21 Proponents of the use of single-cell microelectrode recording (MER) during movement disorder surgery consider that those who use only macrostimulation are not performing physiological exploration and refer to them as “anatomists.”1 It is time to remind the reader that Tasker et al wrote an entire book in 1982 about the use of macrostimulation in mapping the thalamus and midbrain in humans. In The Thalamus and Midbrain of Man: A Physiological Atlas Using Electrical Stimulation,2 these authors delivered a detailed atlas about the gross somatotopy of the subdivisions of the thalamus and midbrain based solely on intraoperative macrostimulation. Furthermore, in a chapter published in Neurosurgery Clinics of North America in 1990, Tasker, compared macro- and microelectrode techniques and stated the following: Macrostimulation is easy, requires minimal instrumentation, is quick, [and] identifies a wide range of brain structures, even at variable distances from [the] probe…. Microelectrode techniques are more difficult, time consuming, [and] require more sophisticated equipment…. Microelectrode can identify only a limited repertoire of structures. The tip must be very close to a structure before it can be recognized at all…. The more limited current spread means that unless an excitable structure is very close, it will be missed; the surgeon may gain no clue from a “negative” trajectory where to seek next.3 With this in mind, it should be acknowledged once and for all by the supporters of MER that macrostimulation is neurosphysiology and that the term anatomists should be reserved for those who do not use any electrode whatsoever (i.e., for those who use a Gamma Knife) in movement disorder. Surgery. What is intraoperative neurophysiology during movement disorder surgery? It is simply a procedure to verify that the imaged anatomical target aimed at is consistent with the physiological target. Neurophysiological exploration aims at corroborating the anatomy with the physiology to treat a (dys)functional symptom. Given the well-known interindividual anatomical variability of subnuclei and pathways in the midbrain, it is mandatory to explore their function in the individual patient to ascertain a good position of the probe in the target prior to lesioning or DBS implant. In that sense, intraoperative physiological corroboration can be performed with a variety of methods used alone or in various combinations. These methods include impedance monitoring, evoked potentials, macroelectrode stimulation, microelectrode stimulation, single-cell microelectrode recording, semi-microelectrode recording, analysis of neural noise, local anesthetic blockade, mechanical introduction effect, and even so-called reversible lesion. These different methods have their advantages and disadvantages, depending on what information one needs or requires and for what purpose. For example, it is evident that if one is doing a research study to find out about the variability of firing rate of the GPi in a Parkinson’s patient before and after intraoperative injection of apomorphine,4 then one must use single-cell MER to answer that particular question. But if one is doing a routine pallidotomy or subthalamic DBS, then macrostimulation would be enough to place the lesion or the DBS electrode adequately. This chapter will not deal with all available intraoperative physiological methods nor with the use of these methods for research purposes. Instead, it will try to answer the questions of whether MER is mandatory to conduct a proper and safe stereotactic functional procedure and whether MER yields better results and fewer complications than a procedure done with macroelectrode guidance. Because no randomized studies exist to answer these issues, the next best approach is to conduct a careful study and review of the published experience in MER and macro-stimulation-guided movement disorder surgery. The proponents of the use of MER in movement disorder surgery put forward the following arguments in favor of using this technique instead of macrostimulation-guided surgery: Furthermore, it has been claimed by some MER proponents that teams using MER are more reliable in reporting results than those not using MER because they use movement disorder specialists and standardized assessment scales.1 The points listed above will be examined in light of the recent literature on movement disorder surgery that has been published by some active MER groups. Additionally the author’s personal opinion about the role and contributions of MER versus macroelectrode-guided surgey will be given. Some authors have claimed that MER allowed them to “sculpt” the lesion to fit into the sensorimotor part of the globus pallidus internalis.5,6 Vitek et al5 wrote that microelectrodes contributed to establish a map, allowing the lesions to lie “within the sensorimotor portion of the GPi while sparing nearby structures for example GPe, nucleus basalis, optic tract and internal capsule.” However, other MER supporters stated that it was unrealistic to think that lesions could be restricted consistently to the GPi.7 De Long et al8 reported on an autopsy performed 7 months after successful pallidotomy showing that the lesion encompassed a large portion of the caudolateral GPi, with some encroachment on the GPe anterodorsally. In a comment on the article by Tsao et al7 published in Neurosurgery in September 1998, Bakay1 stated that his group had reviewed patients operated on elsewhere and discovered that the pallidotomy lesions lay in the amygdala in two and in the internal capsule in one, whereas in the other patients the lesions lay too anterior or too lateral. He wrote: “A list of most of the neurosurgeons involved …. reads like a Who’s Who of stereotactic neurosurgery.” Because lesion location can indeed be determined objectively by carefully studying MR scans,9 I examined the published MRIs in the literature and found the following. In an excellent pioneering article on microelectrode-guided ventral posterior pallidotomy published in Neurology in April 1995,10 there was one coronal MRI figure (Fig. 2, p. 755) showing a microelectrode-guided pallidotomy lesion claimed to lie within 1 mm of the target. This lesion lay obviously very dorsal and anterior because the optic chiasm that lies ∼10 mm anterior to the target was indeed visible on that coronal MR picture. The same authors published another MRI depicting a medial microelectrode-guided pallidotomy illustrating each of two articles that appeared in Advances in Neurology (Fig. 1, p. 586) in 1996,11 and in Stereotactic and Functional Neurosurgery (Fig. 2, p. 166) in 1997.12 This figure showed a ventral and posterior but extremely lateral pallidal lesion. In the November 1997 issue of Movement Disorders.13 an MRI was published depicting microelectrode-guided pallidotomies done bilaterally in a dystonia patient (Fig. 2, p. 868). The right-sided pallidotomy lesion lay in the internal capsule, just medial from the visible medial border of the pallidum internum. The contralateral lesion was clearly more lateral and well located at the intended GPi target. The group of Baylor College of Medicine published an MRI of bilateral microelectrode-guided GPi pallidotomies14: the right-sided lesion appeared to lie in the GPe (Fig. 1, p. 695). Another microelectrode-guided pallidotomy lesion illustrated an article published in Brain in 199815: Figure 1 B, on page 64 of that that article showed a posteroventral pallidotomy lesion located so medial in the GPi that roughly half the lesion in fact lay in the internal capsule. Gross et al16 analyzed variabilities in locations of pallidotomy lesions and showed that, even though microelectrode-guided, the lesions were scattered in the GPi from anteromedial to posterolateral (Fig. 1, p. 409). Later on, the same group of Toronto published several studies showing that those patients who suffered cognitive side effects after MER-guided pallidotomy had their lesions mostly in the anterior, limbic or associative part of the GPi.17,18 One reason for these misplaced lesions, in my opinion, is that the MER actually contributed to the surgeon’s misplacement of a lesion where sensorimotor cells were found, no matter the claims of that group that “microelectrode recordings define the ventral posteromedial pallidotomy target.”19 Because there are no fireproof walls dividing the pallidum in sensorimotor, associative, and limbic parts, it might well be that few sensorimotor cells were actually registered by MER in the anterior associativeᑛ limbic pallidum. This would by no means constitute evidence that it was the sensorimotor, posteroventral pallidal area that was targeted. In 2000, the Kansas group published in Neurosurger an article entitled “Pallidotomy Microelectrode Targeting: Neurophysiology-Based Target Refinement.”20 The authors aimed at a starting target point located 3 mm anterior to the midcommissural point, 20 to 22 mm lateral to the intercommissural line, and 4 to 10 mm ventral to the AC-PC plane. This meant that they aimed at the posterior and ventral areas of the globus pallidus. They wrote in the introduction that “postoperative magnetic resonance imaging … data were used to demonstrate the final lesion location.” In the Result section, they stated that postoperative MRI showed that the “location of the lesion within the globus pallidum was confirmed.” Upon studying the only MRI in this article (Fig. 2), showing an axial cut with a 1-day-old pallidotomy lesion, it was plainly evident that the lesion lay at the level of the foramina of Monro: This lesion lay very dorsal and very anterior compared with the target point previously defined by the authors. This lesion location definitely did not correspond to the authors’ own anatomic coordinates listed above nor to the sensorimotor posteroventral pallidum aimed at. The visualized lesion lay in the anteromediodorsal pallidum, a target favored by Cooper21 in the 1950s when he performed chemopallidectomies. To date I fail to understand how the authors’ meticulously conducted microelectrode studies could refine the lesion location in this particular patient, the MRI of whom was precisely chosen to illustrate how MER neurophysiology could contribute to “target refinement.” In pallidal surgery, the physiological-topographic information provided by microelectrode exploration of the pallidum and its implication for lesion location have been addressed by several researchers. The results of these studies have shown some variabilities in the interpretation of MER results. Sterio et al22 found no correlation between discharge patterns of GPi or GPe cells and tremor activity, and Lozano et al23 found that tremor-synchronous cells were recorded four times more often in the internal part of the GPi (GPii) than in its external part (GPie). Taha et al24 found that arm cells were clustered rostrally and caudally in the GPi, and leg cells were clustered centrally. Bakay1 reported that laterally placed lesions in the GPi produced better arm effect and medially placed lesions produced better leg effect. Guridi et al25 reported that 50% of leg units are in the most dorsal third of the GPi and 66% of the upper limb unit are in the most ventral two thirds of the GPi. Gross et al16 reported that centrally located pallidal lesions were best for akinesia, posterolateral lesions best for tremor, and anteromedial lesions best for dyskinesias, and Uitti et al26 stated that akinesia improved most with posterolateral lesions. Krauss et al,27 on the other hand, could not find any correlation between lesion location and results of their microelectrode-guided pallidotomies. All of these researchers are proponents of MER in movement disorder surgery, yet there is a remarkable disparity between their interpretations of their findings and the implications of MER on lesion placement and symptomatic effect. Furthermore, one might ask why there is such a disparity in the location of their lesions, given that all those lesions were MER-guided and supposed to lie within the MER-defined sensorimotor posteroventral pallidum. An article reporting on a multicenter thalamic DBS study, published in 1997 in Annals of Neurology,28 was illustrated with one figure: Figure 1, page 293, showed a sagittal MR scan with a DBS electrode, the four contacts of which were reportedly lying in the ventral intermediate (Vim) nucleus of the thalamus. Aside from the virtual impossibility of having the 10.5 mm length of all four contacts of the DBS electrode lie within the Vim, a close look at this figure revealed that the tip of the DBS electrode was just at the border of a CSF space; however, there is no CSF space in the vicinity of the Vim. The closest CSF space posterior to the Vim is the ambient cistern, which lies behind the pulvinar, which is ∼10 mm posterior to the Vim. This electrode thus lay in the posterior pulvinar, rather far away from the Vim. Germano29 published a chapter on surgical technique in DBS in which she explained the anatomical relations of the Vim, the pallidum, and the subthalamic nucleus to adjacent structures and insisted on the rationale for using microelectrode techniques. Figure 5C, page 165, in that chapter showed an axial MR scan depicting, according to the figure legend, the most distal contact of the electrode in the Vim. On that scan, one can see that the tip of the electrode is in fact too anterior and too dorsal in relation to the ventricular landmarks and in relation to the expected location of the Vim target. Damier et al30 reported in July 1999 on 17 patients with MER-guided subthalamic DBS and stated that 2 of the patients (12%) had their electrodes several millimeters outside the subthalamic nucleus. Ten months later, Pidoux et al31 presented 23 subthalamic DBS patients and stated that all DBS electrodes were in the subthalamic nucleus. An article on the usefulness of MER in subthalamic DBS surgery was published in 1999.32 The single figure illustrating that article showed the bilateral DBS lead supposedly in the subthalamic nucleus, whereas it appeared in fact to lie extremely assymetrical in both depth and A-P directions. It is evident that there is also mistargeting in MER-guided DBS surgery; however, there are fewer illustrations that can show this mistargeting because some researchers avoid performing MRI on patients with implanted leads, and if performed, the quality of the images is so poor that no meaningful evaluation can be done as to the exact anatomic location of the leads. Therefore, there is no possible evidence that can substantiate claims that MER is mandatory to place the subthalamic DBS electrode in the center of the sensorimotor region, as stated by some.33 The sensorimotor area of the subthalamic nucleus barely constitutes 50% of that area. A review of the literature disclosed that teams using MER for pallidotomy often reported that their method enabled them to make lesions in the sensorimotor posteroventral pallidum without encroaching on adjacent structures.5,10 I found, however, that there were wide variations in lesion size among groups using microelectrode technique, and even within one and the same group. Cohn et al6 from Emory University reported the following concerning their lesions: average diameters 5 mm in transverse, 6 mm in A-P, 7 mm in craniocaudal directions, with an average lesion volume of 118 mm3 (range: 52–264 mm3). They explained these variations thus: “Large range in lesion size reflects adjustments in pallidotomy technique: several of the patients operated early in our series had fewer passes and smaller lesions than those operated more recently”6 Vitek et al5 also from Emory University, reported in another article published the same month that “the lesion was ~3 mm in diameter.” They stated: “In our first 10 cases, we made an average of 6 microelectrode passes per patient…. In our last series, we made only 3 passes per patient.”5 Notwithstanding these conflictual statements about the number of microelectrode passes in the history of pallidotomy at Emory, if we assume a lesion volume of 118 mm3 on average,6 it may be difficult to conceive how such a lesion can remain confined within the sensorimotor portion of the posteroventral internal globus pallidus, without encroaching on either the external pallidum or the internal capsule, or both. Dogali et al10 of New York University reported in 1995 that their MER-guided pallidal lesions ranged between 60 and 90 mm3, with a mean of 75 mm3. Alterman et al,34 also