17 Hemostatic techniques, including metal clips, mechanical tamponade, and ligature, used in other forms of surgery have some utility in neurosurgery; however, the delicate and friable nature of neural tissues has led to the development of other hemostatic techniques. Meticulous hemostasis must be adhered to because of surgeons’ general intolerance for blood accumulation while they are working in a small surgical cavity, intracranially or in the spine.1 In general, there is a lack of basic understanding about how to use these agents, even among practitioners, as there is a paucity of studies that address this topic specifically. Thorough understanding of the mechanisms of action and of the advantages and disadvantages of the various materials and techniques available is crucial for safe and efficacious surgery. This chapter discusses some of the chemical, electrical, thermal, and adjunctive methods for hemostasis in use in neurosurgery today. The first descriptions of hemostasis date back to ancient Egypt, where descriptions of thermal cautery have been found, ranging from the use of hot irons and stones to boiling oil. Described by Galen, this was the preferred method of hemostasis for most of antiquity. Although effective, using conductive heat to induce hemostasis caused damage to the surrounding tissues. The advantage of this technique was that it worked quickly and effectively, which was certainly a necessity in times when anesthetics and asepsis were not available.2 In the 1500s, Ambroise Paré pioneered the use of ligature for hemostasis rather than cauterization during amputation. To achieve this, he designed and used the “bec de corbin,” a predecessor of the modern hemostat. Sterilization and aseptic technique had not been developed, and therefore, the use of ligature often led to the spread of infection. However, this was a breakthrough, and it remains a mainstay of modern surgical technique.3 In the 1920s, a milestone in hemostasis was achieved with the invention of monopolar electrocautery by William T. Bovie and Harvey Cushing. By using induction rather than conduction to transfer heat to tissues, the area of heat generation could be more finely controlled. First used in 1926 in an operation, by Cushing, this predecessor to modern monopolar electrocautery is still the most widely used method to achieve hemostasis in surgery.4 Descriptions of chemical hemostasis with styptics and caustics can be found in the Hippocratic Corpus. Styptics, substances that induce vasoconstriction, date back to the early Greeks and Romans, who used vegetable and mineral styptics for the treatment of battle wounds. In the Middle Ages, copper sulfate was used as a caustic. Caustics produced hemostasis by denaturing proteins indiscriminately, with uncertain and unreliable quality.2 Still, the use of caustic substances remained popular until the introduction of ligature. The advantage of chemical caustics was that they could be used on capillary bleeding, too small for ligatures. As technology advanced, less reactive and destructive substances have been developed. Mechanical hemostasis, an effective technique for stopping surgical and traumatic bleeding, dates back to the Romans, who used straps of bronze and leather as tourniquets to reduce bleeding during amputations.5 Before the advent of microsurgery and bipolar coagulation, neurosurgeons would have to stop frequently to apply pressure via cotton packs to stop bleeding, thus leading to long surgical times. The tissues had a tendency to rebleed once the packs were removed. To avoid rebleeding, Cushing used skeletal muscle instead of cotton packs to induce mechanical hemostasis. The muscle could be left permanently; however, the use of muscle was discontinued due to unacceptable tissue reactions.6 With the introduction of gelatin sponges, mechanical hemostasis continues to be widely used in neurosurgery even today. First used in 1945, Gelfoam (Upjohn, Kalamazoo, MI) and Surgifoam (Ethicon, New Brunswick, NJ) (Table 17.1) are water-insoluble absorbable sponges prepared from purified porcine skin gelatin. The hemostatic properties are primarily due to mechanical hemostasis, as it is capable of absorbing up to 45 times its weight of whole blood.7 It may also act as a supportive structure, producing a matrix that facilitates clotting as well as forming an artificial clot.8 It has been proposed that platelets enter the sponge, then release thromboplastin after being activated by contact with the walls of the interstices of the sponge. The