Neurodiagnostic tests aid in determining both localization and diagnosis. The main neurodiagnostic tests are: neuroimaging, cerebrospinal fluid (CSF) analysis, electroencephalography (EEG), and electromyography/nerve conduction studies (EMG/NCS). EEG is discussed in the context of the diagnosis of seizures and epilepsy (Ch. 18) and EMG/NCS in the context of the diagnosis of neuromuscular disease (Ch. 15 for the principles; Chs. 16–17 and 27, 28, 29, 30 for clinical use). Neuroimaging and CSF analysis are discussed throughout this book, but an introduction to their use and interpretation is provided here.
Neuroimaging allows for visualization of the structures of the neuraxis, and is used as an extension of the neurologic examination. If a patient’s symptoms and signs localize to a particular part of the neuraxis, neuroimaging of that region can provide additional information about the underlying pathologic process. The disease-based chapters of Part 2 of this book discuss when neuroimaging should be obtained with regard to particular symptoms and diseases, and how it can aid in diagnosis of various neurologic conditions.
In clinical practice, many patients have undergone neuroimaging studies before a neurologist has evaluated them, and some patients may be referred for neurologic evaluation because of neuroimaging findings rather than clinical findings. In such scenarios, part of the clinical reasoning process is interpreting the neuroimaging in the context of the clinical findings: Do the neuroimaging findings correlate with the patient’s clinical presentation? Are there aspects of the patient’s clinical presentation that do not have a neuroimaging correlate? Are there incidental findings that have no relationship to the patient’s current presentation but require further evaluation or longitudinal clinical and radiologic surveillance? Whether neuroimaging is obtained and reviewed before evaluation of a patient or in the process of a patient’s evaluation, neuroimaging must always be interpreted in the context of the patient’s history and physical examination in order to prevent misinterpretation/overinterpretation/underinterpretation of radiologic findings.
The cornerstones of neuroimaging of the nervous system are computed tomography (CT) and magnetic resonance imaging (MRI), both of which can be performed with or without intravenous contrast, and both of which can be used to evaluate the cerebral vessels (CT angiography [CTA] and venography [CTV]; MR angiography [MRA] and venography [MRV]). More specialized radiographic techniques used in the evaluation of neurologic diseases include CT and MR perfusion studies, MR spectroscopy, nuclear imaging (positive emission tomography [PET] and single photon emission computed tomography [SPECT]), and transcranial Doppler ultrasound.
What follows is a primer on neuroimaging. It is not intended to be of sufficient scope and detail for radiologists and radiology trainees, but is meant to provide the clinician with an approach to interpreting neuroimaging studies of the brain and spinal cord (bone and extracranial soft tissues are not discussed).
Whether using CT or MRI, and whether evaluating the brain or spine, the two main goals are as follows:
Identification of normal structures and any disturbance or distortion of these structures: Is everything there that is supposed to be there, and does it look as it is supposed to look?
Are tissue structures of normal size or is there atrophy or expansion of one or more regions of the brain or spinal cord?
Are the fluid compartments of normal size and configuration (i.e., ventricles, cisterns, sulci of brain; central canal of spinal cord)?
Are structures where they are supposed to be or is there herniation (displacement of structures beyond their normal compartments)?
Identification and characterization of abnormal lesions: Is there anything there that should not be there, and if so, how can it be characterized?
CT: Are there regions of hypodensity (darker than normal tissue density) or hyperdensity (brighter than normal tissue density)?
MRI: Are there regions of hypointensity (darker than normal tissue intensity) or hyperintensity (brighter than normal tissue intensity)?
When interpreting neuroimaging studies, it is helpful to begin by scrolling once from top to bottom and then from bottom to top through all obtained sequences, first taking inventory of normal structures and abnormal findings, and then characterizing them in radiographic terms before interpreting them (e.g., “There is a hypodensity spanning the left frontal and temporal lobes with sharply demarcated borders and no other apparent abnormalities.”). This will allow for an initial radiologic differential diagnosis that can be aligned with the clinical presentation and will avoid potentially premature conclusions (e.g., focusing on an obvious abnormality at the expense of not noting other more subtle abnormalities or global abnormalities).
The easiest neuroimaging abnormalities to see are asymmetries (e.g., a lesion on one side that contrasts with the normal contralateral side). It can be more challenging to note symmetric abnormalities (e.g., diffuse cerebral edema, symmetric ventriculomegaly, symmetric atrophy), especially when first learning to interpret neuroimaging. This skill emerges over time after reviewing a large number of normal and abnormal studies (just as appreciating what the range of normal is for a physical examination finding such as reflexes requires examining a large number of patients).