of New York University, reported 4 years later, in February 1999, a mean pallidotomy volume of 262 ± 76 mm3. The mean volume of Alterman’s microelectrode-guided pallidotomies is virtually the same as the mean volume of Gamma Knife pallidotomies reported by Young et al in 1998.35 Alterman et al examined to what extent MER improved their targeting of the posteroventral pallidum in 132 pallidotomies performed between July 1992 and November 1996, and they referred to several articles published previously from their center, where details on technique and results of their pallidotomy can be obtained. The authors insisted that the number of microelectrode passes had decreased from a mean of 3.6 (range 1–15) in the years 1992–1993 to a mean of 1.8 passes (range 1–4) in 1996, which contributed to a radical decrease in surgical time. The authors attributed these improvements mainly to experience and not to better imaging because the decrease in surgical time predated their use of fast spin echo/inversion recovery MRI by more than 2 years. Their 132 MER-guided pallidotomies had a mean lesion volume of 262 ± 76 mm3, which would translate to a mean lesion diameter of 7.9 ± 5.2 mm. According to the authors, by using MER, they could correct the MRI-defined target by a three-dimensional distance of 1 to 4 mm in 86% of the patients. However, the large volume of lesions reported here must necessarily encompass several of the adjacent millimeters of pallidal tissue that the authors, on the basis of MER, had deemed inappropriate as targets for coagulation, prompting coordinate corrections prior to lesioning.34 The previous study from that same center was the one published by Dogali et al10 in 1995. The mean volume of their lesions was 75 mm3, with a range between 60 and 90 mm3. This translates to an average lesion diameter of 5.2 mm, with a range between 4.8 and 5.5 mm. Dogali et al stated that all their lesions lay within 1 mm of the target, which was contradicted by the illustration of that same article, as explained above. Thus, in the subsequent article by Alterman et al, the mean lesion volume was 3.5 times larger than that previously published by Dogali et al. Was this due to the decrease in number of microelectrode passes? Samuel et al’s15 lesions were 145 ± 49 mm3, and the lesions of Krauss et al27 were 44.4 ± 17.6 mm3, with a range of 14 to 92 mm3. Obeso et al36 illustrated a chapter on ablative surgery with a figure of a pallidotomy lesion: Figure 46.3, page 1055, shows a very large pallidotomy lesion encompassing a great part of the globus pallidus. All of these authors used microelectrode techniques to guide the placement and extent of their lesions to fit within the sensorimotor posteroventral GPi. Laitinen et al,37 using macrostimulation-guided pallidotomy, obtained posteroventral pallidal lesions lesions ranging between 21 and 160 mm3, with a mean of 95 mm3. Johansson et al38 obtained with the same technique lesions of 73 mm3 in mean, with a 95% confidence interval of 47 to 102 mm3. Hariz and Hirabayashi,39 using the same surgical technique, reported lesions of 87 mm3 in mean with a range of 9.6 to 255 mm3. DBS in the Vim is the oldest and probably best documented among the DBS procedures, probably because Vim stimulation is antecedent to pallidal or subthalamic stimulation, and, in the United States, only Vim thalamic stimulation so far has been approved by the Food and Drug Administration. Studying the electrical settings of DBS that are needed to control tremor may give an indirect indication as to the accuracy of electrode placement and its proximity to the Vim target. Publications on microelectrode-guided Vim DBS reported in general higher parameters of stimulation than non-microelectrode-guided DBS, both in Parkinson’s disease and in essential tremor patients. The American multicenter study on Vim stimulation28 reported a mean stimulation amplitude of 3 V at 3 months in both ET and PD patients. At 12 months, the parameters were 3.03 V, 86.5 msec in ET and 3.38 V, 117.5 msec in PD patients. In another microelectrode-guided Vim DBS study from Baylor College of Medicine, the stimulation parameters at 3 months were 2.7 V and 256 msec in ET patients and 2.9 V and 231 msec in PD patients.40 In a case study from Vanderbilt University41 one PD patient had a Vim DBS electrode implanted using six microelectrode passes for target determination; the electrical parameters of stimulation in this patient were at 1 week postsurgery 3.3 V and 150 msec. Such a high voltage and pulse width at only 1 week after Vim surgery cannot but indicate that the electrode is not in the proper target, no matter the six MER passes. For comparison, the results of the European Vim stimulation multicenter study (in which 13 centers were involved, 11 of them not using microelectrode technique), showed the following42: at 3 months 2.17 V, 92.73 msec in ET patients and 2.34 V, 88.92 msec in PD patients; at 12 months 2.4 V, 83.86 msec in ET and 2.51 V, 81.92 msec in PD patients. In an article published in 1999 about a patient with dystonia treated by MER-guided pallidal stimulation, Kumar et al43 stated that the patient had the following electrical parameters for stimulation of her right-sided GPi: 10 V, 1000 μsec and 50 Hz. I agree that dystonia patients may need higher stimulation current in the GPi than PD patients. However, there are limits: anyone who has stimulated the GPi of a patient with energies half of those published above will agree that there is no way that the stimulating contact in the case of Kumar et al’s patient is in the posteroventral GPi and delivering this extremely high electrical current. Either the active contact in this case report lies in some CSF space, or the pulse generator is actually not delivering the above stated energy. Interestingly, the contralateral pallidum of Kumar et al’s patient needed only 3 V43 Leksell’s non-microelectrode-guided pallidotomies, performed in the 1950s, when modern imaging techniques were lacking, resulted in 0% mortality in the 81 patients reported by Svennilson et al44 in 1960. Laitinen’s non-microelectrode-guided pallidotomies performed on a large number of patients between 1985 and 1995 had 0% mortality and a very low rate of severe morbidity.45 In two publications on microelectrode-guided pallidotomies, a high rate of mortality and severe morbidity were reported. In 1998, Brain published a detailed article presenting the pallidotomy experience in 26 patients in London.15 In this study, the severe morbidity amounted to 15.4% and mortality to 7.7% of the patients. The authors concluded their study by stating that “patient selection…. should be based largely on anticipated improvement in [levodopa-induced dyskinesias], but this must be balanced against the associated morbidity and mortality.” In 1998, Neurology published an article Chicago describing the pallidotomy experience in 26 patients.46 This study revealed a 30.7% morbidity and 3.8% mortality. The authors made the following statement concerning pallidotomy: “Morbidity may limit its use….” They concluded their article with the following sentence: “We are concerned that the procedure will gain widespread use outside of PD research centers before its efficacy and safety have been better defined.” These statements delivered from two well-known centers should have been a warning, not against pallidotomy as such (which, after all, has been performed for decades), but against the widespread use of their microelectrode technique. Two articles, both published in June 1998 and both originating from Emory University, showed variable pallidotomy complications. In the article by Cohn et al6 describing 83 patients, there were 7 patients with hemorrhage. Cohn et al wrote: “Seven patients had hemorrhage along the probe tract. Of these, three had hematomas 2 to 3 cm in size within the surgical tract in the frontal lobe and four had hemorrhage involving the ipsilateral caudate, internal capsule and putamen. Only one patient had significant mass effect….” The article by Vitek et al,5 which described 160 patients, reported “major complications” in five patients, three with hematoma: one case was aspirin-related, located “superficially in the white matter”; one was in the GPi, occurring