thromboplastin then reacts with calcium and prothrombin, causing the production of thrombin, thus initiating the clotting cascade.9 The gelatin sponge may be used alone or soaked with saline, but is often soaked with thrombin (Pfizer, New York, NY), thereby supplementing its mechanical hemostatic properties with the chemical hemostatic properties of thrombin. If used in concert with thrombin, excess thrombin should be removed from the gelatin sponge. The sponge is applied directly to the bleeding surface. A cotton pledget is then applied on top of the sponge and held for at least 10 seconds, whereupon the pledget may usually be removed. To achieve maximum utility, blood should be allowed to soak to the outer surface of the sponge. The gelatin sponge may also be cut to a smaller thickness, and may be sliced as small as 2 mm without fragmenting. The thinnest effective sponge should be used to achieve hemostasis, as Gelfoam swells tremendously.10 A principal disadvantage of the gelatin sponge is its tendency to leak plasma after clot formation.11 In addition, because of its capacity to swell, compression of neural structures can result. There have been multiple case reports of symptomatic compression of brain and spinal structures, including cauda equina syndrome, stenosis, and paresthesias.12 Although Gelfoam is thought to be relatively nonreactive, there have been case reports of giant-cell granuloma formation at the implantation site.13 The time to absorption depends on several factors, including the site used, saturation with bodily fluid, and the amount used. By 4 to 6 weeks after implantation into soft tissues, the gelatin sponge is usually completely absorbed.9,14–16 By contrast, when used on bleeding nasal, rectal, or vaginal mucosa, it has been observed to liquefy within 2 to 5 days. The cellular reaction observed has been reported to be similar to that of natural clot resorption and does not seem to cause excessive scar formation.15 Still, it should not be used in the closure of skin incisions, as the gelatin sponge may interfere with the healing of skin edges due to the interposition of the sponge between the tissue surfaces. In addition, Gelfoam should not be used with autologous blood salvage circuits, as it may theoretically result in disseminated intravascular coagulation.17 Although non-antigenic, the gelatin sponge is still a foreign body, and thus may serve as a nidus for infection. Several studies have shown increased infection, especially when used in contaminated fields.18–19 Consequently, the minimum amount of gelatin sponge should be used if it will potentially be left in the surgical field. Additionally, mixing antibiotic sponges with the gelatin sponge is not recommended. The gelatin sponge is also available in a variety of forms. Gelfoam Plus (Baxter, Deerfield, IL) is a Gelfoam sponge that comes prepackaged with thrombin. It is also available in powder form mixed with thrombin (Floseal, Baxter Deerfield, IL; Surgiflo, Ethicon, New Brunswick, NJ), which is particularly useful for cancellous bone bleeding. There are two commercially available forms of oxidized cellulose: oxidized cellulose (Oxycel, Becton Dickinson, Sandy, UT) and regenerated oxidized cellulose (Surgicel, Ethicon, New Brunswick, NJ). Both are available in a variety of forms and preparations including pads, strips, and powder. Oxidized cellulose consists of cellulose that is subjected to oxidation by nitrous oxide.20 This oxidation causes conversion of hydroxyl radicals on the cellulose to become carboxyl groups, leading to a functional unit consisting of anhydroglucuronic acid.21 Surgicel, regenerated oxidized cellulose, is a similar material, but made by a different process. It is formed by dissolving the pure α-cellulose in an alkali solution. It is then extruded as a continuous fiber, knitted into gauze, and oxidized. The resultant fabric is thus more uniform in composition as well as the oxidation more closely regulated. Consequently, this results in less variation of the product in terms of absorbability and tissue reaction. Both agents are similar with respect to hemostatic properties.1 Both products were developed in the 1940s, when it was observed that oxidized cellulose facilitated hemostasis. It was first thought that the hemostatic properties were purely due to mechanical effects. It was proposed that the oxidized cellulose acted as a physical matrix that platelets adhered to, thus leading to artificial clot formation. It was later discovered that there was some contribution