Introduction to EEG Interpretation
Electroencephalography continues to be an essential diagnostic test in clinical practice and its use has evolved over nearly 90 years since its invention. Its role endures despite the numerous breakthroughs in laboratory medicine and the ready availability of high-resolution brain imaging of both structure and function. The reason for this is clear; EEG depicts macroscopic electrophysiologic activity with unique specificity for attributes of brain function and this rendering has exquisite temporal resolution, useful spatial resolution, and can be provided for relatively low cost in almost any clinical situation. For these reasons, EEG is a principal test in the diagnosis of epilepsy, and it has expanded as a tool for monitoring brain function in real time. It clearly has not been replaced by any imaging modality and its future is secure, especially with ongoing progress in recording and data processing technologies. Despite the technical advances, EEG remains a visually interpreted test. Automated analysis is not yet reliable, and accurate interpretation requires both knowledge and experience. Ultimately, experience is a familiarity with the great breadth of EEG activity. The text and figures in this reference are intended to supplement interactive EEG education and to help build both knowledge and familiarity.
Overview of the Basis for EEG
Approaching EEG interpretation for the first time requires some understanding of EEG’s basis, recording technology, descriptive terminology, and general capabilities and limitations. With understanding of these facets, the EEG activity is more clearly interpreted and the diagnostic questions can be more readily addressed.
A detailed discussion of theories for the generation of EEGs is beyond the scope of this reference; however, the following is a basic review of fundamentals. Routine, clinical EEG is the depiction of electrical potentials from the cerebrum; however, it provides a highly limited view of cerebral activity. EEG has an inherent low sensitivity due to the distance between the electrodes and the brain generators and the presence of interposed, resistive tissues. The fields detected by EEG represent only a minority of cerebral current flow because currents generating the potentials flow according to the anatomy of the cerebral surface, and EEG is insensitive to the fields that are tangential to the scalp (Olejniczak, 2006; Schaul, 1998). Moreover, detection of neuronal potentials at the scalp requires sufficient synchronization of neighboring neuronal potentials with a minimal area of 6 to 10 cm2 for at least some forms of activity (Cooper et al., 1965; Tao et al., 2005). This biases EEG to potentials that are longer in duration because such potentials are more likely to overlap in time and thereby synchronize. As such, EEG depicts the excitatory and inhibitory postsynaptic potentials due to their longer durations. The necessary synchronization area also reduces EEG’s spatial resolution to this scale. Nevertheless, EEG has excellent temporal resolution, and can detect changes in the electrical potentials that are in the range of milliseconds.
Recording the low-amplitude cerebral potentials, especially in the context of the much larger amplitude ambient electrical fields, requires elimination of considerable electrical noise. This is accomplished using differential amplification, which is also called common mode rejection. The principle is to use the noise’s widespread and uniform presence to identify it and subtract it from the cerebrally generated activity, termed the signal. In other words, ambient noise is essentially identical at multiple electrodes, but each electrode identifies a different signal from the subjacent brain region. Amplification based on the difference (differential amplification) between two electrode channels produces an output channel that does not include the noise that is in common (common mode rejection). The drawback of this approach is that the output is the difference between the two electrode channels and it does not indicate the brain activity at one electrode location alone.
Identifying the cerebral activity at one location would be possible if one of the electrodes was neutral, that is, it did not detect cerebral activity but still detected the noise; however, placing an electrode far enough away from the brain to make it cerebrally neutral decreases its sensitivity to cerebral electrophysiologic activity and increases its sensitivity to other electrophysiologic activity, so such placement
is not practical when the goal is depicting the low-amplitude cerebral potentials. As such, each EEG output channel includes signal from more than one cerebral region and accurate interpretation must include recognition of this fact.
is not practical when the goal is depicting the low-amplitude cerebral potentials. As such, each EEG output channel includes signal from more than one cerebral region and accurate interpretation must include recognition of this fact.