immediately after lesioning; and one was “a subdural hematoma that presented as brain herniation the day after pallidotomy” and that eventually led to death “several months later as a result of the perioperative complications.” Concerning this last hematoma, Vitek et al wrote: “This hematoma occurred at a site distant from the area of instrumentation and was likely a result of brain shifting secondary to the craniotomy and did not result from the recording or lesioning process.” This is a peculiar statement from a neurosurgical point of view: CSF leak is known to happen during hours of recording on a supine patient, and it will lead to various grades of pneumocephalus and potential risk of subdural hematoma on either side of the head. In a letter to the editor discussing the article by Vitek et al,47 I stressed the dangers of using microelectrodes in terms of risks for provoking hematoma. In their response to this letter, Vitek et al48 wrote: “Most hemorrhages occur at the time of lesioning and represent all of our significant hemorrhage morbidity cases.” In an article published in Neurosurgery, an MER team detailed their experience in pallidotomy49 They used three to five microelectrode tracks and lesions with 1×3 mm electrodes: 36 attempts resulted in 32 pallidotomies. Three attempts were aborted (large intracerebral hematoma after the first microelectrode pass in one patient, and the GPi could not be identified in two). Additionally, the team had one unintentional lesioning of the subthalamic nucleus. Five of the 11 patients who were considered to be nonresponders (i.e., 15.5% of all pallidotomies) “had small or improperly placed lesions despite extensive use of microelectrode mapping…”.49 Linazasoro et al50 reported severe hematoma in 7.4% of their first 27 patients operated on with microelectrode pallidotomy; they stated that “the major risk is intracerebral hematoma.” One year later, the same group found that “intraoperative microerecording is considered the best method to avoid side effects and partial results.”51 Obeso et al52 defined the risks of microelectrode-guided pallidotomy in 1997 as follows: “The risks amount to 1% in mortality and 2–6% in severe morbidity-hemiplegia.”52 In an article entitled “The Pallidotomy Debate,” Carrol et al53 compared complications reported in five publications on microelectrode-guided pallidotomy with complications from eight publications on macroelectrode-guided pallidotomies. They showed that intracerebral hemorrhage occurred in 7% of MER-operated patients versus 0.6% in non-MER-operated patients. However, these results were questioned by Vitek et al,48 who wrote: “We do not believe that it is scientifically sound to determine complications of this or any other technique by simply adding up multiple reports.” Compared with the results of MER groups, the reported complications of macroelectrode teams were generally milder, the occurrence of hematoma was extremely rare, and the mortality was virtually nil.38,45,54–59 It must be noted, however, that articles by macrostimulation teams are not as frequent as those by MER teams, and therefore MRI figures of nonmicroelectrode pallidotomies or DBS electrodes are not seen as often in the literature. Hariz and DeSalles57 published an article on the complications of pallidotomy and acknowledged malplacement in some of their macrostimulation-guided lesions. The Harvard group reported on successful repeat surgery using macrostimulation on patients in whom previous macrostimulation-guided pallidotomies done elsewhere resulted in nonaccurately placed lesions.60 In all but one61 of the publications of nonmicroelectrode teams were there any deaths related to surgery. A meta-analysis study of complications of micro- versus macroelectrode-guided surgery is yet to be done. It would probably substantiate what has already been shown in the multicenter study of pallidal and subthalamic DBS,62 that the number of hematomas was significantly more frequent in patients who had had microelectrode exploration than in those operated on with macrostimulation guidance. DBS procedures have gained popularity largely because they are considered safer for patients.61,63–65 Because ablative lesions may result in permanent complications, and because some authors consider that most hemorrhages occur at the time of lesioning,48 DBS theoretically should eliminate the risk for severe morbidity. At the meeting of the Movement Disorder Society in New York in October 1998, the preliminary results of a multicenter study on DBS in advanced PD were presented. Anthony Lang66 presented the results of DBS in pallidum in 36 patients (25 bilateral) and reported, among other complications, 28% system complications, 8% hemorrhage, 11% infection, and 3% seizures. He concluded by stating that complications were not uncommon but that risk:benefit was quite acceptable. Obeso67 presented the results of DBS in the subthalamic nucleus in 36 patients (33 bilateral) and reported 8% hemorrhage and 22% infections. He concluded that the risk cannot be minimized but that the benefit is substantial. All centers involved at that time in these studies except one in Lund, Sweden, used MER techniques for target identification. The non-MER center of Lund, however, had neither hematoma nor infections among their operated DBS patients (Dr. Stig Rehncrona, Department of Neurosurgery, Lund, Sweden, personal communication). This was confirmed later on in an article in the New England Journal of Medicine, which reported on the outcomes of subthalamic and pallidal DBS in 18 centers.62 The study group found that centers using MER had more hemorrhages than those not using MER. In contrast, Benabid et al68,69 have been using MER regularly in their practice since 1987. They reported a very low rate of serious complications in their experience of 311 electrode implantations in 199 patients, most of whom had a Vim thalamic stimulation. This team reported their experience with 125 subthalamic DBS patients and found 6.4% with infections and 2.4% with intracerebral hematomas.69 Perhaps their technique, using five parallel microelectrode tracks, no intersecting passes, and a plug in the burr hole to avoid CSF leaks during the lengthy surgery on supine patients, contributed to the low rate of permanent complications. However, the rate of transcient confusion was not rare, probably reflecting, in my opinion, both the length of surgery and the edema and minor hematomas provoked by the transdural passage of five guiding cannulae through the frontal lobes bilaterally. A radiologist group in Philadelphia published an MRI showing what a brain looked like following an MER-guided pallidotomy70 One could clearly see in that illustration a wide track of massive frontal edema extending from the cortex to the pallidal area, with bleeding along the track, in the putamen, in the head of the caudate nucleus, and elsewhere. Keeping in mind that the aim of the MER-guided surgery was to place an accurate lesion in the posteroventral pallidum precisely to avoid damage to surrounding brain structures, the edema made it appear as if this patient had had an extended frontal leucotomy in one hemisphere. The complications of DBS that are related to the hardware and surgical procedure have been alarmingly high in some microelectrode centers. Whereas Oh et al71 in Toronto published an incidence of 25% device-related complications in their first 100 DBS, Lyons et al72 in Kansas presented a rather high rate of complications in their total DBS experience in 206 patients who underwent 275 DBS procedures, amounting to a rate of 49% in their specific subthalamic DBS experience on 29 patients.73 Although these complications are all related one way or another to the implant and to the learning curve, the surgical technique used, relying on lengthy MER exploration, should not be neglected, especially with respect to the high rate of infections. Hariz et al74 published a series of 58 DBS patients, including the first “learning curve” cases, and ended up with a rate of 8.6% of “device-related complications” (fracture of electrode, dislocation, and erosion), but with a 0% rate of infection or hematoma or death. This team is certainly no better surgically than others, but