due to its caustic characteristics. When oxidized cellulose encounters blood, it reacts with it to form a reddish gelatinous mass containing hematin (which accounts for the color change to reddish-black). In contrast to the gelatin sponge, oxidized cellulose relies more on its chemical hemostatic properties than on mechanical properties.22–24 Because of the oxidation of the cellulose, the resultant fabric has a very low pH. It is best used alone. The addition of saline or thrombin can decrease its effectiveness. Thrombin is denatured when it comes into contact with the low pH of the oxidized cellulose, thus destroying its chemical hemostatic properties.25 Additionally, due to its low pH, oxidized cellulose has some bacteriostatic properties, thus denaturing some bacterial proteins and making them more susceptible to antibiotics. Oxidized cellulose has been found to have activity against a wide variety of bacterial pathogens.26 In a comparison of artificially inoculated wounds, oxidized cellulose was found to have a significantly lower rate of infection compared with Gelfoam26 and microfibrillary collagen,27 although a higher rate than in the control population.27 This suggests that the use of oxidized cellulose can partially ameliorate the tendency of foreign bodies to serve as a nidus for infection. It was noted, however, that as the amount of oxidized cellulose increased, the incidence of infection increased as well.26 Thus, despite the antimicrobial properties of oxidized cellulose, it is advisable to use the smallest effective amount if it will be left in the surgical field. Oxidized cellulose tends to cause relatively little tissue reaction. The gelatinous mass that results from application can be removed without causing rebleeding, and its presence rarely causes foreign body reaction.28 The rate of absorption depends on where it is implanted, but studies have demonstrated absorption time in the range of 3 to 6 weeks when implanted on cat cortex.29 Studies have also shown that oxidized cellulose reduces adhesion formation and thus may have some utility in preventing the dura from adhering to cortical surfaces.29 Oxidized cellulose does have some disadvantages, though. First, it can inhibit bone formation. When placed in subperiosteal rib resections, oxidized cellulose was associated with decreased bone formation. This leads to the speculation that its use should be minimized when bony fusion or healing is desired.30 Second, in the product insert, it is advised that oxidized cellulose should not be used to wrap vascular anastomoses, as this can lead to narrowing and stiffening of the wrapped vessels.31 Lastly, due to its tendency to swell after coming in contact with blood, implantation of oxidized cellulose can lead to compressive syndromes. There have been various case reports over time of compressive neuropathies, paresthesias, and even blindness due to compression from swollen oxidized cellulose.32,33 Microfibrillar, or microcrystalline, collagen (Avitene, Davol, Warwick, RI), a water-insoluble, partial hydrochloric acid amino salt of collagen in the form of fibers containing microcrystals, was first reported in 1967.34 The first report of its use as a hemostatic agent was in 1969.35 Avitene contains 1-µm microcrystals of purified bovine dermal collagen. Avitene’s proposed mechanism of action is by facilitating aggregation of platelets. Microcrystalline collagen provides a surface for platelets to aggregate to while coagulation factors are released. The interstices of the collagen prevent subsequent loss of platelets.36 Because of this platelet-dependent clotting activity, the effectiveness of microfibrillar collagen is severely decreased in cases of extreme thrombocytopenia (< 10,000/mL).37 Available in either flour or sheet forms, it should be kept dry before use, as moisture decreases its activity. It is also very hydrophilic, and thus will adhere to surgical gloves. Consequently, it is best handled with sterile forceps.25 As with other hemostatic adjuncts, it is a foreign body, and can serve as a nidus for infection. As a result, the smallest effective amount should be used.27 Microfibrillar collagen tends to float off of briskly bleeding surfaces, such as sinus bleeding.38 When used for those applications, it is recommended that a dry sponge be placed over the bleeding surface after application of microfibrillar collagen. The sponge should be left in place for at least 30 seconds, whereupon it may be removed without causing rebleeding. The excess