Summary of Key Points
- •
Intraoperative monitoring in spine surgery is a surrogate for a neurologic examination in the anesthetized patient. Modalities employed include somatosensory evoked potentials, motor evoked potentials, and spontaneous or triggered electromyography activity.
- •
The use of neuromonitoring in routine surgery should be at the discretion of the operating surgeon. Individual practice patterns vary significantly.
- •
Intraoperative neuromonitoring has been shown to predict adverse outcomes of paraparesis, paraplegia, and quadriplegia in spine surgery. Evidence supporting its use in deformity surgery is particularly compelling.
The purpose of intraoperative monitoring in spine surgery is to provide timely information on the electrophysiologic integrity of the spinal cord and nerves, thereby helping a surgeon to prevent new neurologic deficits. It is a surrogate for a neurologic examination in the anesthetized patient.
Brief History
Neuromonitoring techniques have been a component of spine surgery since the 1970s when somatosensory evoked potentials (SSEPs) were used to monitor spinal cord function during the surgical correction of scoliosis. Since the genesis of intraoperative monitoring in the 1970s, there has been a dramatic evolution in the use of SSEPs, and as a result they remain the mainstay of spinal cord monitoring. Nuwer and colleagues published a landmark paper in 1995 evaluating the clinical efficacy of intraoperative SSEP monitoring performed during scoliosis surgery in a large multicenter survey of 51,263 spine surgeries. They reported an overall sensitivity of 92% and specificity of 98.9% in the ability of SSEPs to detect new postoperative neurologic deficits.
How They Work
Intraoperative monitoring enables evaluation of sensory and motor pathways. The most common techniques include SSEPs, motor evoked potentials (MEPs), and spontaneous or triggered electromyography (EMG) activity.
SSEPs
SSEP monitoring primarily evaluates the dorsal column-medial lemniscal pathway. It is useful, however, in providing a global assessment of cord function given the assumption that many mechanisms of intraoperative injury would affect the cord diffusely. The monitoring of upper extremity SSEPs usually involves stimulating the median or ulnar nerve near the wrist. These impulses are transmitted through the stimulated nerve, brachial plexus, dorsal columns, dorsal column nuclei, medial lemniscus, thalamus, and to the primary sensory cortex. Potentials can be seen and recorded at various points along this pathway. Similarly, lower extremity SSEPs pass the popliteal fossa, cauda equina, and on to the dorsal columns/somatosensory cortex as with the upper extremity.
SSEP responses are of low amplitude, frequently less than 1µV. As the amplitude is less than the surrounding normal cerebral EEG activity, many recordings are made time-locked to multiple stimuli and averaged together to filter out external noise. Hence, there may be a delay of up to 3 to 5 minutes in determining if a significant change has occurred depending on the signal-to-noise ratio. The risk of a neurologic deficit increases with prolonged decrease (greater than 50%) in amplitude and increase in latency. It is important to recognize these changes as early as possible so immediate intervention may be employed if feasible. Transient moderate loss of amplitude incurs a medium risk of deficit. Sudden sustained loss of SSEPs carries the worst prognosis, although a deficit is not always certain (quoted incidence of 50% to 75%).
SSEPs are certainly a good indicator of spinal cord function but not of nerve root function. SSEPs are typically elicited by stimulation of either the posterior tibial (lower limb SSEPs) or the median nerve (upper limb SSEPs). Hence, the integrity of every other peripheral nerve is not monitored and unknown. Dermatomal SSEPs have been developed to analyze specific segmental dermatomes. There is a theoretic benefit to the use of dermatomal SSEPs to study nerve root function, but in practice they do not correlate well with clinical outcome. Direct EMG recordings provide better information on individual nerve roots (discussed later).
Because SSEPs are transmitted through the dorsal columns of the spinal cord, it is possible (for example) to incur injury to the anterior spinal artery resulting in anterolateral column deficits while sparing the dorsal columns. Therefore, some patients may still develop neurologic deficits despite unchanged SSEPs. However, because most cord injuries do not discriminate between anterior and posterior spinal pathways, stable intraoperative SSEPs are generally associated with a favorable outcome.