their surgery is based on good stereotactic imaging coupled with experienced macrostimulation, rendering a surgical time of a couple of hours for unilateral DBS and maximum twice as long time for a bilateral pocedure. In a comment on the study by Tsao et al,7 Bakay1 wrote that “most anatomic advocates fail to use standardized parkinsonian evaluation testing and rarely use a movement disorder specialist.” Although it may be true that the pioneers of modern, non-MER-guided pallidotomy did not “use” movement disorder neurologists and established outcome scales in their early publications37 (the “established” United Parkinson’s Disease Rating Scale (UPDRS) scale was first published in 1987, and the pioneers of the modern pallidotomy started to reintroduce this surgery in January 1985), this is not the case anymore, neither in their publications nor in publications from other non-MER groups.38,55,56,58,59,61,75–77 Furthermore, the use of scales and movement disorder neurologists, in itself, is no guarantee that results will be more truthfully reported. Finally, it has indeed been stated by prominent movement disorder neurologists who assessed pallidotomy effects that “large changes in motor function in specific tasks or specific parts of the body can be concealed if the usual Parkinson’s disease rating scales are employed.”78 There are published reports by most recognized movement disorder neurologists showing a virtual cure from Parkinson’s disease in Fazzini et al79 reported on 4-year follow-up in five patients operated on with microelectrode-guided unilateral pallidotomy. The preoperative UPDRS score in one of these patients was 43; 4 years after surgery, this patient had a UPDRS score of 0. Another patient’s UPDRS score dropped from 63 preoperatively to 64 years later. On the whole, Fazzini et al found an 80% improvement in their patients 4 years after surgery. In contrast, Baron et al80 reported a 7% improvement in UPDRS scores in 10 patients 4 years after surgery.80 The non-MER group of Vancouver published recently a 2-year reassessment of their pallidotomy patients, showing that there still was improvement in tremor and dyskinesias while the initial improvement in other symptoms had disappeared.81 Table 21-1 shows that the results of well-conducted studies54,56,58,59,77,82–86 on pallidotomy did not differ significantly according to the use or nonuse of MER techniques. Regardless of what surgical technique had been used, the most consistent finding in the literature is that pallidotomy exerts its main effect on limb dyskinesias, dystonia, and tremor, and least on axial symptoms and gait freezing. Although the percentages of improvement in various aspects of UPDRS reported in the literature were rather disparate, this disparity was not between reports from MER groups and non-MER groups, but within either group, as has been shown by Starr et al87 in their comprehensive survey on the effect of unilateral pallidotomy, as shown in Table 21-1.
Is MER Necessary in Movement Disorder Surgery? The Case Against
MARWAN I HARIZ
Arguments in Favor of MER
Accuracy of Location of MER-Guided Stereotactic Lesions
Accuracy of Location of MER-guided DBS Electrodes
Size of the Lesions
Electrical Parameters of DBS
Surgical Risks and Complications of MER-Guided Pallidotomy
Surgical Risks and Complications of MER-Guided DBS
Results of Surgery
Technique | Author/Year | No. of Patients | % Reduction | Reference |
---|---|---|---|---|
Micro | Kopyov et al/1997 | 29 | 25 | 82 |
Micro | Uitti et al/1998 | 41 | 21 | 83 |
Micro | Kumar et al/1998 | 39 | 31 | 84 |
Micro | Melnick et al/1999 | 29 | 16 | 85 |
Micro | Lai et al/2000 | 89 | 35 | 86 |
Macro | Giller et al/1998 | 47 | 31 | 54 |
Macro | Masterman/1998 | 32 | 24 | 77 |
Macro | Kondziolka et al/1999 | 58 | 23 | 56 |
Macro | Eskandar et al/2000 | 68 | 20 | 59 |
Macro | de Bie et al/2001 | 32 | 26 | 58 |
Macro, macroelectrode; Micro, microelectrode
“% Reduction” indicates the percentage in reduction of United Parkinson’s Disease Rating Scale off-medication motor scores. “Reference” indicates note number in References list.
Macroelectrode or Microelectrode?
The numerous examples about misplaced lesions, mislocated DBS electrodes, and complications from the literature presented in this chapter are not casual anecdotal or coincidental reports. They have not occurred once or twice, but too many times and in too many centers not to be considered evident reality; “scientifically sound”48 or not, these examples are still a reality. Therefore, they should be taken seriously and should raise serious questions about the place of MER technique and about the application and interpretation of this technique in movement disorder surgery.
This does this mean that MER techniques are to be condemned. I believe that MER is an exquisite method for studying the cellular activity of basal ganglia in several diseases and the effect of a treatment. Especially in animal models this technique is irreplaceable in learning more about the behavior of the basal ganglia. What is questionable is the efficacy and safety of the routine application of this technique in humans; furthermore, it is dubious whether this technique should be imposed on, or practiced by teams who have neither the training nor the experimental background to be able to use this technique in a way that is safe for patients. Additionally, for teams who have the experimental background of MER as used in animal models, it is important to keep in mind the tremendous difference there is between performing several exploratory tracks on a monkey brain and doing the same on a patient to obtain the “optimal” location of a lesion, a location that is questionable, as has been shown previously. What is not questionable, however, is the increased risks for the patient because of this technique. The high rate of death is totally unacceptable in a surgery that is elective, symptomatic, not life-saving, and minimally invasive, and the aim of which is to improve the life quality of the patient. I personally prefer to have a suboptimally located or suboptimally sized lesion or even no lesion at all in a patient who may not have benefited adequately from surgery than to have a very well located lesion or DBS electrode in a comatose, hemiplegic, or dead patient. The primum nil nocere axiom of functional stereotactic surgery should always prevail when operating on a patient with a nonmortal disease such as essential tremor or PD.
Macroelectrode technique, like MER techniques, needs proper tools and training to be safe and efficient. Macroelectrode technique also has its proper requirements that should be fulfilled by those using it. For example, one must have a reliable and accurate stereotactic image showing the detailed anatomy of the target. This is possible for the pallidum and subthalamic nucleus, where proper imaging sequences can show the details of the target and its surroundings.88,89 One must also have a reliable and accurate stereotactic frame with distortion-free fiducials that do not lie far from the head at the extreme periphery of the MRI. One should have a well-calibrated radio frequency machine with good discriminative impedance readings to allow proper discrimination between gray matter, white matter, and CSF along the path of the probe toward the target. To ascertain an acceptable location-specificity of stimulation enabling proper and precise interpretation of responses to stimulation, the radio frequency electrode should have a tip that is neither longer nor wider than 2 mm. Last but not least, the surgeon should have a good analytic knowledge about the anatomy and electrophysiology of the target and its surrounding structures, as well as personal experience in interpreting the stimulation responses. Provided these requirements, the advantages of macroelectrode-guided surgery over MER will be less confusion, less brain edema, less hemorrhage, and fewer severe complications; the patient will remain reliable and readily assessable during surgery; surgery will be faster and less expensive, with fewer infections; and the patient will require a shorter stay in the hospital. Table 21-2 lists some of the characteristics of macro- versus microelectrodes in movement disorder surgery.
