and Gordon N. Dutton2
(1)
Department of Psychology, LMU Munich, München, Germany
(2)
Department of Visual Science, Glasgow Caledonian University, Glasgow, UK
Perception provides the means to access and engage with our physical and social environment and to learn through this process. It is essential for the acquisition of knowledge through short- and long-term experiences and for the guidance and control of our behaviour and movements. Perception comprises all activities involved in the recording, processing and coding of information, including cognitive (e.g. attention, memory, planning and supervision), motor (e.g. eye, hand, limb and body movements) and emotional components (e.g. affective evaluation). The neurobiological foundation of the range of perceptual functions and capacities comprises the peripheral (sense organs) and central nervous system components that are specialised to process, code and evaluate the information recorded and to make it accessible for further mental and behavioural activities. The integration of this myriad data set within respective brain structures constitutes a crucial prerequisite for temporally and spatially coherent perception, action and experience (Mather 2006; Goldstein 2010).
The different perceptual systems (vision, audition, taste, smell, the somatosensory system and sense of balance) possess similar neurobiological principles of construction. Stimuli are registered in the sensory organs, are translated into reliable neuronal codes and are transmitted to the central structures serving the respective sensory systems. Information processing and coding predominantly occurs in networked elements of the cerebral cortex. This guarantees detection, localisation and discrimination as well as the more complex capacities of identification, recognition and association, as well as storage of visual stimuli. Functional neuronal units in sensory cortical areas are specialised in processing and coding for specific pieces of sensory information. For example, the visual cortex possesses different areas for the analysis of colour, form, movement and spatial information (e.g. position, distance, direction) and for objects, faces, places and text material (letters, words and syntax). The auditory cortex contains areas specialised in processing and coding of sounds, pitch, music and speech. In the somatosensory cortex, various qualities of tactile, proprioceptive and kinaesthetic stimuli are processed and coded in separate areas (for comprehensive reviews, see Goldstein 2010; Yantis 2013). This so-called functional specialisation of the cerebral cortex enables the brain to process information (e.g. object features) in parallel and serial modes in one or in several sensory modalities, which are then integrated in consecutive steps to a uni- or multimodal percept. The final goal of visual development is to establish ‘primary’ visual perception, which integrates the active observer with subjective visual experience, i.e. perception is linked to a first-person perspective that mediates between conflicting visual information, on the one hand, and expectation and experience, on the other (Pollen 2011).
Perception does not operate in isolation, but is embedded in a network of mental functions. Figure 1.1 shows the various components of and interactions between the various components of this network. Attention, memory, executive function, motivation, mood and emotions as well as language and action have an impact on perception but are also reciprocally influenced by perception. These mutual interactions mean that disorders of perception are not only caused by direct disturbance of a specific perceptual system (so-called primary perceptual disorders) but can also result as a consequence of dysfunction of the other functional systems, because processes of stimulus registration, processing and encoding may be indirectly affected (so-called secondary disorders) and thereby impair perceptual ability and capacity. This concept applies particularly to the development of perceptual functions and capacities in early childhood. The normal development of perception relies crucially upon cognitive, motor, motivational and emotional components reaching the requisite milestones. A child will not explore the environment in the absence of adequate curiosity, nor will the child focus interest on and fixate a stimulus for detailed processing, identification and storage, without adequate attention. The child cannot gain experience with guidance and control of motor response and behaviour, nor develop and acquire action routines without perceptual learning and the requisite memory capacity. Moreover, perception is fundamental for the development of attention, memory, executive function, sensorimotor activities, language, motivation and emotion (see also Chaps. 3 and 5).


Fig. 1.1
A framework of mental functioning, where visual perception is embedded and interacting reciprocally
The brain is built of complex and highly developed networks, comprising many functional systems (see Table 1.1). Learning to interact with the physical and social world is mediated by experience, progressive optimisation of these systems/subsystems and their interactions and underpins successful development. Any disorder of brain development, either due to unfavourable congenital factors or to acquired impairment of normally developing or developed brain, can affect capacities in the various functional domains, either selectively or in combination, and disrupt or delay their further development.