Differential amplification does not distinguish common noise from common signal, so cerebrally generated activity that is broadly distributed and present at both electrodes comprising a channel is not apparent in the channel’s output. The use of channels comprising distant electrodes benefits the identification of broadly distributed activity; however, such channels are more limited in indicating the location of activity. The output from distantly separated electrodes indicates a difference in the electrical field, and the location of the change in field can be near either electrode or a location that is closer to one electrode than the other but not near either of them. Determining the location of EEG activity that has focal distribution requires output channels comprised of closely placed electrodes, which is the opposite of what is needed for broadly distributed signals. Overall, EEG interpretation is most accurate when it includes both types of output channels and also multiple electrode pairings. The use of such configurations is discussed with montages below.
Orientation to the EEG Record
For standardization reasons, the locations of the electrodes used in recording EEGs are defined by international agreement as the “10–20” electrode system (Fig. 1-1) (Klem et al., 1999). This system uses measurements of the head referenced to reliable anatomic landmarks to minimize the variation in electrode placement across recording technologists and to provide the maximal uniformity in electrode to brain structure correspondence across patients (Fig. 1-2). The nasion and inion define the sagittal midline, and the superior attachments of the ears to the scalp (preauricular points) define the coronal midline. Using increments of 10% and 20% along these principal lines, additional lines are defined, and electrodes are placed at the 10% and 20% increment points of both the principal and additional lines.
A standard nomenclature is used for the electrode locations based on a letter prefix, which indicates the region of the head, and a number suffix, which indicates the exact location within the region. The common letter prefixes are: F for the frontal regions, C for the central regions, P for the parietal regions, T for the temporal regions, O for the occipital regions, and A for the ears. The most commonly used naming system, illustrated in Figure 1-1, has odd number suffices to indicate the left side of the head, even number suffices to indicate the right side, the suffix z to indicate the sagittal midline, and a suffix that includes p to indicate the frontal pole. For example, Fp1 is over the left frontal pole, F4 is over the right frontal lobe, and Cz is at the vertex, that is, the intersection of the sagittal and coronal midlines. In the commonly used 10–20 system, higher numbers generally indicate greater distance from the sagittal midline. As such, F8 is lateral to F4. The midtemporal electrodes are the exception to this because they have the same suffices as their neighboring C electrodes, which are closer to the vertex. A revised international system, called the “10–10” system, corrects this inconsistency with a modified nomenclature and it also includes names for electrode locations between the standard locations of the 10–20 system (Fig. 1-3). This corrected system is not used as commonly in clinical EEG labs.
The EEG record, also called a tracing, truly is a polygraph composed of multiple horizontal output channels, each of which is generated by two electrode inputs. The channels are named for the electrode location names that generate them. A routine EEG includes at least 16 channels, but the advent of digital EEG has allowed flexibility in the number of channels and routine EEGs now commonly have 21 or more channels. The specific electrode location pairs chosen for each channel and the layout of the channels across the EEG page depend on the preferences of the laboratory performing the EEG, but certain EEG page organizations are common and recommended (Flink et al., 2002). The page organizations are termed montages and are divided into two basic approaches that are commonly termed bipolar and referential (Figs. 1-4 and 1-5) (American Electroencephalographic Society, 1994). These terms can be misleading because both organizations use two electrodes, so technically both are bipolar; and both organizations compare one electrode to another, and the second electrode can be termed a reference. However, the common terms help distinguish the two basic organizations from one another.
The channels in a bipolar montage are created from electrodes that are adjacent to one another on the scalp, and this contrasts with a referential montage, which can also be called a common reference montage and uses one or two electrode locations as the reference electrode (second electrode in the pair) for all of the channels. Bipolar montages typically have chains of electrodes that construct a line with each channel including one electrode from the preceding channel. For example, a bipolar montage’s chain can have the following series of channels: Fp2–F8, F8–T4, T4–T6, T6–O2. In this way, the chain includes every electrode comparison across the head from one aspect to the aspect on the opposite side. Referential montages do not have chains or the reversing sequence in the channels’ electrode pairs. Instead, they span regions with maximized symmetry to help clarify the distribution of activity.
