Fig. 11.1
Coronally oriented head ultrasound scan . Note the blood in the left germinal matrix region, within the frontal horn of the left lateral ventricle and within the left fronto-parietal white matter
This case raises several important questions:
- 1.
Why did this premature infant develop a significant hemorrhage?
- 2.
Could the bleeding have been prevented?
- 3.
How should the infant be managed and evaluated over the next several days to weeks?
Background
The overall incidence of PV-IVH has declined over the past three decades; however the occurrence of severe hemorrhage remains substantial, particularly in the tiniest of the very-low-birth-weight population [1–3] (Table 11.1). Thus, approximately 26 % of infants of a gestational age between 23 and 25 weeks and 12 % between 26 and 27 weeks still develop the most severe forms of hemorrhage [3]. This is highly relevant because as survival of infants born at the cutting edge of viability continues to increase, severe hemorrhage rates are likely to increase, and with them long-term neurodevelopmental deficits.
Table 11.1
Intraventricular hemorrhage
Gestational age | 23 weeks | 24 weeks | 25 weeks | 26 weeks | 27 weeks | 28 weeks |
---|---|---|---|---|---|---|
N = 496 | N = 1223 | N = 1426 | N = 1530 | N = 1811 | N = 1967 | |
Grade of IVH | ||||||
Grade 3 | 15 % | 9 % | 8 % | 5 % | 4 % | 6 % |
Grade 4 | 21 % | 14 % | 13 % | 7 % | 5 % | 9 % |
Neuropathology
The primary lesion is bleeding from vessels within the periventricular subependymal germinal matrix located between the caudate nucleus and thalamus at the level of the foramen of Monro [2] (Fig. 11.2). The matrix is a transient gelatinous region that provides poor support for a large, immature network of blood vessels primarily supplied by Heubner’s artery, a branch of the anterior cerebral artery [4]. The venous drainage also appears to be important with regard to the risk for bleeding. Thus the drainage includes the terminal, choroidal, and thalamo-striate veins that lead to the internal cerebral vein. The blood flow then makes a “U-turn” in the subependymal region at the level of the foramen of Monro, where most of hemorrhage originates [2]. This creates the potential for obstruction of the venous drainage resulting in venous distention with obstruction of the terminal and medullary veins, with subsequent rupture. The primary vascular source of PV-IVH has not been clearly established. Thus the initial descriptions suggested a venous origin of hemorrhage [2] while subsequent pathologic studies described it as emanating from capillary or arterial vessels [4]. Based on studies conducted over the years it is most likely, that PV-IVH results from forces acting upon both the arterial and venous circulations [1, 2].


Fig. 11.2
Pathologic specimen from a premature infant demonstrating blood within the germinal matric, left lateral ventricle, third and fourth ventricles
Importance of the Germinal Matrix
The germinal matrix is an active site of active cellular proliferation, and is a source of both neuronal precursors early in gestation as well as glial elements that become oligodendroglia and astrocytes in the third trimester [2]. The matrix involutes after approximately 32 weeks and by term gestation, this region is essentially absent. Destruction of the matrix as a result of hemorrhage may result in impairment of myelination, brain growth, and subsequent cortical development [2].
Grading the Hemorrhage
The hemorrhage may be confined to the germinal matrix region (grade 1 IVH) or it may extend and rupture into the adjacent ventricular system (grade II or III IVH) depending on the extent of blood, or may extend into the adjacent white matter (termed a grade IV IVH or intraparenchymal echogenicity (IPE)) [2, 5, 6] (Fig. 11.1). The latter lesion, which is often unilateral, represents an area of hemorrhagic necrosis of varying size within periventricular white matter, dorsal and lateral to the external angle of the lateral ventricle [2, 6, 7].
Pathogenesis of IVH
In general terms, three factors appear to be central to the genesis of hemorrhage. The first relates to inherent vulnerability of the germinal matrix, with immature vessels and poorly supportive gelatinous matrix as described above [2]. The second relates to the concept of a pressure passive circulation often referred to as loss of autoregulation, and the third relates to perturbations in cerebral blood which are common in the sick premature infant.
