the use of whole-brain proton spectroscopic MRI (sMRI) was used to define the tumor margin in patients with GBM in combination with 5-aminolevulinic acid (5-ALA) tumor fluorescence and histopathology. Whole-brain sMRI metabolite maps were coregistered with MRI neuronavigation to guide neurosurgical tumor sampling. sMRI metabolite abnormalities were located centimeters away from the contrast-enhancing border of GBM tumors, confirming the infiltrative border of GBM tumors and providing a basis for understanding recurrence patterns of GBM tumors.
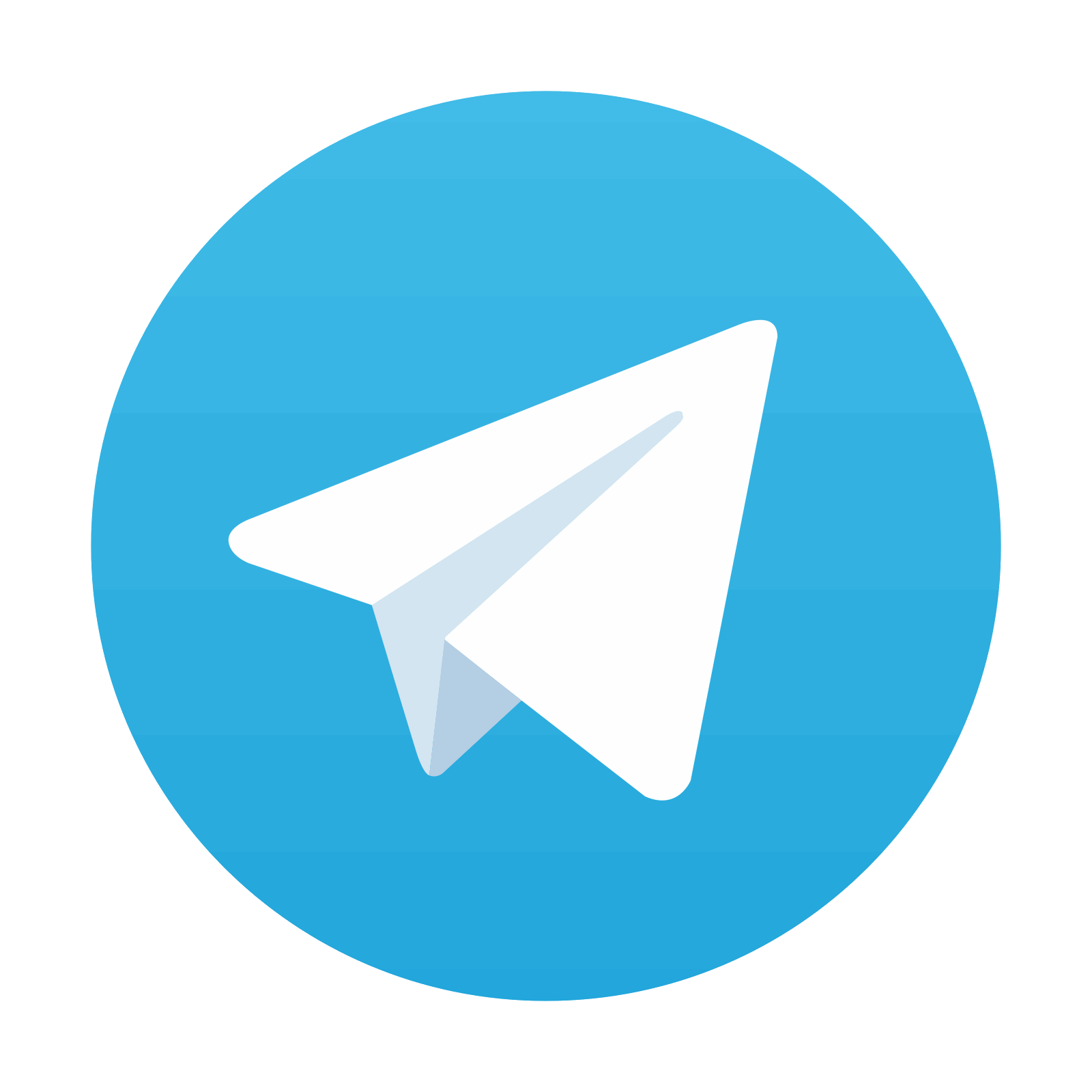
Stay updated, free articles. Join our Telegram channel

Full access? Get Clinical Tree
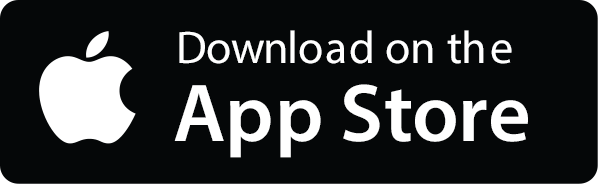
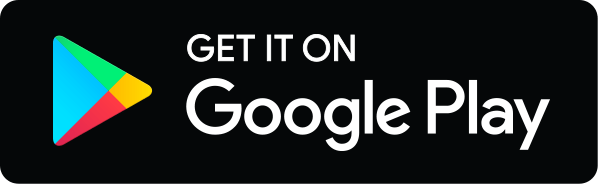