The depiction of channel output polarity is based on a convention that specifies a downward deflection for positive and an upward deflection for negative
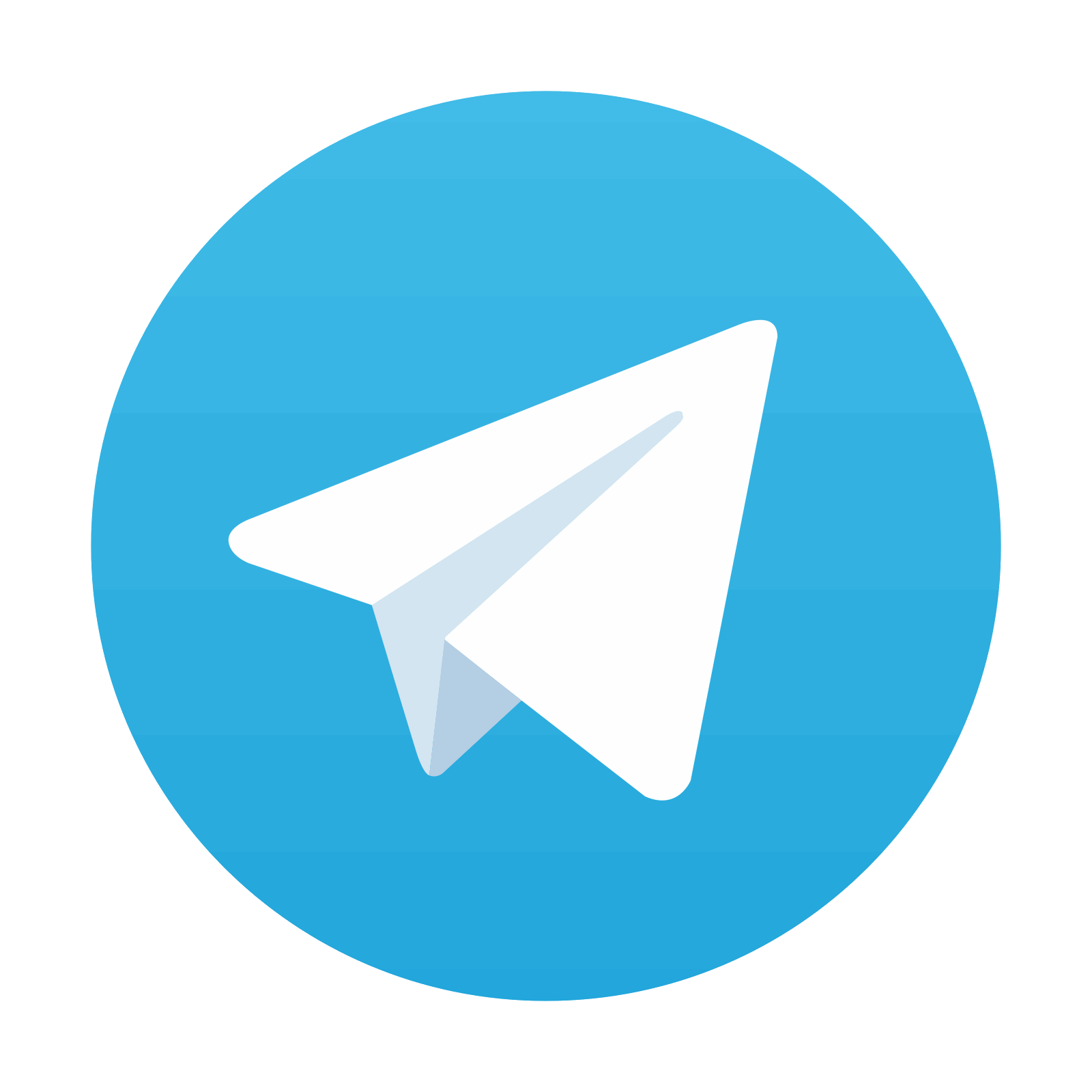