microfibrillar may then be removed by gentle suction or curettage without risk of causing rebleeding.39 The flour form of microfibrillar collagen can be difficult to handle. To facilitate its use, wafers can be made by filling a syringe with the microfibrillar collagen. The tip is then cut off, whereupon the microfibrillar collagen is extruded, yielding a small disk. Another method that has been used is to place the microfibrillar collagen on a bed of oxidized cellulose. The oxidized cellulose is then folded over the microfibrillar collagen, thus forming a “sandwich.” The resultant sandwich may then be placed in areas that would otherwise be difficult to access.40 Given the small particles of the flour form of microfibrillar collagen, it is particularly useful in stopping cancellous bone bleeding. It has demonstrated superior efficacy when compared with other hemostatic agents, such as thrombin alone or thrombin combined with Gelfoam.41,42 In contrast to oxidized cellulose or bone wax, it does not interfere with bone healing; thus, it is useful particularly when fusion is desired.41,43 When used for bleeding bone, it is recommended that the product be firmly packed into the bone surface followed by application of pressure for 5 to 10 minutes.44 Because microfibrillar collagen is composed of foreign proteins, there is a potential for allergic reactions in individuals. Still, collagen is relatively less antigenic, and generally causes only minor inflammatory reactions.45 Depending on where it is implanted, it is usually assimilated by 7 weeks, leaving little residual material.25 It has been associated with adhesions when implanted intraperitoneally in animals,46 but does not tend to cause narrowing of vascular anastomoses, in contrast to oxidized cellulose.31 Thrombin, in its current relatively pure formation, has been in use since the 1930s.47 It is produced by converting bovine prothrombin to thrombin by thromboplastin in the presence of calcium chloride.48 It is then processed into powder form and supplied in vials ranging from 1,000 to 10,000 NIH units (1 NIH unit is the amount of thrombin required to clot 1 mL of oxalated plasma). Thrombin is also available as a recombinant formulation (Recothrombin®, The Medicines Company, Parsippany, NJ). Because its action is dependent on fibrinogen, thrombin can cause coagulation in whole blood, plasma, or simply a solution of fibrinogen.1 Thrombin can be applied in its powder form directly to bleeding surfaces or can be diluted in sterile saline and applied either as a wash or by spraying. Its most popular use is in combination with a gelatin sponge, where it can be applied directly to bleeding surfaces, synergizing the chemical hemostatic properties of thrombin with the mechanical ones of the gelatin sponge. As mentioned earlier, thrombin should not be used with oxidized cellulose. In addition, it should not be used with microfibrillar collagen, as moistening the microfibrillar collagen destroys its hemostatic properties. Although thrombin causes very fast clot formation, it is easily washed off. Thus, it is best used in cases of mild to moderate bleeding; it is not sufficient on its own to stop brisk, arterial bleeding. In addition, in cases of severe fibrinogen depletion, thrombin is ineffective.1 Given that it is bovine in origin, it can be antigenic in humans; thus, allergic reactions can occur. It should also not be allowed to enter the vascular system, as disseminated intravascular coagulation may occur. In animal studies, thrombin caused increased cerebral edema when used in concentrations similar to human neurosurgical procedures (100–1,000 units/cm3). Consequently, it was concluded that the use of thrombin should be minimized to those situations in which other hemostatic methods such as bipolar cautery are insufficient to stop bleeding.49 Bone wax has been in use since the 1800s. Sir Victor Horsley, in 1892, was the first surgeon to use it in a neurosurgery application. With his seminal written contribution to the then-nascent field of neurosurgery, the application of bees’ wax to stop bone bleeding became popularly known as “Horsley’s wax.”50 The formulation currently in use, known as beeswax, softened with 12% isopropyl palmitate with or without up to 30% paraffin wax, is remarkably similar to the wax that was formulated by Horsley. Because of its ease of use and effectiveness, it remains a popular method of achieving bone hemostasis.50 The hemostatic properties of bone wax are purely mechanical. It functions by tamponading