Macro | Micro | |
---|---|---|
Safety for patient | Very high | High |
Speed of exploration | Rapid | Slow |
Needs several tracks | Seldom | Always |
Patient cooperation | Needed | Not needed |
Robustness at repeat use | Excellent | Poor |
Technical problems | Very rare | Not infrequent |
Sophistication | Low | High |
Detection of somatotopy | Good | Very good |
Need for dedicated personnel | No | Yes |
Costs | Low | High |
Mandatory in MD surgery | Yes | No |
Conclusion
MER technique is important for research and the detailed cellular study of basal ganglia, but it may increase surgical risks: prolonged surgery may increase stress reactions, and the patient may become unreliable to assess; additionally, prolonged surgery may increase infection risk, and the sharp probe tip and multiple passes may increase the risk of hemorrhage. So far there is no evidence that microelectrode technique improves results, but there is accumulating evidence that it increases the rate of severe complications (death, hemorrhage). In the absence of a randomized trial on the use of macrostimulation versus MER techniques in movement disorder surgery, a comprehensive and critical review of the literature remains the only way to evaluate the benefits and drawbacks of either method.
The available literature clearly suggests that MER neither decreases risks nor increases targeting accuracy of ablative surgery or DBS procedures, compared with macrostimulation techniques. MER does not result in smaller lesions or in lower electrical parameters of DBS compared with macrostimulation techniques. The surgical results of pallidotomy reported by MER supporters seem not to be different from those of non-MER groups, in terms of impact of pallidotomy on patients’ symptoms or duration of benefit.
MER techniques are most appropriate for the exploration of the basal ganglia and the thalamus, but not for routine procedures in the widespread practice of pallidotomy and DBS. Patients undergoing microelectrode-guided pallidotomy or DBS should be informed of, and consent to, the additional risks involved with MER, compared with methods based on modern, accurately performed, stereotactic target imaging, in combination with experienced intraoperative macrostimulation.
In 1998 Bakay1 was right when he wrote that “good stereotactic surgery for movement disorders can be performed with or without the microelectrode, and poor surgical results can occur both with and without the microelectrode.” A fundamental controversy remains, however: what are the respective proportions of “poor results” for each method? I believe that a fundamental axiom should remain permanently present in the mind of anybody performing functional neurosurgery for movement disorders, especially if MER is used: movement disorder surgery is above all a primum nil nocere procedure.90
REFERENCES
- Bakay RAE. Comments. Neurosurgery. 1998;43:513.
- Tasker RR, Organ LW, Hawrylyshyn PA. The Thalamus and Midbrain of Man: A Physiological Atlas Using Electrical Stimulation. Springfield, IL: Charles C Thomas; 1982.
- Tasker R. Thalamotomy. Neurosurg Clin N A. 1990;1(4):841–864.
- Levy R, Dostrovsky JO, Lang AE, Sime E, Hutchison WD, Lozano AM. Effects of apomorphine on subthalamic nucleus and globus pallidus internus neurons in patients with Parkinson’s disease. J Neurophysiol. 2001;86:249–260.
- Vitek JL, Bakay RA, Hashimoto T, et al. Microelectrode-guided pallidotomy: Technical approach and its application in medically intractable Parkinson’s disease. J Neurosurg. 1998;88:1027–1043.
- Cohn MC, Hudgins PA, Sheppard SK, Starr PA, Bakay RA. Pre-and postoperative MR evaluation of stereotactic pallidotomy. Am J Neuroradiol. 1998;19:1075–1080.
- Tsao K, Wilkinson S, Overman J, Koller WC, Batnitzky S, Gordon MA. Pallidotomy lesion locations: Significance of microelectrode refinement. Neurosurgery. 1998;43:506–513.
- DeLong MR, Vitek JL, Sheppard RS, et al. Comparison of physiological mapping, magnetic resonance imaging, and histologic lesion in a patient who underwent microelectrode-guided pallidotomy for Parkinson’s disease. Ann Neurol. 1995;38:298.
- Hariz MI, Fodstad H. The pallidotomy debate: Are microelectrode-guided pallidotomies always located in the pallidum? [letter to the editor]. Br J Neurosurg. 1998;12:595–597.
- Dogali M, Fazzini E, Kolodny E, et al. Stereotactic ventral pallidotomy for Parkinson’s disease. Neurology. 1995;45:753–761.
- Dogali M, Sterio D, Fazzini E, Kolodny E, Eidelberg D, Beric A. Effects of posteroventral pallidotomy on Parkinson’s disease. In: Battistin L, Scarlato G, Caraceni T, Ruggieri S, eds. Advances in Neurology. Vol 69. Philadelphia: Lippincott-Raven; 1996:585–590.
- Beric A, Sterio D, Dogali M, Alterman R, Kelly P. Electrical stimulation of the globus pallidus preceding stereotactic posteroventral pallidotomy. Stereotact Funct Neurosurg. 1996;66:161–169.
- Lozano AM, Kumar R, Gross RE, et al. Globus pallidus internus pallidotomy for generalized dystonia. Mov Disord. 1997;12:865–870.
- Ondo WG, DeSaloms M, Jancovic J, Grossman RG. Pallidotomy for generalized dystonia. Mov Disord. 1998;13:693–698.
- Samuel M, Caputo E, Brooks DJ, et al. A study of medial pallidotomy for Parkinson’s disease: Clinical outcome, MRI location and complications. Brain. 1998;121:59–75.
- Gross RE, Lombardi WJ, Lang AE, et al. Relationship of lesion location to clinical outcome following microelectrode-guided pallidotomy for Parkinson’s disease. Brain. 1999;122:405–416.
- Trepanier LL, Kumar R, Lozano AM, Lang AE, Saint-Cyr JA. Neuropsychological outcome of GPi pallidotomy and GPi or STN deep brain stimulation in Parkinson’s disease. Brain Cogn. 2000;42:324–347.
- Lombardi WJ, Gross RE, Trepanier LL, Lang AE, Lozano AM, Saint-Cyr JA. Relationship of lesion location to cognitive outcome following microelectrode-guided pallidotomy for Parkinson’s disease: Support for the existence of cognitive circuits in the human pallidum. Brain. 2000;123:746–758.
- Lozano AM, Hutchison WD, Tasker RR, Lang AE, Junn F, Dostrovsky JO. Microelectrode recordings define the ventral posteromedial pallidotomy target. Stereotact Funct Neurosurg. 1998;71:153–163.
- Kirschman DL, Milligan B, Wilkinson S, et al. Pallidotomy microelectrode targeting: Neurophysiology-based target refinement. Neurosurgery. 2000;46:613–624.
- Cooper IS. The Neurosurgical Alleviation of Parkinsonism. Springfield, IL: Charles C Thomas; 1956.
- Sterio D, Beric A, Dogali M, Fazzini E, Alfaro G, Devinsky O. Neurophysiological properties of pallidal neurons in Parkinson’s disease. Ann Neurol. 1994;35:586–591.
- Lozano AM, Hutchison WD, Dostrovsky JO. Microelectrode monitoring of cortical and subcortical structures during stereotactic surgery. Acta Neurochir Suppl (Wien). 1995;64:30–34.
- Taha JM, Favre J, Baumann TK, Burchiel KJ. Characteristics and somatotopic organization of kinesthetic cells in the globus pallidus of patients with Parkinson’s disease. J Neurosurg. 1996;85:1005–1012.
