At rest, the resting membrane potential, or the absolute difference in electrical potential between the inside and the outside of the inactive neuron, results predominantly from the membrane permeability to potassium as a result of the open state of the potassium leak channels. This resting membrane potential is approximately −70 mV. If an electrical circuit diagram is used to demonstrate the transmembrane potential at rest, with conductance and resistance of Na+, K+, and Cl− shown in parallel, the contribution of conductance of K+ and Cl− are responsible for the overall current flow and membrane potential.
When a negatively charged stimulus (physiologic or external) is applied to the extracellular axon membrane, there is a decrease in the value of the resting membrane potential as the charge difference between the extracellular and intracellular membranes decreases (called depolarization). If the membrane is depolarized only a small degree, only a few sodium channels are activated, and a local potential is generated. If this charge difference reaches the excitation threshold for opening of many voltage-gated sodium channels (approximately −50 to −55 mV) the conductance of sodium rapidly becomes greater than that of K+, and Na+ ions rapidly move from the extracellular to intracellular space, resulting in a movement of the transmembrane potential difference toward the equilibrium potential of sodium (+60 mV). This depolarization locally reverses the polarity of the membrane, the inside becoming positive with respect to the outside.
This rapid change in conductance results in the action potential. Action potentials are “all-or-none,” allowing for rapid transmission of information over long distances along the nerve. The change in sodium conductance is transient and lasts only a few milliseconds. As the sodium channels become inactive and the potassium channels re-open, the sodium conductance decreases and potassium conductance increases, resulting in an increase in flow of potassium out of the cell and repolarization of the membrane.
The rate of return of the membrane potential to the baseline slows after sodium conductance has returned to baseline, producing a small residual on the negative component of the action potential, which is called the negative afterpotential. This afterpotential is positive when the membrane potential is recorded with a microelectrode within the cell, but it is negative when recorded with an extracellular electrode. The increase in potassium conductance persists and results in a hyperpolarization after the spike component of the action potential—the after-hyperpolarization—which is due to continued efflux of potassium ions, with a greater than resting difference in potential between the inside and the outside of the cell. The after-hyperpolarization is positive when measured with extracellular electrodes and therefore is called a positive afterpotential.
The changes in Na+ and K+ channel activation and inactivation overlap to a degree. As a result, the membrane potential is a function of the ratios of the conductances of the Na+, K+, and Cl− ions. These can be demonstrated in an electrical circuit diagram, demonstrating current flow relative to the conductances (ion channel permeability) of Na+, K+, and Cl− in the resting states and after a threshold-reaching stimulus.
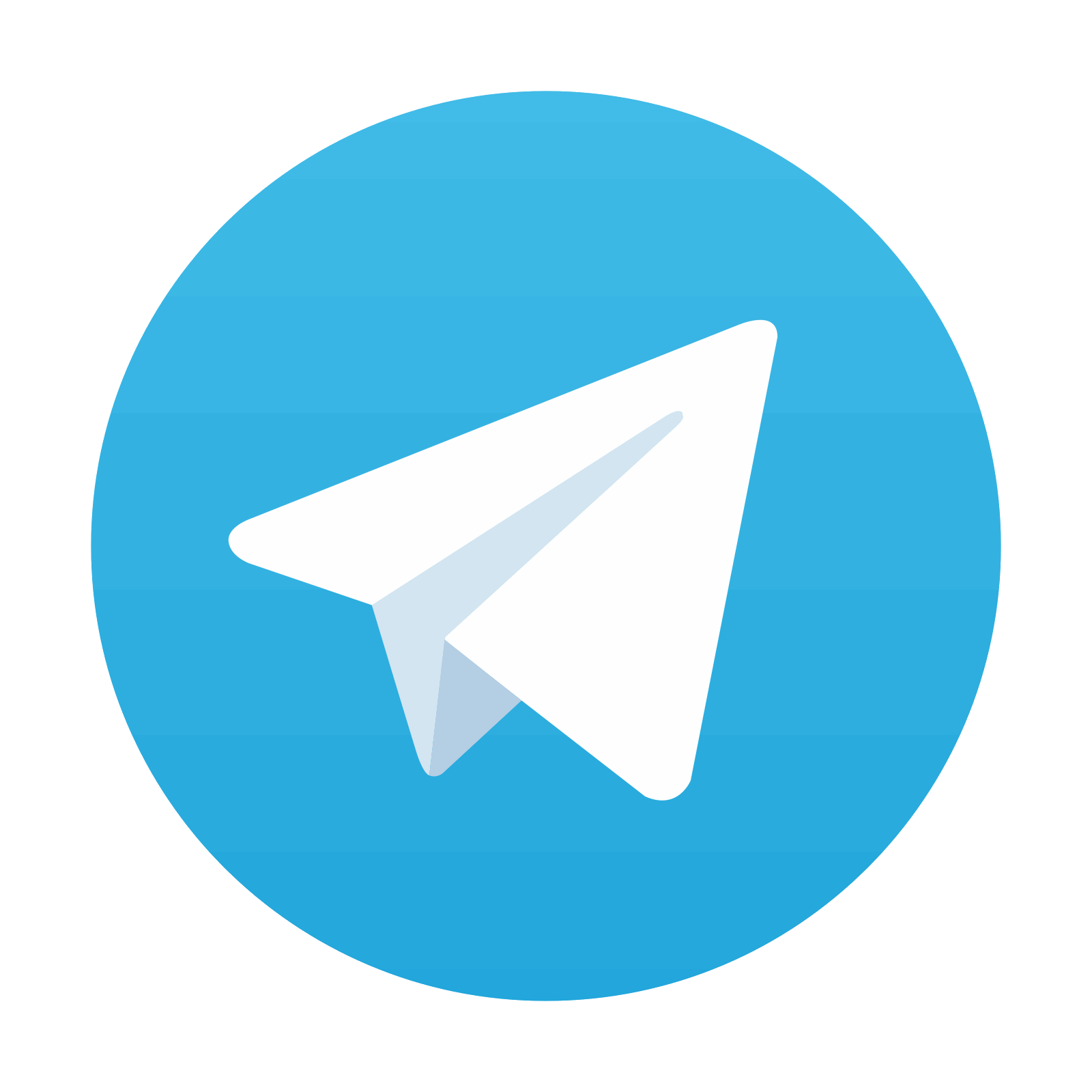
Stay updated, free articles. Join our Telegram channel

Full access? Get Clinical Tree
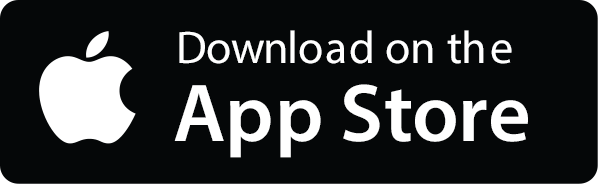
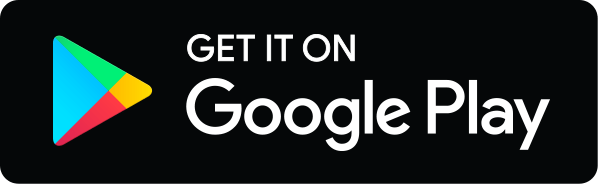