bleeding cancellous channels. Because it is insoluble, it is not metabolized or absorbed by the body, thus remaining indefinitely at the site of application.51 Despite its widespread use, it is not without drawbacks. It can potentially cause chronic inflammation52 and foreign body granuloma formation, lower the bacterial clearance from cancellous bone,53 and serve as a nidus for infection.54 In addition, it can exert local mass effect, and can compress adjacent neural or vascular structures, leading to pain, weakness, blindness, or occlusion of dural sinuses.55,56 For this reason, judicious use of bone wax is recommended. One of the most serious drawbacks is the tendency of bone wax to interfere with bone healing.57 It has been observed to interfere with bone healing in sternotomy operations.58 In addition, because it is physiologically inert, it may serve as a barrier to bone healing for an indefinite period of time. Because of this, the use of bone wax should be avoided in cases where bone fusion is desired, as in the case of spinal fusions. Given the drawbacks of beeswax-based bone wax, alternative formulations have been developed. Ostene (Ceremed, Los Angeles, CA), which is composed of water-soluble alkylene oxide block copolymers, has been demonstrated in rat models to be quickly absorbed as well as not to interfere with bone healing. This product is commercially available, but given a relatively short track record, it is not possible to comment on any potential long-term disadvantages.51 Fibrin glue (Tisseel, Baxter, Deerfield, IL; Evicel, Ethicon, New Brunswick, NJ) is composed primarily of two components: human fibrinogen and human thrombin.59 The fibrinogen component is delivered as a “sealer protein,” which is composed of fibrinogen with aprotinin to prevent fibrinolysis. When the two components are combined, it mimics the final stage of the human coagulation cascade.60 Upon mixing, the soluble fibrinogen is converted by thrombin into fibrin, thus resulting in a rubbery mass that adheres to tissues, thereby achieving hemostasis. In addition, it also has some sealing characteristics; thus, it has found widespread usage as an adjunct to prevent cerebrospinal fluid (CSF) leakage.61 The only on-label indications for fibrin glue are hemostasis in the setting of cardiopulmonary bypass and splenic injuries, and as a sealant to prevent leakage from colonic anastomoses following reversal of temporary colostomies. It is explicitly stated in the product insert that fibrin glue has not been evaluated for safety in neurosurgical procedures. Still, despite these statements, fibrin glue has become a popular hemostatic adjunct and sealant in neurosurgical procedures. Also, because fibrin glue does not rely on host factors for hemostasis, it can effectively be used even in heparinized patients.62 Despite its off-label usage, it is widely reported as a hemostatic agent in neurosurgical procedures, including stopping epidural, cortical, and dural sinus bleeding. It has also been used in peripheral nerve repairs, and has been found to be comparable to suture repair in various animal studies.63 Fibrin glue can be delivered either by dual-barrel syringe or spray. In either case, after the fibrin glue has been applied, it should be fixed or held in place for 3 to 5 minutes to ensure adherence to tissues. If applied by spray, care should be taken not to hold the device too close to the tissue, as there have been cases of air embolisms.64 In addition, only a thin layer should be applied. Excess clot thickness can delay the natural wound healing process. Oxidized cellulose may cause denaturation of the proteins in fibrin glue, decreasing its efficacy.61 Because fibrin glue is derived from human pooled plasma, there exists a theoretical risk of disease transmission. In addition, it also contains synthetic aprotinin, for which cases of anaphylaxis have been reported.65,66 Monopolar (or unipolar) cautery was developed in 1920s by William T. Bovie, a physicist at the Massachusetts Institute of Technology. In cautery’s earliest incarnation, Bovie found that excellent coagulation could be produced by a spark-gap generator producing irregular damped waveforms. This generator was also able to produce a synchronous sinusoidal waveform, thus resulting in cutting.67 First brought into the surgical arena by Harvey Cushing, cautery revolutionized the field of surgery, and brain surgery in particular. It enabled Cushing to perform tumor removal in areas that had been previously inaccessible due to