Table 1.1
Functional systems and associated functions/capacities (A) and associated brain structures (B): a simplified overview
A. Functional systems | |
Perceptual systems | |
Vision: visual field, acuity and contras vision, form and shape perception, colour vision and visual space perception, including stereopsis, movement vision, visual recognition (objects, faces, letters, paths and places, etc.) | |
Audition: perception of sounds, voice, music and speech; spatial audition | |
Somatosensory perception: touch, proprioception, kinaesthesis; body perception | |
Olfactory perception: detection, discrimination and identification/recognition of smell stimuli | |
Gustatory perception: detection, discrimination and identification/recognition of gustatory stimuli | |
Social perception: identification/recognition of social stimuli in the various perceptual domains, particularly in vision and audition | |
Cognitive systems | |
Attention | |
Intensity: level and duration of maintenance of alertness; mental processing speed | |
Selectivity: focused attention (concentration), divided attention, attentional flexibility (attention regulation) | |
Spatial attention: shifts of attention in space; field of attention | |
Memory | |
Time dimension: short-term memory, working memory, long-term memory; prospective memory | |
Content dimension: episodic, semantic and procedural memory | |
Executive function | |
Cognition: planning, problem solving, flexibility, (self-)monitoring, multitasking | |
Behaviour: response inhibition, flexibility and control of (internally and externally triggered/guided) behaviour | |
Speech and language Understanding and producing verbal language; reading and writing | |
Emotional and motivational systems | |
Emotions: feelings and mood and appropriate regulation | |
Motivation: activation and modulation of mental processes and behaviour and appropriate regulation | |
Sensorimotor or motor systems | |
Gross motor functions: body posture and control of body movements, including movements of legs (e.g. walking) and arm movements | |
Fine motor functions: hand movements (grasping and manipulating of objects) | |
Articulation: production of speech | |
Oculomotor functions: vergence, accommodation; saccades, pursuit movements, fixation | |
B. Brain structures | |
Occipital lobe | Vision |
Temporal lobe | Vision (visual identification and visual memory); language perception; verbal memory |
Parietal lobe | Space (visual and multimodal); visuomotor guidance (gaze, hand movements); somatosensory perception, body representation |
Frontal lobe | Motor activities (planning, execution and control; praxis) |
Executive cognitive functions | |
Regulation of motivation and emotional responses/mood adaptation processes (e.g. environment-intention) | |
Limbic system | Motivation, mood, emotional behaviour |
Memory | |
Emotional evaluation of internal and external stimuli | |
Midbrain, brainstem | Alertness, attention (intensity) |
Cerebellum | Motor activities (including cognitive adaption processes of motor responses) |
The development of the central nervous system (CNS) takes place through generation of neurons (neurogenesis) to differentiation of the cerebral cortex, in relatively regulated steps (Sanes et al. 2006). In early childhood, the capacity of the CNS to adapt to changes of internal (i.e. processes and alterations in the brain) or external conditions (i.e. stimuli in the environment) is maximal; this facility is referred to as developmental (neuro)plasticity (Johnson 2001). Selection, optimisation and compensation, and their intermediary processes, have been considered to be fundamental components of the ‘architecture’ of human ontogeny, which is incomplete and thus allows flexible adaptation to internal and external changes to achieve and maintain a ‘positive balance between gains and losses’ throughout the life span (Baltes 1997, p. 366). To some extent, the child’s brain possesses the potential of self-regulation and autonomy as it builds its intrinsic structure (Ryan et al. 1997) on which functional systems are based, in particular those concerned with coding and storing information, i.e. with gaining and learning from perceptual experience. As Black (1998, p. 168) has emphasised, many regions of the brain are ‘responsive to experience’ but differ with respect to their functional specialisation and the timing of their development. In addition, plasticity is ‘typically embedded in a developmental programme’ and requires appropriate timing and quality of sensory input. Experience-dependent plasticity in the visual cortex is at the same time also practice-dependent plasticity, which manifests particularly in so-called sensitive periods. During these periods, neural circuitries are formed, which at the physiological level are associated with receptor activation and inhibition. Transmitters (e.g. NMDA and GABA) play a crucial role in determining onset and closure of the time window of the sensitive period (Murphy et al. 2005). As a consequence, early visual deprivation, for example, arising from early bilateral congenital cataracts, can limit plasticity for ‘recovery’ of visual acuity and contrast sensitivity, and the age of treatment plays an important role, with early surgery affording the best outcome (Gelbart et al. 1982; Maurer et al. 2006).