- Guridi J, Gorospe A, Ramos E, Linazasoro G, Rodriguez MC, Obeso JA. Stereotactic targeting of the globus pallidus internus in Parkinson’s disease: Imaging versus electrophysiological mapping. Neurosurgery. 1999;45:278–287.
- Uitti RJ, Obwegeser AA, Lucas JA, et al. Pallidotomy location and correlation with motor, speech and neuropsychological outcome. Mov Disord. 2000;15:1039.
- Krauss JK, Desaloms JM, Lai EC, King DE, Jancovic J, Grossman RG. Microelectrode-guided posteroventral pallidotomy for treatment of Parkinson’s disease: Postoperative magnetic resonance imaging analysis. J Neurosurg. 1997;87:358–367.
- Koller W, Pahwa R, Busenbark K, et al. High frequency unilateral thalamic stimulation in the treatment of essential and parkinsonian tremor. Ann Neurol. 1997;42:292–299.
- Germano IM. Chronic deep brain stimulation: Indications and techniques. In: Germano IM, ed. Neurosurgical Treatment of Movement Disorders. Park Ridge, IL. American Association of Neurological Surgeons; 1999:159–167.
- Damier P, Bejjani BP, Houeto Y, Agid Y. Basis and treatment of a failure of subthalamic stimulation. Parkinsonism Relat Disord. 1999;5(suppl):S100–S101.
- Pidoux B, Houeto J-L, Welter M-L, et al. Is electrophysiological monitoring mandatory during stereotactic surgery for subthalamic deep brain stimulation in Parkinson’s disease? Neurology. 2000;54(suppl 3):A282–A283.
- Levesques MF, Taylor S, Rogers R, Le MT, Swope D. Subthalamic stimulation in Parkinson’s disease, preliminary results. Stereotact Funct Neurosurg. 1999;72:170–173.
- Bronte-Stewart HM, Hill BC, McGuire K, Minn AY, Courtney TA, Heit G. Superior outcomes of bilateral STN DBS in IPD attributed to precise intraoperative localization techniques. Neurology. 2001;56(suppl 3):A279.
- Alterman RL, Sterio D, Beric A, Kelly PJ. Microelectrode recording during posteroventral pallidotomy: Impact on target selection and complications. Neurosurgery. 1999;44:315–323.
- Young RF, Shumway-Cook A, Vermeulen SS, et al. Gamma Knife radiosurgery as a lesioning technique in movement disorder surgery. J Neurosurg. 1998;89:183–193.
- Obeso JA, Guridi J, Alvarez L, Macias R, Linazasoro G. Ablative surgery for Parkinson’s disease. In: Jancovic J, Tolosa E, eds. Parkinson’s Disease and Movement Disorders. 3rd ed. Baltimore: Williams & Wilkins; 1998:1049–1064.
- Laitinen LV, Bergenheim AT, Hariz MI. Leksell’s posteroventral pallidotomy in the treatment of Parkinson’s disease. J Neurosurg. 1992;76:53–61.
- Johansson F, Malm J, Nordh E, Hariz M. Usefulness of pallidotomy in advanced Parkinson’s disease. J Neurol Neurosurg Psychiatry. 1997;62:125–132.
- Hariz MI, Hirabayashi H. Any relation between size and site of the stereotactic lesion and symptomatic results of pallidotomy and thalamotomy? Stereotact Funct Neurosurg. 1997;69:28–45.
- Ondo W, Jancovic J, Schwartz K, Almaguer M, Simpson RK. Unilateral thalamic deep brain stimulation for refractory essential tremor and Parkinson’s disease tremor. Neurology. 1998;51:1063–1069.
- Charles PD, Maciunas RJ, Davis TL, et al. Thalamic stimulation: An innovative treatment for tremor. Tenn Med. 1997;90:275–277.
- Limousin P, Speelman JD, Gielen F, Janssens M, and study collaborators. Multicentre European study of thalamic stimulation in parkinsonian and essential tremor. J Neurol Neurosurg Psychiatry. 1999;66:289–296.
- Kumar R, Dagher A, Hutchison WD, Lang AE, Lozano AM. Globus pallidus deep brain stimulation for generalized dystonia: Clinical and PET investigation. Neurology. 1999;53:871–874.
- Svennilson E, Torvik A, Lowe R, Leksell L. Treatment of parkinsonism by stereotactic thermolesions in the pallidal region: A clinical evaluation of 81 cases. Acta Psychiatr Neurol Scand. 1960;35:358–377.
- Laitinen LV. Pallidotomy for Parkinson’s disease. Neurosurg Clin North Am. 1995;6:105–112.
- Shannon KM, Penn RD, Kroin JS, et al. Stereotactic pallidotomy for the treatment of Parkinson’s disease: Efficacy and adverse effects at 6 months in 26 patients. Neurology. 1998;50:434–438.
- Hariz MI, Bergenheim AT, Fodstad H. Crusade for microelectrode guidance in pallidotomy [letter to the editor]. J Neurosurg. 1999;90:175–177.
- Vitek JL, Bakay RAE, DeLong MR. Response. J Neurosurg. 1999;90:177–179.
- Van Horn G, Hassenbusch SJ, Zouridakis G, Mullani NA, Wilde MC, Papanicolaou AC. Pallidotomy: A comparison of responders and nonresponders. Neurosurgery. 2001;48:263–271.
- Linazasoro G, Guridi J, Gorospe A, Ramos E, Mozo A, Obeso JA. Posteroventral pallidotomy in Parkinson’s disease: Clinical results in 27 patients. Mov Disord. 1996;11(suppl 1):240.
- Linasazoro G, Guridi J, Vela L, et al. Stereotactic surgery in Parkinson’s disease. Neurologia. 1997;12:343–353.
- Obeso JA, Rodriguez MC, Gorospe A, Guridi J, Alvarez L, Macias R. Surgical treatment of Parkinson’s disease. Baillieres Clin Neurol. 1997;6:125–145.
- Carrol CB, Scott R, Davies LE, Aziz T. The pallidotomy debate. Br J Neurosurg. 1998;12:146–150.
- Giller CA, Dewey RB, Ginsburg MI, Mendelsohn DB, Berk AM. Stereotactic pallidotomy and thalamotomy using individual variations of anatomic landmarks for localisation. Neurosurgery. 1998;42:56–65.
- Kishore A, Turnbull IM, Snow BJ, et al. Efficacy, stability and predictors of outcome of pallidotomy for Parkinson’s disease: Six-month follow-up with additional 1-year observations. Brain. 1997;120:729–737.
- Kondziolka D, Bonaroti E, Baser S, Brandt F, Kim YS, Lunsford LD. Outcomes after stereotactically guided pallidotomy for advanced Parkinson’s disease. J Neurosurg. 1999;90:197–202.
- Hariz MI, DeSalles AAF. The side-effects and complications of posteroventral pallidotomy. Acta Neurochir Suppl (Wein). 1997;68:42–48.
- de Bie RM, Schuurman PR, Bosch DA, de Haan RJ, Schmand B, Speelman JD. Outcome of unilateral pallidotomy in advanced Parkinson’s disease: Cohort study of 32 patients. J Neurol Neurosurg Psychiatry. 2001;71:375–382.