uncontrollable bleeding.4 Over the years, modifications have been made to the original monopolar cautery unit, but the mechanism of action remains the same. The cutting or coagulation action of the monopolar unit is delivered by the active electrode, which provides a high current per cubic millimeter due to its small size. Accordingly, the return path is provided a much larger ground plate. The coagulating waveform is arrhythmic and random, thus eliminating molecular resonance. This lack of resonance with tissue results in coagulation rather than cutting. The current then travels through the tissues to the ground plate, preferentially traveling through higher conductivity structures such as blood vessels and nerves. This results in two effects. First, there is significant current and heat generation 1 to 2 cm away from the electrode, making it unsuitable for microsurgery. In addition, because the current preferentially travels through nerves and blood vessels, more damage may occur to these structures as well as inducing nerve or muscle activation.67 If the waveform is sinusoidal and regular, as opposed to arrhythmic, a cutting current will be generated. In the range of 0.5 to 3 MHz, it induces molecular resonance, thus causing division of tissues while minimizing stimulation of muscle and nerve tissues. If the power is decreased to too low a level, coagulation rather than cutting will occur. This is an inherently inferior technique for coagulation, as partial contact will cause cutting due to higher density of current delivery. As with coagulation settings, cutting current causes heat generation 1 to 2 cm distant from the point of contact and will travel preferentially along nerves and blood vessels, causing increased damage along those pathways. In either case, if the electrode is held close to, but not touching, tissue, sparking will occur. This fulguration will result in destruction of tissues.68 Due to the need for fine control of cauterization, bipolar cautery units were developed. The first bipolar cautery system was devised by Greenwood by connecting one side of forceps to the electrical current and the other to the ground plate. The power setting was also accordingly decreased, as the current spread was significantly decreased compared with monopolar cautery. This system was not isolated, however. That is, if only the electric current side of the forceps came in contact in tissues, the current could spread through the patient via any grounding contact on the patient’s body. Effectively speaking, this was of minor concern, as the power utilized was much less compared with monopolar cautery, thus limiting the possibility of indiscriminate tissue damage. Despite the effectiveness and utility of this system, it was not popularized and was used only by Greenwood himself.69 With advances in solid-state electronics, Malis developed the bipolar cautery unit that is still in use today. As opposed to spark generators, which were used previously, solid-state generators were capable of producing finely controlled synchronous or asynchronous waves that could either cut or coagulate as needed with fine control. This system was also isolated; thus, no current would spread if only one side of the forceps came in contact with the patient. As with monopolar cautery, synchronous waves result in molecular resonance, thus leading to cutting. If the wave is asynchronous, no resonance occurs, thus resulting in coagulation.67 The current passes predominantly between the forceps with very little leakage through the patient. As a result, the power needed as compared with monopolar cautery is much reduced. In addition, because of the relative lack of current spread, bipolar cautery is appropriate for use in microsurgery.70 The closer the tips of the forceps are held together, the more focused the current delivery will be with less current leakage. It is thus advised to hold the tips as close together as possible for maximal utility. Irrigation is generally recommended, as this prevents charring or sticking.71 The modern bipolar unit has an irrigation unit integrated into the bipolar forceps. The forceps are also bayoneted to prevent obstruction of view when used in microsurgery. Over time, the tips of the bipolar forceps become rough or pitted due to electrolysis. Uneven contact of the tips with tissue can result in sticking of tissues to the forceps. Current bipolar forceps tips are composed of a metallic alloy that is resistant to pitting or roughening due to electrolysis. Accordingly, it