An enriched, age-appropriate complex environment triggers and accelerates the morphological and functional maturation of the visual system, with maternal behaviour (or that of the first person of reference) representing and acting as a fundamental ‘mediator’ (van Praag et al. 2000; Baroncelli et al. 2010). Visual experience is also necessary for the maturation of multisensory neurons and the integration of multimodal spatial information, as well as the development of spatial cognition (Pasqualotto and Proulx 2012). Systematic and repeated perceptual learning results in specific and lasting improvements in lower (pattern orientation; Jehee et al. 2012) and higher (face and texture processing; Hussain et al. 2011) visual as well as cognitive capacities (e.g. working memory; Jolles et al. 2012) not only in the adult but also in the young child. However, changes in neural circuitry can also occur after sensitive periods have ended and can, to a lesser degree, influence spatial and temporal integration modes in the visual cortex and contribute to further maturation of visual functions (Murphy et al. 2005). The final outcome of developmental regulation of associative learning in perception and cognition may be understood as a ‘balance of plasticity and stability that is optimal for information processing and storage’ (Dumas 2005, p. 189). Interestingly, periods of maximal learning capacity in brain development correspond to the behavioural development during the first year of life and are also paralleled with the degree of glucose metabolism within salient functional units. In the newborn, primary sensory and motor cortical structures, cingulate cortex, thalamus, brainstem, cerebellum (vermis) and hippocampi show the highest degree of glucose utilisation. At 2–3 months of age, glucose metabolism increases in the primary visual cortex, parietal and temporal cortex, basal ganglia and cerebellar hemispheres. In the first 4 years, the child’s brain shows more than twice the glucose consumption of the adult brain; this high rate is maintained until about 10 years of age. At the age of 16–18 years, brain glucose metabolism gradually declines and eventually corresponds to adult values (Chugani 1998). Therefore, ‘sensitive’ periods in terms of brain maturation exist not only for perceptual systems but also for cognitive development in general (e.g. Gale et al. 2004), whereby ‘biocultural’ factors influence plasticity within different time scales (e.g. moment to moment and throughout the life span) and multiple neurobiological, cognitive, behavioural and sociocultural levels (Li 2003).
The elevated developmental sensitivity to internal and external changes can result in both positive and negative outcomes. Every disruption of the developmental process, either because of brain dysfunction disturbing self-regulation and autonomy or due to unfavourable environmental conditions, has morphological and/or physiological consequences, because plasticity, as an intrinsic property of the CNS, is always operating as internal and external factors change (Ryan et al. 1997; Nava and Roder 2011). Consequences of dynamic ‘maladaptation’ manifest themselves in delays or disruptions of functional development. It is important to note that ‘young is not always better’ in terms of neuroplasticity, as a factor in overcoming consequences of unfavourable conditions, but that the developing brain may also be more vulnerable than the adult brain in some respects (Giza and Prins 2006). Thus, brain plasticity and vulnerability may rather represent ‘extremes along a continuum’ of morphological and functional change (Anderson et al. 2011). This is an important fact, because it signifies that neuroplasticity continues to operate life-long. Although the window of critical periods for the development of particular brain functions may be almost closed, the larger door of brain plasticity may still remain (sufficiently wide) open and thus offer the opportunity to improve impaired brain functions in older childhood and even in adulthood (e.g. Baltes 1997; Li et al. 2006; Kolb et al. 2011; Kolb and Teskey 2012; Zihl et al. 2014). Thus, despite age-related decline of maximal learning capacity, residual plasticity can enhance functional efficiency by systematic practice beyond the sensitive period (Ostrovsky et al. 2006; Astle et al. 2011). Measures aimed at improving functional outcome levels after acquired brain injury in adults are contingent upon prior life experience (May 2011). However, developmental plasticity, and thus functional outcome of brain dysfunction, is set by the risk associated with the degree and extent of brain injury (severity, dimensions and topography of brain injury), the maturational state of the brain system involved, the integrity of brain structures belonging to the affected intra- and interhemispheric network(s) and associated medical conditions (e.g. epilepsy, medication). In addition, the outcome may be moderated by age at and time since the onset of brain dysfunction as well as the functional resources (‘reserve’) available within the child’s brain and by social environment (family, school and community) (Chugani et al. 1996; Dennis 2000).