The main neuroimaging modalities used to examine the brain and spine are CT and MRI. Noncontrast CT has the advantages of being able to be completed rapidly and being better at analyzing abnormalities of bone. It may be more sensitive than MRI for detecting acute intracranial hemorrhage, but is less sensitive for identifying subtle pathologic changes and is particularly insensitive in the posterior fossa (due to beam hardening artifact). MRI has several advantages: greater resolution than CT, increased ability to detect small and/or subtle abnormalities that may not be visible on CT, ability to use different MRI sequences (discussed below) to allow for abnormalities to be viewed in different ways (which aids in characterizing them), and no radiation exposure. Compared to CT, MRI takes longer to perform, is more expensive, and cannot be performed in patients with cardiac pacemakers, other implanted ferromagnetic medical devices, or exposure to shrapnel.
CT can be viewed using different windows that highlight different aspects of the image (e.g., brain vs bone) (Fig. 2–1). CT relies on differences in density of tissues to generate an image. Denser tissues (and some pathologic findings) are brighter (hyperdense), whereas less dense tissues (and some pathologic findings) are darker (hypodense). At the extremes, bone is the most dense structure on a head CT and appears the brightest, while air (e.g., in the sinuses) is the least dense and is therefore the darkest. CSF is denser than air but not as dense as brain. Brain is denser than CSF. Within the brain, the gray matter is denser than the white matter, so the cortex and deep gray matter structures (basal ganglia and thalamus) are slightly brighter than white matter. The most common CT window used by neurologists is the brain window, which allows for these various structures to be identified and differentiated, but is less sensitive for noting bony abnormalities (e.g., fractures), which are best visualized using the bone window.
Abnormal brain CT findings (e.g., beyond changes in the shape or size of normal structures such as atrophy, ventricular enlargement) can be classified as hyperdensities or hypodensities.
The most common causes of hyperdensity in the brain on CT are hemorrhage and calcification (Fig. 2–2). Contrast enhancement is also hyperdense (see “Contrast-Enhanced Neuroimaging” below). The distinction between hemorrhage and calcification as a cause of a CT hyperdensity can generally be made in the context of the clinical history (e.g., acute-onset focal deficits with a corresponding hyperdense lesion suggests hemorrhage), but if there is doubt, Hounsfield units can be determined (60–100 for blood, 100–200 for calcification; for reference, bone is typically greater than 1000). Calcifications may represent normal findings (e.g., in the choroid plexus, in the falx cerebri, in the pineal gland, and in some older individuals in the basal ganglia). Pathologic hyperdensities on brain CT can be caused by:
Acute intracranial hemorrhage (intraparenchymal, intraventricular, subarachnoid, subdural, epidural; see Ch. 19)
Calcification (tumors or infectious lesions may have calcified components; e.g., neurocysticercosis)
Hypercellular tumor (e.g., lymphoma)
Thrombosed blood vessel (e.g., hyperdense vessel sign in acute ischemic stroke and cord sign in venous sinus thrombosis [Ch. 19])
Atherosclerotic plaques within arteries
Contrast-enhancing lesions such as tumor, abscess, acute demyelination, subacute stroke (see “Contrast-Enhanced Neuroimaging”)
Pathologic hypodensities on brain CT can be caused by (Fig. 2–3):
Any type of pathology that causes vasogenic edema: tumor, inflammation, infection, trauma, ischemic stroke (although CT may be normal in the acute phase of ischemic stroke; see Ch. 19)
Sequelae of prior injury (trauma, infarct, demyelinating lesion)
FIGURE 2–3
Hypodensities on head CT. A: Left temporo-occipital hypodensity caused by ischemic infarct in the middle cerebral artery territory causing hypodensity that extends to the inner table of the skull. B: Left frontal hypodensity due to toxoplasmosis. Note that the lesion respects the gray–white junction, sparing the overlying cortex (see text for explanation).


The differential diagnosis for a discrete region of hypodensity on brain CT includes stroke, tumor, inflammatory lesion, and infectious lesion. Since strokes respect a vascular territory, the hypodensities that they cause generally have clear, distinct borders. In contrast, edema around a tumor, infectious lesion, or an acute demyelinating lesion typically has less distinct boundaries. Additionally, if the hypodensity in question is near the cortical surface, an infarct will most often include the cortex since a cortical vessel will supply both the cortex and the underlying white matter. In contrast, most neoplastic, infectious, and demyelinating lesions involve the subcortical white matter and, therefore, the hypodensity caused by such lesions will usually respect the gray–white boundary, sparing the overlying cortex (Fig. 2–3).
The main clinically important MRI sequences for evaluating the brain are T1, T2, FLAIR, DWI and ADC, and SWI (or GRE) (Fig. 2–4). These sequences differ in the way they are acquired in order to allow for visualization of different types of pathology.
FIGURE 2–4
MRI sequences (normal MRI of the brain). A: T1-weighted. B: T2-weighted. C: FLAIR (fluid-attenuated inversion recovery). D: DWI (diffusion-weighted imaging) E: ADC (apparent diffusion coefficient) F: GRE (gradient echo).