SSEPs are adversely affected by halogenated (inhalational) anesthetics such as halothane and isofluorane.
MEPs
Motor evoked potentials have been in use since the early 1990s. They were pioneered by Merton and Morton in 1980. The first device designed for producing MEPs was approved by the Food and Drug Administration (FDA) in 2002, and since then they have been available on a routine basis. Prior to the widespread use of MEP monitoring, the only way to assess corticospinal tract integrity and the resulting motor function during surgery was the Stagnara wake-up test, which involved waking patients during surgery and asking them to move their feet. MEPs are used to detect injury to the anterolateral columns of the spinal cord. They are particularly sensitive in detecting iatrogenic injury, both mechanical and ischemic.
Similar to SSEPs, MEPs are sensitive to a number of anesthetic agents due to an inhibitory effect on cortical axon synapses and spinal anterior horn cells. At the time of Merton and Morton’s experiments, halogenated anesthetics and nitrous oxide were commonly used in spine surgeries. The tendency of these anesthetics to depress motor neuron activity was quickly discovered, and intravenous anesthesia was introduced, initially using fentanyl and propofol, to mitigate this effect. Therefore, when monitoring MEPs the use of total intravenous anesthesia (TIVA) is recommended. Even when TIVA is employed, high levels of propofol may suppress α-motoneurons, so this should be taken into consideration when interpreting MEP loss. Although it may introduce additional challenge to the anesthesia team and surgeon because of patient movement and the absence of paralyzing agents (neuromuscular blockade), TIVA offers clear benefits in obtaining MEPs over inhaled anesthetic agents. An interdisciplinary effort among the surgical, neuromonitoring, and neuroanesthesia teams is imperative.
The generation of MEPs involves applying a magnetic or electric stimulus to the scalp, which must then travel through bone to activate the motor cortex beneath. Hence, relatively large stimulation intensities are involved, commonly co-activating local musculature such as the temporal, masseter, and occipital muscles. Following motor cortex activation, a distal muscle contraction (arm or leg) can be recorded (MEP) or a nerve action potential (“direct” or “D” wave). D waves are measured by an electrode positioned in the epidural space somewhere over the cervical or thoracic spinal cord. They are produced by direct stimulation of the hemispheric white matter. D waves are the first wave detected, and successive waves are referred to as indirect or I waves. Unlike D waves, I waves require direct synaptic activity and are therefore suppressed by general anesthesia. Muscle MEPs are, however, most commonly recorded and give the surgeon instantaneous feedback.
In addition to the motor cortex, stimulation can be performed in the spinal cord directly with recording electrodes either on nerve or in muscle. Although this technique offers the advantage of being less sensitive to anesthetic agents, responses obtained via direct spinal cord stimulation are less likely to represent motor function but rather antidromic sensory responses.
Although MEPs are the current optimal modality for motor tract monitoring, they are not without their limitations. Unlike SSEPs, which offer continuous monitoring, MEPs must be acquired intermittently and their acquisition is more difficult technically. Best quoted acquisition rates in the upper extremities for MEPs are 95% (versus 98% for SSEPs) and 67% in the lower extremities (versus 93% for SSEPs). MEPs are contraindicated in patients with deep brain stimulators and cochlear implants.
Aside from potential anesthetic complications, the most common complication associated with MEPs is tongue-biting in patients without neuromuscular blockade. Other safety considerations relate to high voltage and current.
EMGs
Intraoperative EMG monitoring facilitates detection of iatrogenic injury and indicates the functional status of peripheral nerves. Spontaneous or triggered EMGs can be monitored. Spontaneous EMGs do not involve stimulation. Rather, surgical manipulation induces spontaneous firing, which manifests as compound muscle action potentials (CMAPs) and potentially indicates mechanical injury. Ischemic injury is poorly detected by spontaneous EMG. Careful muscle selection and electrode placement is essential, as there may be variability in activity within individual muscles. It is also important to distinguish acute denervation from chronic denervation. In the presence of preexisting nerve root irritation, intermittent low amplitude patterns will be seen on baseline EMG. Intraoperative events correlate with the frequency, amplitude, and duration of firing. Increased frequency and increased amplitude reflect a greater potential for damage. Continuous or persistently abnormal EMG refractory to reversal of a surgical maneuver is concerning for nerve injury.