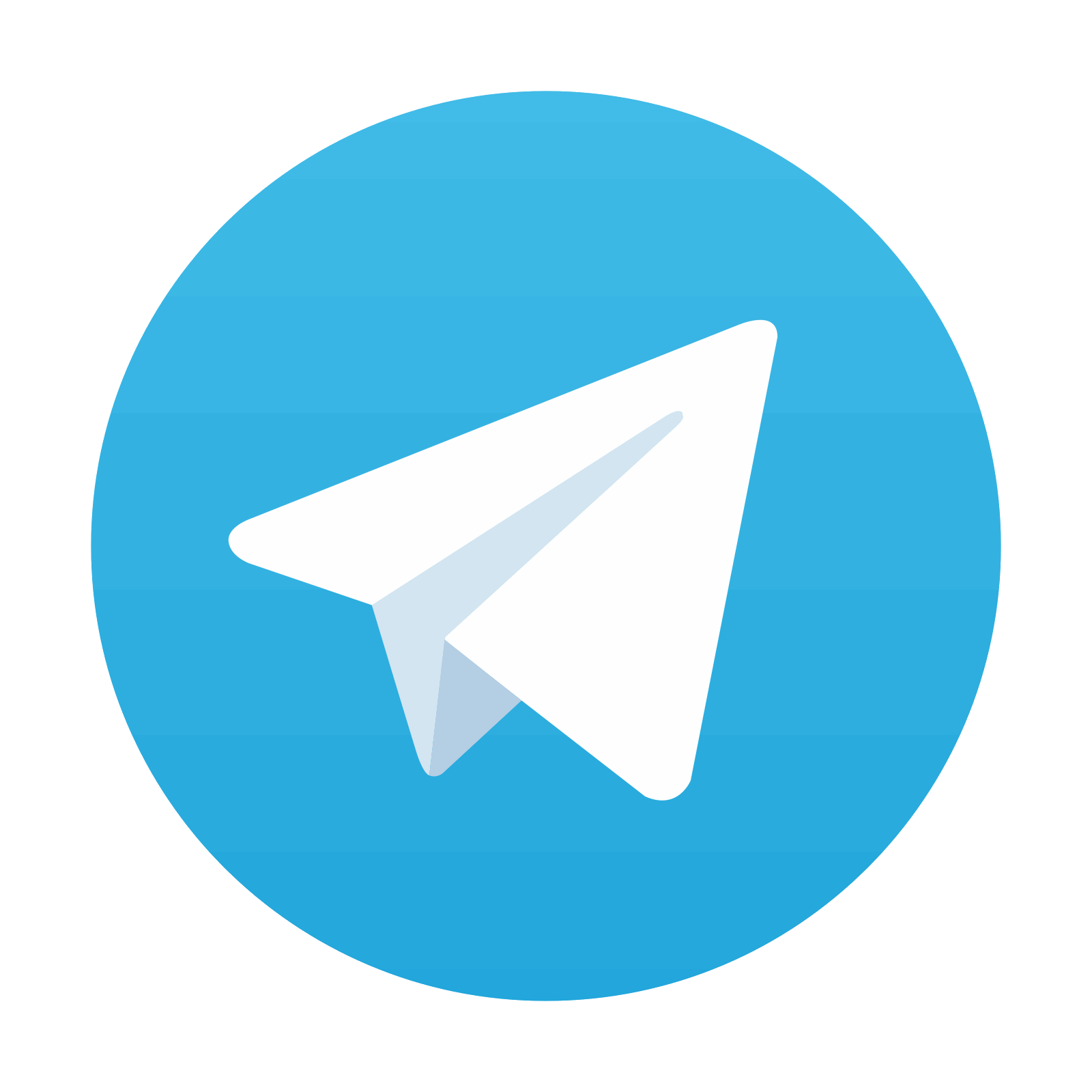
Stay updated, free articles. Join our Telegram channel

Full access? Get Clinical Tree
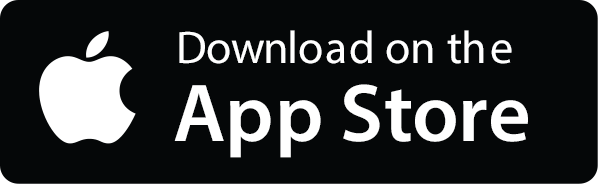
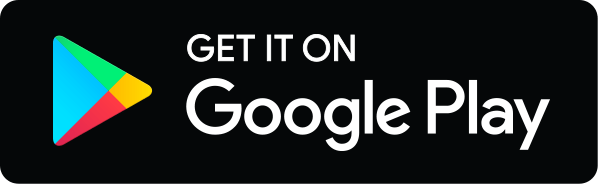