Cerebral Autoregulation and Pressure-Passive Circulation
Autoregulation is defined as a state whereby CBF remains constant as cerebral perfusion pressure varies over a certain range [8]. The mean arterial pressure at which CBF decreases during hypotension is termed the lower limit, and the arterial pressure at which CBF increases is called the upper limit of autoregulation. In newborn animals, the range of blood pressures at which CBF remains constant is lower than in adult animals [9, 10]. More importantly, in fetal lambs, the resting blood pressure is only slightly higher than the lower limit of the autoregulatory curve [10]. If a similar situation exists in newborn infants, moderate hypotension could result in reduced CBF and create the potential for ischemic brain injury. Evidence has indicated that the autoregulatory response remains intact in human neonates with mild asphyxiation or who have minimal respiratory distress [2]. By contrast seminal data of Lou and colleagues [11] derived from 19 newborn infants with varying degrees of respiratory distress studied in the first hours of life were the first to indicate that CBF varied considerably with spontaneous variations in blood pressure, suggesting that autoregulation was, in fact, lacking. Subsequent studies over the subsequent years utilizing different methods to assess CBF or cerebral blood flow velocity including Doppler, near-infrared spectroscopy, and xenon have supported the concept of a pressure passive cerebral circulation in the sick infant; that is, CBF varies directly with changes in systemic blood pressure [12–15].
To summarize, although intact cerebral autoregulation has been documented in the premature infant, it appears to function within a limited blood pressure range, and is likely to be absent particularly in the sick hypotensive preterm infant [12–15]. This clinical state places the developing brain at great risk for injury during times of hypotension and or elevated blood pressures .
Intravascular Perturbations
Evidence has pointed to a critical role for intravascular factors and specifically perturbations in ABP as a major mechanism of capillary rupture and hemorrhage. First, the cerebral circulation of the sick infant was considered pressure passive (see above) [12–15]. Second, experimental studies indicated that germinal matrix hemorrhage could be produced by systemic hypertension with or without prior hypotension [16, 17]. Third, infants with lower mean ABP in the first postnatal days and infants who received rapid volume expansion to correct hypotension were more likely to develop IVH [18–20].
In the original pathologic description of IVH, elevations in venous pressure were proposed as an important source of hemorrhage, based in part on the previously described venous drainage of germinal matrix and white matter [2]. Indeed simultaneous increases in venous pressure were observed in infants who exhibit variability in ABP, such as with respiratory distress syndrome (RDS) and associated complications, e.g., pneumothorax or pulmonary interstitial emphysema, or with mechanical or high-frequency ventilation [21–23].
To summarize, the cumulative data suggested that there is a significant contribution from both arterial and venous perturbations to the development of IVH. Over the years, studies have indicated that these intravascular responses can be modulated by additional factors, i.e., inflammation associated with chorioamnionitis (negative influence) [24] or the administration of glucocorticoids to the mother (positive influence) [25, 26].
Periventricular White Matter Injury (WMI) Associated with IVH
The cause of the WMI associated with hemorrhage still remains unclear. The original hypothesis was that the intraparenchymal lesion represented an “extension” of hemorrhage from the germinal matrix or lateral ventricle into previously normal periventricular white matter. However subsequent neuropathologic data indicate that the intraparenchymal lesion represents an area of hemorrhagic necrosis [2, 5, 7]. The WMI appears to be closely linked to the adjacent hemorrhage and two potential pathways have been proposed to explain this intricate relationship. The first suggests a direct relationship to the hemorrhage based on several clinical observations. First, the WMI is always noted concurrent with or following a large GM and/or IVH, and is rarely if ever observed prior to the hemorrhage, and second the WMI is always observed ipsilateral to the side of the larger hemorrhage with bilateral bleeding [1, 2]. This consistent relationship between the GM and WMI may in part be explained by the venous drainage of the deep white matter as described previously. A second explanation is that the WMI evolves de novo and the IVH and WMI occur concurrently. Since both the GM and the periventricular white matter are border zone regions, the risk for ischemic injury increases during periods of systemic hypotension, particularly in the face of a pressure passive cerebral circulation [1, 2]. Hemorrhage in these regions may then occur as a secondary phenomenon i.e. reperfusion injury. In support of this theory is the fairly consistent observation of the simultaneous detection of PV-IVH and WMI by cranial ultrasound imaging. Moreover elevated hypoxanthine and uric acid levels, perhaps as markers of reperfusion injury, have been observed on the first postnatal day in infants who subsequently developed WMI [27, 28].
Case Continued
The infant continued to exhibit moderate respiratory distress and developed a left sided pneumothorax requiring chest tube placement. The arterial blood gases showed moderate hypercarbia (pCO2 55 to 58 mmHg and mild acidosis—pH 7.20–7.26). The hematocrit continued to fall to 22 % on DOL 4. A repeat cranial sonogram revealed more prominent “echoes” within the left fronto-parietal white matter, and the lateral ventricles were more distended as a result of increased hemorrhage. Serial sonograms over the first 6 weeks demonstrated progressive enlargement of the lateral, third, and fourth ventricles (Fig. 11.3).


Fig. 11.3
Coronal sections demonstrating the large left-sided intraventricular hemorrhage with involvement of the ipsilateral white matter (a); followed by cystic evolution and dilation of the lateral ventricles (b) and further dilation (c)
Question
- 1.
Why did the hemorrhage progress?
- 2.
