Intraoperative MRI (iMRI) is a neurosurgical adjunct used to maximize the removal of glioma, the most common primary brain tumor. Increased extent of resection of gliomas has been shown to correlate with longer survival times. iMRI units are variable in design and magnet strength, which can affect patient selection and image quality. Multiple studies have shown that surgical resection of gliomas using iMRI results in increased extent of resection and survival time. Level II evidence supports the use of iMRI in the surgical treatment of glioma.
Key points
- •
Intraoperative MRI (iMRI) is a neurosurgical adjunct used to maximize the removal of glioma, the most common primary brain tumor.
- •
Increased extent of resection of gliomas has been shown to correlate with longer survival times.
- •
iMRI units are variable in design and magnet strength, which can affect patient selection and image quality.
- •
Multiple studies have shown that surgical resection of gliomas using iMRI results in increased extent of resection and survival time. Level II evidence supports the use of iMRI in the surgical treatment of glioma.
Introduction
Surgery for Glial Neoplasms
Surgical resection is a mainstay of a multidisciplinary approach to the management of gliomas. Numerous retrospective studies have shown that maximizing the extent of resection of gliomas improves overall survival time. Intraoperative MRI (iMRI) is a surgical adjunct designed to help the operating surgeon maximize the removal of glial tumors. During surgical resection, brain shift, surgically induced edema, and volume loss from resection can render traditional image guidance systems inadequate. These systems rely on a preoperative MRI for registration and navigation and therefore cannot compensate for the anatomic and physiologic changes that occur during surgery. iMRI incorporates an MRI scanner in the operating room, allowing the surgeon to obtain an MRI during the course of surgery, thus providing “real-time” feedback. iMRI can be used to assess the extent of tumor resection, assess changes in neuroanatomic structures, and incorporate changes in functional cortical and subcortical brain into the stereotactic navigation system. Residual tumor can then be resected while the patient is still in the operating room with an exposed craniotomy. The nature of a glial neoplasm influences how the operating neurosurgeon uses the iMRI. In this article, iMRI in the context of glioma resection is reviewed.
Radiographic Qualities of Glial Tumors
High-grade glial neoplasms, such as glioblastoma (World Health Organization [WHO] grade 4 brain tumors), generally demonstrate extensive enhancement on postcontrast T1-weighted MRIs ( Fig. 1 ). Low-grade glial neoplasms (WHO grade 2 brain tumors) typically do not demonstrate significant enhancement but do display hyperintensity on T2-weighted MRI or fluid-attenuated inversion recovery (FLAIR) sequences ( Fig. 2 ). These differences in imaging characteristics must be taken into account during surgery in the iMRI operating environment. In this article, these tumors will be dichotomized into low-grade (non–contrast enhancing) and high-grade (contrast enhancing) gliomas. Low-grade gliomas (LGGs) are WHO grade 2 tumors, including oligodendroglioma and astrocytoma. High-grade gliomas are WHO grade 3 and 4 tumors, including anaplastic oligodendroglioma, anaplastic astrocytoma, and glioblastoma.
Introduction
Surgery for Glial Neoplasms
Surgical resection is a mainstay of a multidisciplinary approach to the management of gliomas. Numerous retrospective studies have shown that maximizing the extent of resection of gliomas improves overall survival time. Intraoperative MRI (iMRI) is a surgical adjunct designed to help the operating surgeon maximize the removal of glial tumors. During surgical resection, brain shift, surgically induced edema, and volume loss from resection can render traditional image guidance systems inadequate. These systems rely on a preoperative MRI for registration and navigation and therefore cannot compensate for the anatomic and physiologic changes that occur during surgery. iMRI incorporates an MRI scanner in the operating room, allowing the surgeon to obtain an MRI during the course of surgery, thus providing “real-time” feedback. iMRI can be used to assess the extent of tumor resection, assess changes in neuroanatomic structures, and incorporate changes in functional cortical and subcortical brain into the stereotactic navigation system. Residual tumor can then be resected while the patient is still in the operating room with an exposed craniotomy. The nature of a glial neoplasm influences how the operating neurosurgeon uses the iMRI. In this article, iMRI in the context of glioma resection is reviewed.
Radiographic Qualities of Glial Tumors
High-grade glial neoplasms, such as glioblastoma (World Health Organization [WHO] grade 4 brain tumors), generally demonstrate extensive enhancement on postcontrast T1-weighted MRIs ( Fig. 1 ). Low-grade glial neoplasms (WHO grade 2 brain tumors) typically do not demonstrate significant enhancement but do display hyperintensity on T2-weighted MRI or fluid-attenuated inversion recovery (FLAIR) sequences ( Fig. 2 ). These differences in imaging characteristics must be taken into account during surgery in the iMRI operating environment. In this article, these tumors will be dichotomized into low-grade (non–contrast enhancing) and high-grade (contrast enhancing) gliomas. Low-grade gliomas (LGGs) are WHO grade 2 tumors, including oligodendroglioma and astrocytoma. High-grade gliomas are WHO grade 3 and 4 tumors, including anaplastic oligodendroglioma, anaplastic astrocytoma, and glioblastoma.
Extent of resection and outcome
Several recent studies have demonstrated an advantage for maximizing the resection of both high- and low-grade glial neoplasms. Although Level I evidence is lacking for surgical resection (compared with a nonsurgical intervention), retrospective studies examining volumetric resection have demonstrated a survival advantage for more completely resected tumors. For glioblastoma, more complete resection of the enhancing disease is associated with a survival benefit. More recent molecular characterization of high-grade glioma has shown that isocitrate dehydrogenase 1 mutant tumors benefit from resection of the enhancing and, if present, nonenhancing component as well.