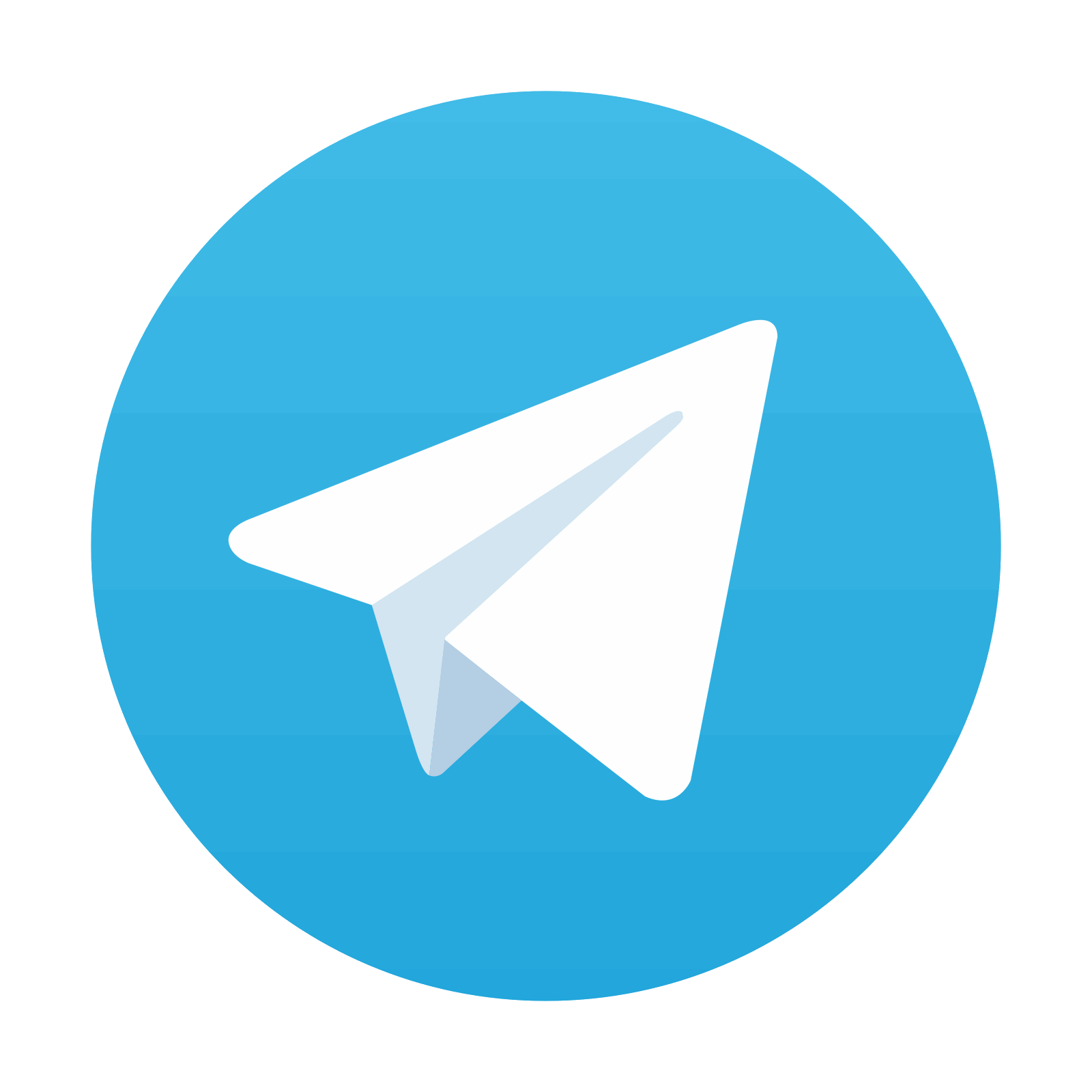
Stay updated, free articles. Join our Telegram channel

Full access? Get Clinical Tree
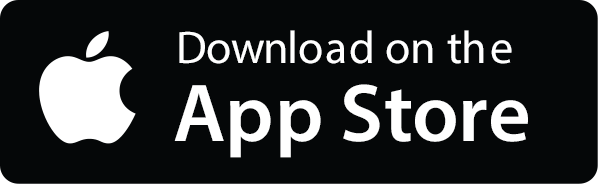
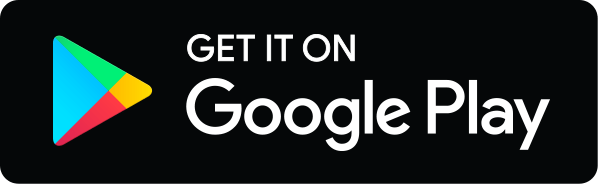