is recommended that the tips never be cleaned with an abrasive “scratch” pad. Instead, a clean wet sponge is as effective and will avoid damaging the forceps.67 Laser, an acronym for light amplification by stimulated emission of radiation, has attained some utility in the neurosurgical armamentarium. First used in the 1960s, lasers became increasingly popular in the 1980s. However, due to their perceived inefficiency, lack of accuracy (compared with bipolar cautery), and nonergonomic characteristics, its use fell out of favor. Today, although lasers are still being used in the neurosurgical arena, its use is not nearly as widespread as may have been predicted when they were first developed and popularized.72 Lasers are typically used in three situations in surgery: photocoagulation, photovaporization, and photoactivation. Which of these three actions will take place is dependent on the properties of the laser and the tissue itself. Laser properties include power output, wavelength, beam density, and time of exposure. The properties of tissue include the absorption coefficient, the extinction length, and the presence of light-absorbing chromophores.73 Today, there are three types of laser that are commonly used in neurosurgery. First, the CO2 laser, with a wavelength of 10.6 µm, can produce coagulation, cutting or vaporization.74 The argon laser, with a shorter wavelength of 488 to 516 nm, is scattered more widely in tissue. As a result, the energy delivered is dispersed more widely as heat causing a broader zone of coagulation.73 Lastly, the neodymium: yttrium-aluminum-garnet (Nd:YAG) laser (wavelength 1060–1340 nm) spreads even more widely through tissues, causing slow, deep heating of tissues. This results in a wide area of coagulation.75 The Nd:YAG laser was also investigated in vascular anastomoses in the 1980s, though its relatively inexact heating effects limited its utility near eloquent tissues.76 The first lasers delivered high-energy pulses. When used in the first animal experiments, they were found to cause rapid heating and expansion of mice brains, causing instantaneous death due to herniation.77,78 When the power was lowered, continuously powered lasers were developed, which enable slower, more finely controlled heating to be delivered to tissues, resulting in coagulation or cutting with finer accuracy. It was these experiments that described the characteristic effects of laser vaporization, a vaporized core surrounded by a rim of desiccated tissue. This, in turn, was surrounded by a zone of edematous tissue caused by heat.79,80 Following these experiments, lasers were then used in tumor extirpation. The concept of surgery without requiring actual contact with tissues was quite attractive. It was demonstrated to be effective in a variety of situations including gliomas, fibrous tumors such as meningiomas and peripheral nerve tumors, and scar tissue as in the case of tethered cords.72 Lasers were also investigated in endoscopic techniques, but were found to have limited utility due to loss of energy when refracted through the fiberoptic cables available at the time.72 Although certainly efficacious, lasers were often found to be slower than other available technologies, including bipolar cautery and ultrasonic aspiration. In addition, due to the relatively large size and cumbersome ergonomics of the equipment, laser use fell out of favor.81 Recently, a newer CO2 laser has been developed by OmniGuide (Cambridge, MA). It consists of a flexible, hollow fiber lined with an omnidirectional mirror, ameliorating some of the disadvantages of previous CO2 lasers. Because of its low profile, it can be used in microsurgical situations.82 It remains to be seen if lasers will gain widespread usage in neurosurgery. KEY POINTS • Hemostatic agents are categorized as chemical, mechanical, or thermal. • All hemostatic agents are foreign bodies, and can serve as a nidus for infection. The minimum necessary amount should be used. • Hemostatic agents are derived from animal proteins, and thus can be antigenic. • Hypersensitivity reactions have been seen with thrombin and fibrin glue. • Gelatin sponge and oxidized cellulose swell and can cause compression of adjacent structures. • Bone wax and oxidized cellulose can retard bone formation, limiting its use where bone healing is desired. • Bipolar cautery delivers finely applied current and is suitable for microsurgery, in contrast to monopolar cautery. • Lasers, although effective, have not found widespread use in neurosurgery.