What is the cause of the progressive ventriculomegaly?
Linking Systemic and Cerebral Vascular Perturbations in Infants with Respiratory Distress Syndrome (RDS) to PV-IVH
Perturbations on the Arterial Side
Numerous studies established an association between RDS its complications, i.e., pneumothorax and IVH [22, 23]. The mechanisms linking the two conditions appear to be mediated via systemic perturbations including fluctuations or rapid increases in blood pressure in large part related to respiratory mechanics often precipitated by the infant breathing out of synchrony with the ventilator and/or in associations with complications such as a pneumothorax [23, 29]. Simultaneous perturbations on the venous side were noted with resultant increases in venous pressure [21]. In a series of studies conducted in sick intubated and ventilated premature infants, these striking systemic changes with parallel changes in the cerebral circulation were associated with the subsequent development of IVH, and could be prevented by eliminating the fluctuations [23, 30]. Importantly over time, the advent of newer ventilators that work in tandem with the infants own respiratory efforts coupled with the antenatal use of steroids perhaps by stabilizing the germinal matrix vessels has made this association less prominent [25, 26]. However in a susceptible infant (no exposure to antenatal steroids) close attention to fluctuations in blood pressure should be a priority, and when present should be minimized .
Perturbations in Venous Pressure
Fluctuations in venous pressure simultaneous with the ABP perturbations in preterm infants with RDS who developed PV-IVH have also been observed [21]. The fluctuations or increases in venous pressure can be exacerbated by the use of higher mean airway pressures such as may be observed with high-frequency oscillatory ventilation, or pneumothorax. The potential importance of these venous fluctuations in the genesis of hemorrhage is intertwined with the venous drainage of the deep white matter and in particular the peculiar U-shaped turn ending at the terminal vein in the region of the germinal matrix. Increases in venous pressure in this region raise the likelihood of venous distension and resultant obstruction of the terminal and medullary veins resulting in a venous infarction.
Hypercarbia and Intraventricular Hemorrhage
The potential contribution of hypercarbia has only in recent years been clearly delineated in premature infants [31]. Thus in a study undertaken in the first week of life in VLBW infants, increasing PaCO2 resulted in an increase in CBF and progressive impairment of cerebral autoregulation [31]. Hypercapnia defined by a maximum PaCO2 recorded during the first 3 days of life has also been associated with severe IVH. In a retrospective cohort study of 574 VLBW infants, as the maximum PaCO2 increased from 40 to 100 mmHg, the probability of severe IVH increased from 8 to 21 % [32]. In a single-center retrospective review of 849 infants weighing <1250 g extremes in PaCO2, both low and high, as well as fluctuations in PaCO2 during the first 4 days of life increased the risk of severe IVH [33]. These cumulative observations indicate that extremes in PaCO2 should be avoided during the period in which infants are at high risk of IVH. This is highly relevant since permissive hypercapnia has been advocated as a ventilator strategy to minimize barotrauma to the lungs of preterm infants, and thus prevent the evolution to chronic lung disease [34] (Fig. 11.4).


Fig. 11.4
Schematic representation depicting the association of vascular and venous perturbations in an infant with respiratory distress syndrome and the relationship to subsequent intraventricular hemorrhage
Surfactant Administration and PV-IVH
The introduction of surfactant administration to reduce the severity of RDS in the late 1980s was the most important development in the management of sick premature infants. Since surfactant reduced the severity of RDS as well as the rate of pneumothorax, it was postulated that it would also lead to a decrease in the rates of IVH. However, this was not borne out in the many surfactant trials [35]. This lack of effect has been attributed to the impact of surfactant administration on fluctuations of CBF in sick preterm infants who have pressure passive circulation and the rapid changes of PaCO2 and PaO2 that may subsequently lead to brain injury [36, 37].
Post-hemorrhagic Hydrocephalus (PHH)
PHH complicates approximately 40–50 % of severe IVH [2]. The progression of PHH may be rapid, i.e., days, and in such cases, it is usually associated with raised intracranial pressure . The obstruction under such circumstances appears to be secondary to an impairment of cerebrospinal fluid absorption caused by particulate clot usually demonstrated by ultrasound scan. This may be at the foramen of Monroe or the aqueduct of Sylvius. Treatment is usually immediate, via external drainage above the site of obstruction. More commonly, a communicating hydrocephalus evolves from 1 to 4 weeks following the diagnosis of hemorrhage. The hydrocephalus is commonly secondary to an obliterative arachnoiditis distal to the outflow of the fourth ventricle. The clinical criteria of evolving hydrocephalus, i.e., full anterior fontanel and rapid head growth, do not appear for days or weeks after ventricular dilation has already been present. Possible explanations for this discrepancy include the relative excess of water in the centrum semiovale and the relatively large subarachnoid space. Thus, serial scans are critical to follow for the evolution of PHH.