Triggered EMGs are most commonly used in screw placement to warn of proximity to a nerve root. Bone conducts electricity very poorly. If an electrical impulse is delivered to a screw or a probe within bone, an adjacent nerve root should remain quiescent except at very high stimulus levels. However, in the setting of (for example) a pedicle breach, the threshold stimulus intensity required to cause EMG activity is greatly reduced.
The use of multimodality intraoperative neurophysiologic monitoring incorporating the use of SSEPs, MEPs, and both spontaneous and triggered EMGs allows optimal detection of neurologic injury.
Use in Routine Spine Surgery
Several professional societies have issued guidelines on the use of neuromonitoring, but these guidelines relate to the technical aspects and predictive value of monitoring rather than the indications for monitoring in routine spine surgery. The argument that monitoring should be used in all spine surgeries is not realistic. This would obviously not be cost effective. If the risk of deficit with a particular surgery is negligible, it is difficult to justify the utilization of monitoring in a carte blanche fashion. Anterior cervical discectomy and fusion (ACDF) is a good example of a routine surgery with a low risk of neurologic deficit but where debate exists about the value of monitoring these cases. In ACDF, one study demonstrated that the rate of cord injury in patients without deficit preoperatively was less than 0.1% and also demonstrated no difference in outcome between patients who were and were not monitored intraoperatively. The researchers concluded in their series that transient SSEP signal changes, which improved with intraoperative interventions, were not associated with new postoperative neurologic deficits and that an intraoperative neurologic deficit is possible despite normal SSEP signals. Another study concluded that decompression and reconstruction for symptomatic cervical spine disease without intraoperative monitoring may reduce the cost of treatment without adversely impacting patient safety. A systematic review of electrophysiologic monitoring during surgery for cervical degenerative myelopathy and radiculopathy found that the reliance on changes in evoked potential monitoring as an indication to alter a surgical plan or administer steroids has not been observed to reduce the incidence of neurologic injury during routine surgery for cervical spondylotic myelopathy or cervical radiculopathy. Accordingly, the authors recommended against its routine use in these circumstances. There are contrasting views, however. In fact, that systematic review added the caveat that there may be circumstances in which the surgeon desires additional intraoperative diagnostic information that electrophysiologic monitoring may confer. Most studies are retrospective in design and include nonmyelopathic patients as well as myelopathic patients. Patients with severe myelopathy may have a higher relative risk of a worse postoperative deficit.
Stecker looked at various cost-effectiveness arguments relating to the use of monitoring. The variables include the cost of providing the monitoring itself, the operative cost of responding to a warning, the cost of an injured patient, the probability that a warning is issued, the conditional probability that the warning is associated with a true injury, and the probability that a true warning could be acted upon to prevent injury. Analysis suggested that in the setting of ACDF, any advantage from intraoperative monitoring would be marginal. Stecker acknowledged that this formulaic approach to cost-effectiveness does not include advantages that might be gained if costs of monitoring were used for another service that might benefit the patient. In conclusion he stated that the advantage of monitoring is clear for younger patients undergoing scoliosis surgery but negligible for elderly patients with multiple comorbidities undergoing ACDF.
Ultimately the use of neuromonitoring in routine surgery should be at the discretion of the operating surgeon. Individual practice patterns vary significantly, even within the authors’ group.