Intraoperative Non-Hematologic Adjuvant Methods for Preventing Blood Loss
General Historic Overview
Absorbable Gelatin Sponge (Gelfoam, Surgifoam)
Oxidized Cellulose (Oxicel) and Oxidized Regenerated Cellulose (Surgicel)
Microfibrillar Collagen (Avitene)
Thrombin
Bone Wax
Fibrin Glue
Cautery
Monopolar Cautery
Bipolar Cautery
Laser
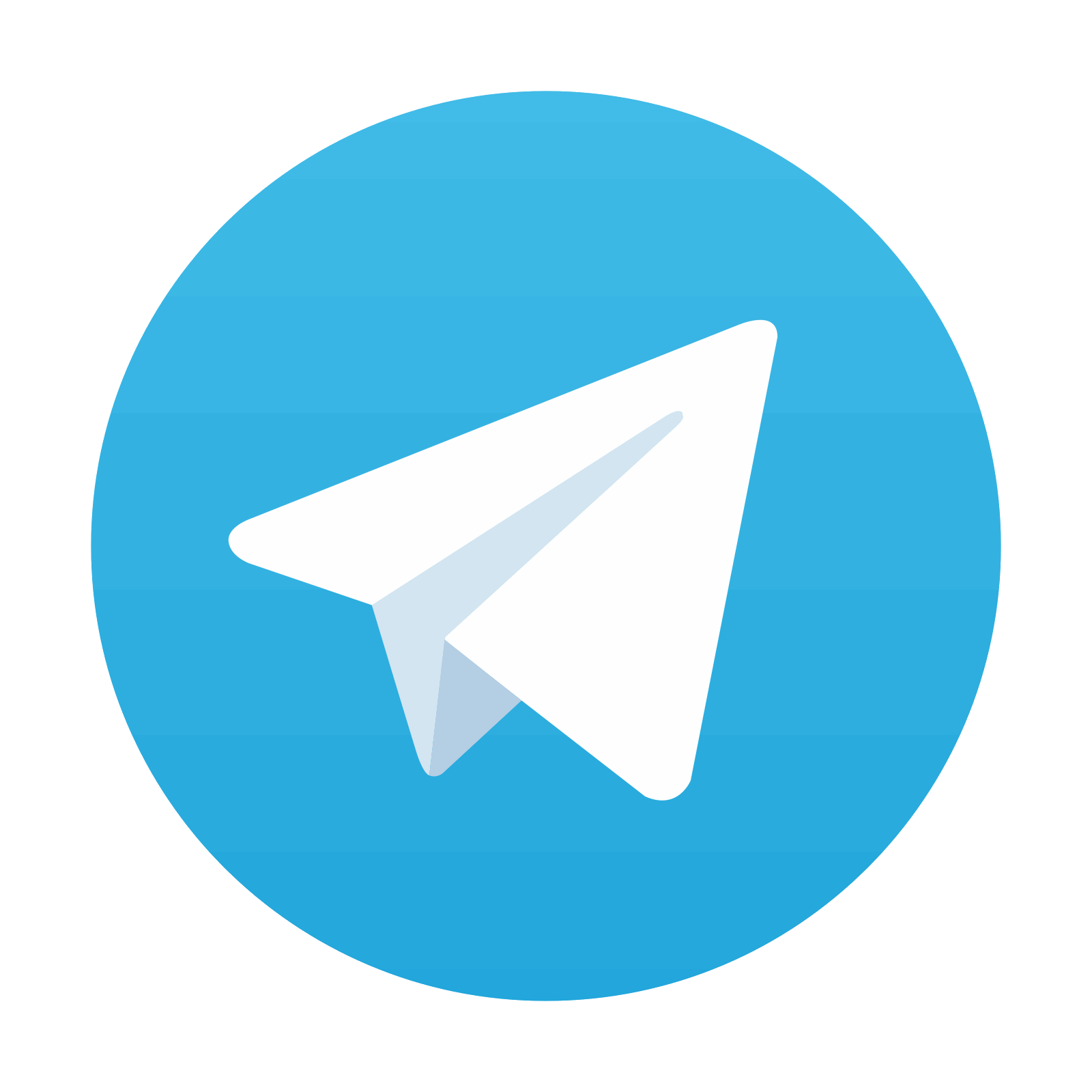