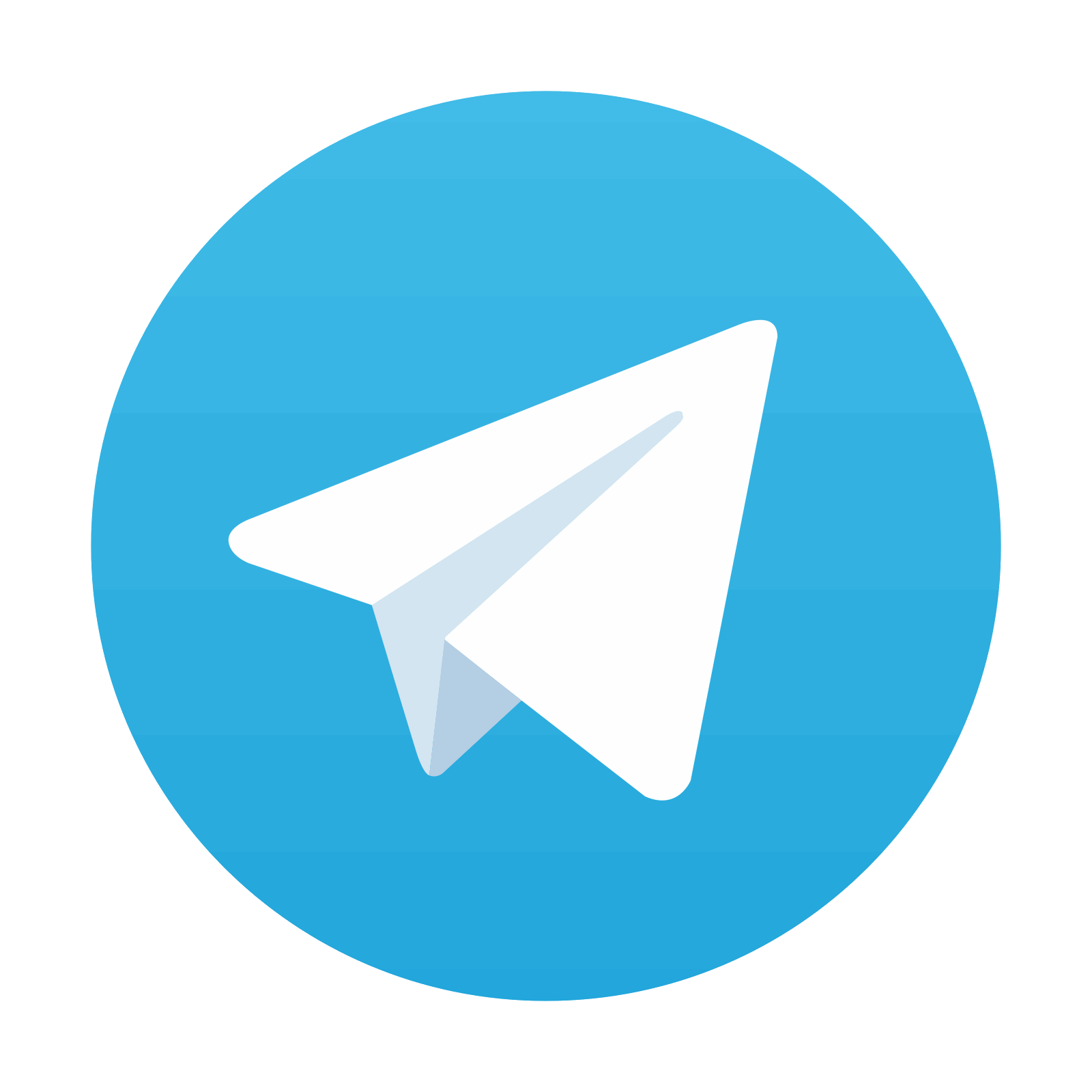
Stay updated, free articles. Join our Telegram channel

Full access? Get Clinical Tree
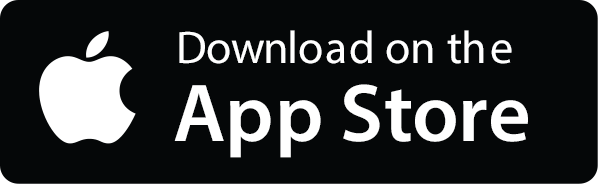
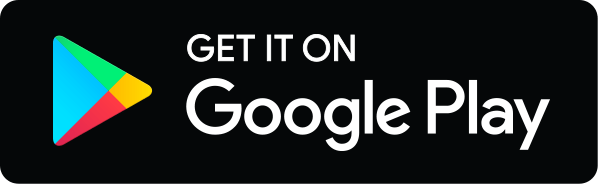