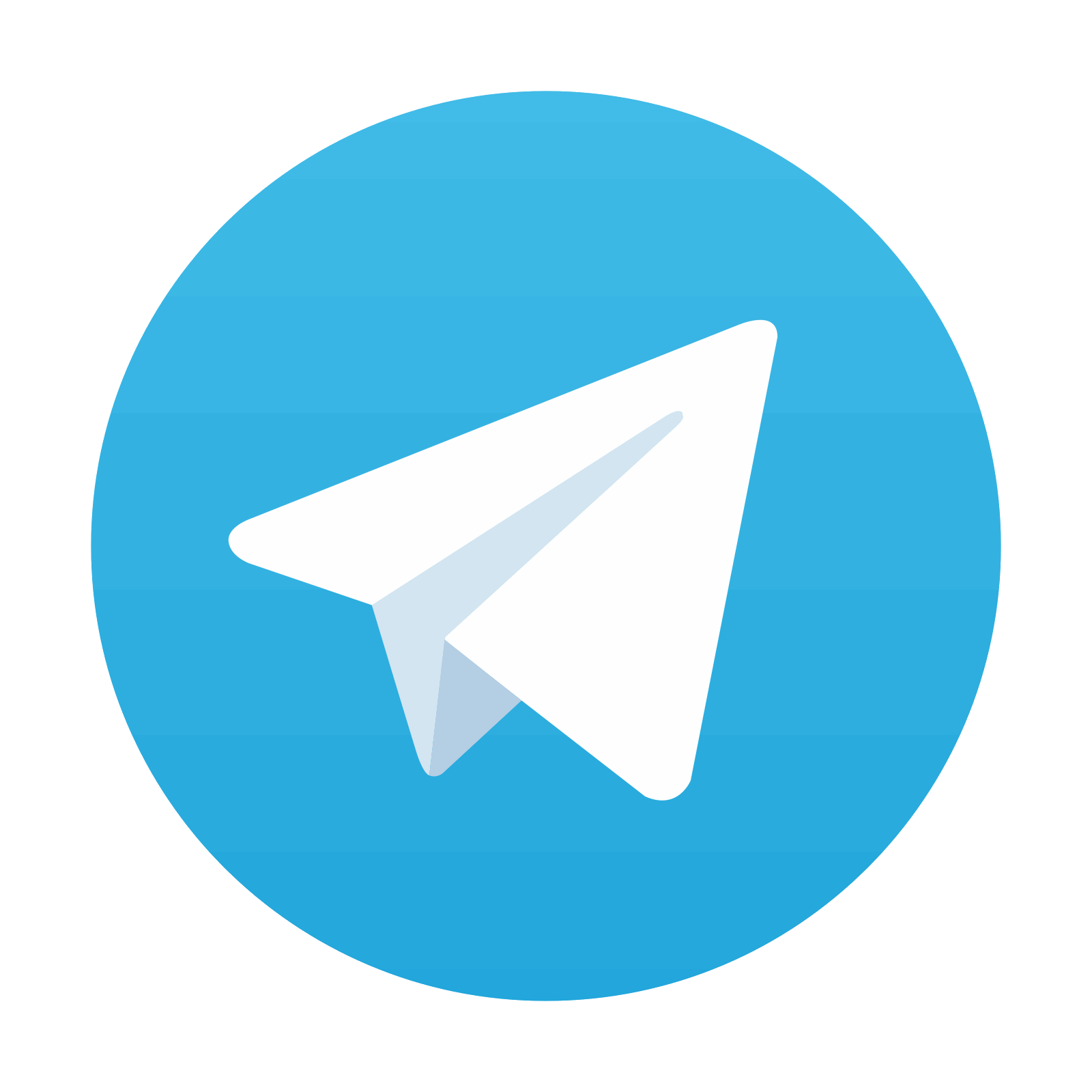
Stay updated, free articles. Join our Telegram channel

Full access? Get Clinical Tree
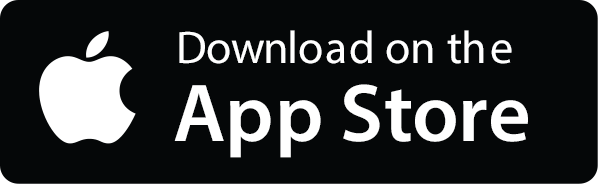
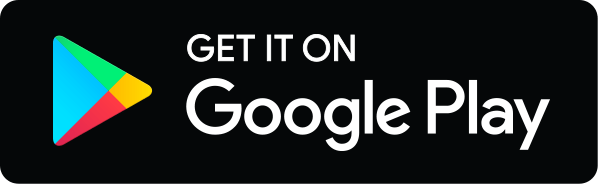