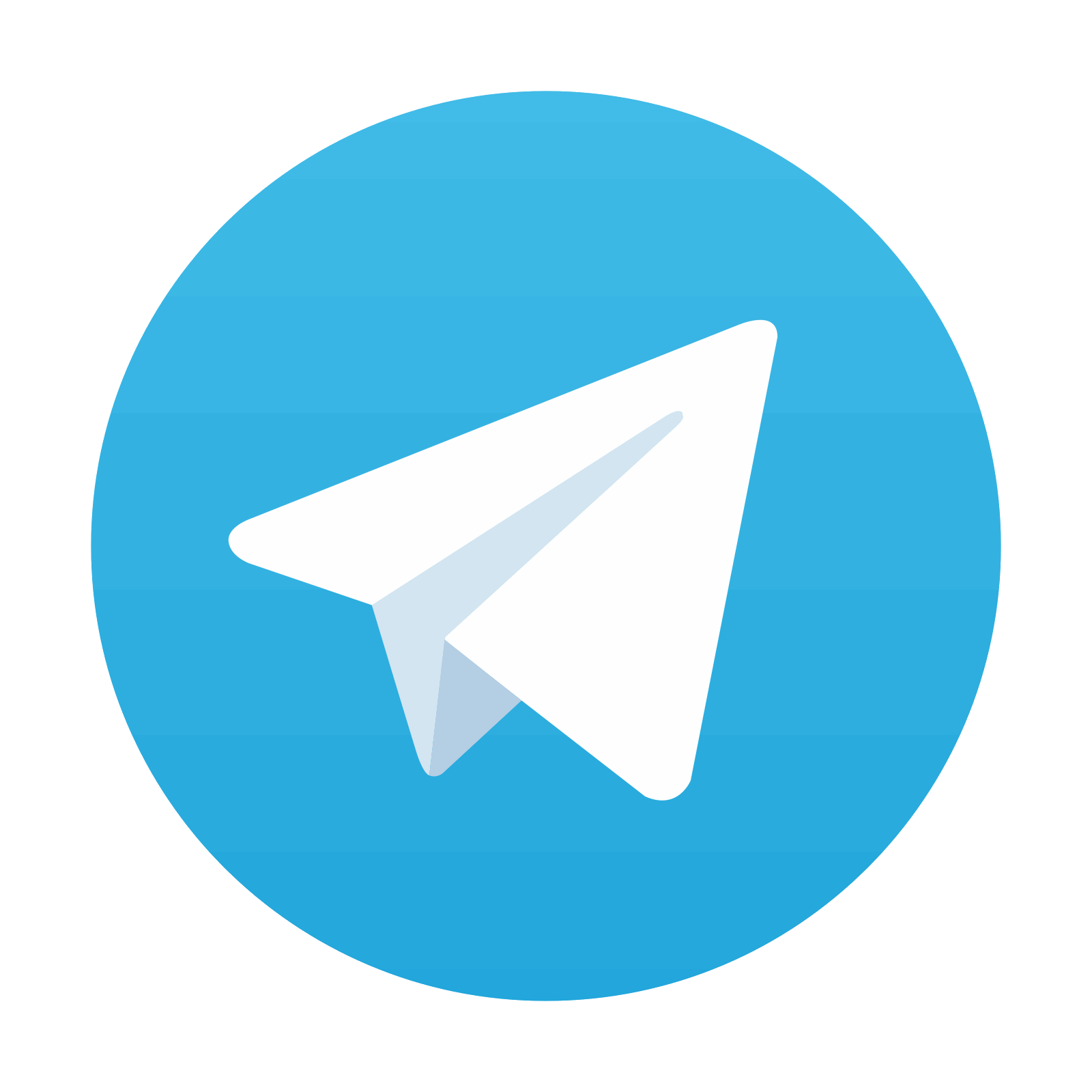
Stay updated, free articles. Join our Telegram channel

Full access? Get Clinical Tree
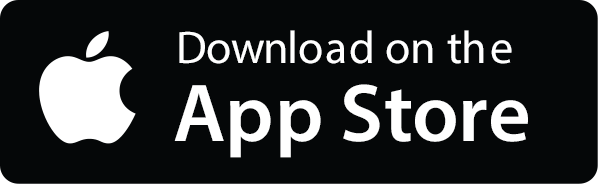
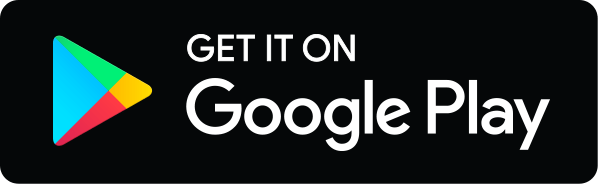