Lacroix and colleagues showed that survival benefit with resection of ≥89% of enhancing tumor. This survival benefit was most profound after resection of ≥98% of the enhancing disease. In another retrospective series of 500 patients with glioblastoma, resection of 78% of enhancing tumor was the threshold at which a survival advantage was achieved. This advantage increased as more tumor was removed. In a retrospective review of 128 patients, residual tumor volume after resection was shown to influence survival with a statistically significant benefit in survival with less than 2 cm 3 of residual enhancing disease. Taken together, these studies underscore the value of maximizing the extent of resection of glioma to improve outcomes.
LGGs have been dichotomized by risk into low- and high-grade groups using specific criteria. However, extent of tumor resection was not among these characteristics. In the not-so-distant past, a conservative approach involving frequent observation had been advocated with an assumption that some low-grade tumors would remain stable for years. It is clear that, given enough time, LGGs will progress, with a potential for transformation to a more malignant tumor. Studies advocating an aggressive surgical approach for the treatment of LGG are relatively recent. Retrospective studies have shown an incremental increase in progression-free and overall survival time with increased resection. Perhaps the most definitive study to date was reported by Jakola and colleagues, which studied the differences in outcome (including overall survival and rates of malignant transformation) between the strategies of biopsy and surgical resection. Here, the investigators found a significant survival advantage to surgical resection. Patients undergoing surgery had a median survival that was not reached during the study period compared with 5.9 years for patients who underwent biopsy alone. Incomplete surgical resection has also been identified as a feature of “high-risk” LGGs. The most recent prospective randomized trial (RTOG 9802) for the treatment of LGGs compared radiation therapy with radiation therapy plus chemotherapy-excluded patients who had a gross total resection of their tumors owing to the favorable impact surgery has on survival time.
Thus, surgical strategies to maximize both low- and high-grade glioma removal during surgery are supported by available studies. As an operative adjunct, the iMRI is designed to provide the operating surgeon with the most accurate depiction regarding the extent of tumor resection so that the amount of tumor removed can be maximized. Volumetric MRI is now the standard for assessing extent of resection and amount of residual tumor and can be obtained in the intraoperative setting. Surgeon-determined extent of resection has been shown to be unreliable and can have a negative impact on survival if not objectively assessed. iMRI can therefore be used to objectively determine the amount of residual tumor during resection, permitting continued removal in a single operation.
History of intraoperative MRI
iMRI has gone through several iterations over the past 2 decades. Improvements in magnet strength (measured in Tesla), which correlates with image quality, and design, which allows surgeons better access to the surgical field, have been the primary changes over time. Software enhancements have also improved the information that can be obtained during the course of surgery.
iMRI scanners can be characterized by the strength of the magnets. Ultra-low-field magnets (0.2 T or less), low-field magnets (0.5 T), and high-field magnets (≥1.5 T) have all been produced. The first iteration of the low-field iMRI was developed in the early 1990s. In this configuration, the operating table is positioned between 2 magnets. This arrangement is termed the “double doughnut” given the proximity of the 2 bores to each other. The narrow space for the surgical field did introduce some limitations in patient positioning and surgeon comfort with respect to accessibility to the patient’s head. One of the most commonly used low-field iMRI units was the PoleStar (Odin/Medtronic) with a field strength of 0.12 T. The scanner is positioned underneath the operating room table, which allows for the use of regular instruments. The scanner is then moved upwards to the patient’s head for scanning. These low-field iMRI units typically have open configurations, which, coupled with lower field strength, compromises image quality (compared with high-field units). They also require longer times for image acquisition. Contrast-enhancing lesions are suitably visualized on low-field iMRI units because the enhancing tumor is discernible even at low-field strength. However, low-grade tumors, which are better seen with T2-weighted or FLAIR sequences, are not as well visualized and it is difficult to discriminate them from normal surrounding brain. Nevertheless, the utility of low-field iMRI has been evaluated for both low- and high-grade gliomas. High-field iMRI scanners provide improved image quality but also require increased space and technological capabilities and are significantly more expensive than low-field units. Most are 1.5 T, although 3-T magnets are in production. Modern high-field MRI units typically consist of an operating bed that is MRI compatible and a magnetic bore in a closed configuration ( Fig. 3 ). The bore may move to the operating table or vice versa, and some units are ceiling mounted making them accessible to more than one room. These larger bores naturally must be able to accommodate the patient and the operating table. Patient habitus, weight, and positioning must all be taken into consideration before positioning the patient within the bore. Systems for fixation of the head also vary depending upon the manufacturer. Virtually all head fixation systems incorporate the radiofrequency coils (usually upper and lower) into the fixation apparatus. Some head holders resemble a typical 3-point fixation head clamp that can then be affixed to the operating table. These head holders permit typical pinning configurations that are common in the regular operating room. Some are more rigid and may not accommodate certain pinning configurations because they are already attached to the operating table ( Fig. 4 ).

Full access? Get Clinical Tree
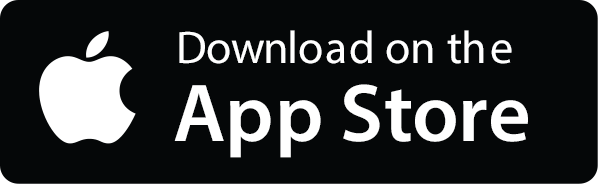
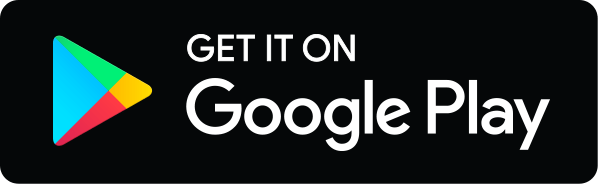