- Eskandar EN, Shinobu LA, Penney JB Jr, Cosgrove GR, Counihan TJ. Stereotactic pallidotomy performed without using microelectrode guidance in patients with Parkinson’s disease: Surgical technique and 2-year results. J Neurosurg. 2000;92:375–383.
- Eskandar EN, Cosgrove GR, Shinobu LA, Penney JB Jr. The importance of accurate lesion placement in posteroventral pallidotomy: Report of two cases. J Neurosurg. 1998;89:630–634.
- Schuurman PR, Bosch DA, Bossuyt PM, et al. A comparison of continuous thalamic stimulation and thalamotomy for suppression of severe tremor. N Engl J Med. 2001;342:461–468.
- APD Study Group. Deep brain stimulation (DBS) of the subthalamic nucleus or globus pallidus pars interna in Parkinson’s disease. N Eng J Med. 2001;345:956–963.
- Benabid AL, Pollak P, Gao D, et al. Chronic electrical stimulation of the ventralis intermedius nucleus of the thalamus as a treatment of movement disorders. J Neurosurg. 1996;84:203–214.
- Tasker RR. Deep brain stimulation is preferable to thalamotomy for tremor suppression. Surg Neurol. 1998;49:145–154.
- Speelman JD, Schuurman PR, de Bie RMA, Bosh DA. Thalamic surgery and tremor. Mov Disord. 1998;13:103–106.
- Lang A, for the Deep Brain Stimulation in Advanced Parkinson’s Disease Study Group. Deep brain stimulation (DBS) of the globus pallidus internus (GPi) in advanced Parkinson’s disease (PD). Mov Disord. 1998;13(suppl 2):264.
- Obeso JA, for the Deep Brain Stimulation in Advanced Parkinson’s Disease Study Group. Deep brain stimulation (DBS) of the subthalamic nucleus (STN) in advanced Parkinson’s disease (PD) Mov Disord. 1998;13(suppl 2):303.
- Pollak P, Benabid A-L, Krack P, Limousin P, Benazzouz A. Deep brain stimulation. In: Jancovic J, Tolosa E, eds. Parkinson’s Disease and Movement Disorders. 3rd ed. Baltimore: Williams & Wilkins; 1998:1085–1101.
- Benabid AL, Koudsie A, Fraix V, et al. High-frequency stimulation of the subthalamic nucleus in advanced Parkinson’s disease: An 8-year experience. J Neurosurg. 2001;94:376A.
- Friedman DP, Goldman HW, Flanders AE. MRI of stereotaxic pallidotomy and thalamotomy. Am J Roentgenol. 1997;169(3):894–896.
- Oh MY, Kim SH, Lozano AM. Hardware-related complications of deep brain stimulation in 100 consecutive electrodes. J Neurosurg. 2001;94:412A.
- Lyons KE, Koller WC, Wilkinson SB, Pahwa R. Surgical and device-related events with deep brain stimulation. Neurology. 2001;56(suppl 3):A147.
- Pahwa R, Lyons KE, Wilkinson SB, Koller WC. One-year follow-up of bilateral subthalamic stimulation in Parkinson’s disease. Neurology. 2001;56(suppl 3):A146.
- Hariz MI, Shamsgovara P, Johansson F, Hariz G-M, Fodstad F. Tolerance and tremor-rebound following long-term chronic thalamic stimulation for parkinsonian and essential tremor. Stereotact Funct Neurosurg. 1999;72:208–218.
- Scott R, Gregory R, Hines N, et al. Neuropsychological, neurological and functional outcome following pallidotomy for Parkinson’s disease: A consecutive series of eight simultaneous bilateral and twelve unilateral procedures. Brain. 1998;121:659–675.
- Counihan TJ, Shinobu LA, Eskandar EN, Cosgrove GR, Penney JB Jr. Outcomes following staged bilateral pallidotomy in advanced Parkinson’s disease. Neurology. 2001;56:799–802.
- Masterman D, DeSalles A, Baloh RW, et al. Motor, cognitive, and behavioral performance following unilateral ventroposterior pallidotomy for Parkinson disease. Arch Neurol. 1998;55:1201–1208.
- Obeso JA, Linazasoro G, Rothwell JC, Jahanshahi M, Brown R. Assessing the effects of pallidotomy in Parkinson’s disease. Lancet. 1996;347:1490.
- Fazzini E, Dogali M, Sterio D, Eidelberg D, Beric A. Stereotactic pallidotomy for Parkinson’s disease: A long-term follow-up of unilateral pallidotomy. Neurology. 1997;48:1273–1277.
- Baron MS, Vitek JL, Bakay RAE, DeLong MR. Treatment of advanced Parkinson’s disease by unilateral posterior GPi pallidotomy: 4-years results of a pilot study. Movement Disorders. 1998;(suppl 2):263.
- Samii A, Turnbull IM, Kishore A, et al. Reassessment of unilateral pallidotomy in Parkinson’s disease: A 2-year follow-up study. Brain. 1999;122:417–425.
- Kopyov O, Jacques D, Duma C, et al. Microelectrode-guided posteroventral medial radiofrequency pallidotomy for Parkinson’s disease. J Neurosurg. 1997;87:52–59.
- Uitti RJ, Wharen RE Jr, Turk MF. Efficacy of levodopa therapy on motor function after posteroventral pallidotomy for Parkinson’s disease. Neurology. 1998;51:1755–1757.
- Kumar R, Lozano AM, Montgomery E, Lang AE. Pallidotomy and deep brain stimulation of the pallidum and subthalamic nucleus in advanced Parkinson’s disease. Mov Disord. 1998;13(suppl 1):73–82.
- Melnick ME, Dowling GA, Aminoff MJ, Barbaro NM. Effect of pallidotomy on postural control and motor function in Parkinson disease. Arch Neurol. 1999;56:1361–1365.
- Lai EC, Jankovic J, Krauss JK, Ondo WG, Grossman RG. Long-term efficacy of posteroventral pallidotomy in the treatment of Parkinson’s disease. Neurology. 2000;55:1218–1222.
- Starr PA, Vitek JL, Bakay RA. Ablative surgery and deep brain stimulation for Parkinson’s disease. Neurosurgery. 1998;43:989–1013.
- Starr PA, Vitek JL, DeLong M, Bakay RAE. Magnetic resonance imaging-based stereotactic localization of the globus pallidus and subthalamic nucleus. Neurosurgery. 1999;44:303–314.
- Hirabayashi H, Tengvar M, Hariz MI. Stereotactic imaging of the pallidal target. Mov Dis. 2001;3(suppl):S162–S166.
- Hariz MI. Complications of movement disorder surgery and how to avoid them. In: Lozano A, ed., Progress in Neurological Surgery. Vol 15, Movement Disorder Surgery. Basel: Karger; 2000:246–265.
- Tasker RR, Organ LW, Hawrylyshyn PA. The Thalamus and Midbrain of Man: A Physiological Atlas Using Electrical Stimulation. Springfield, IL: Charles C Thomas; 1982.
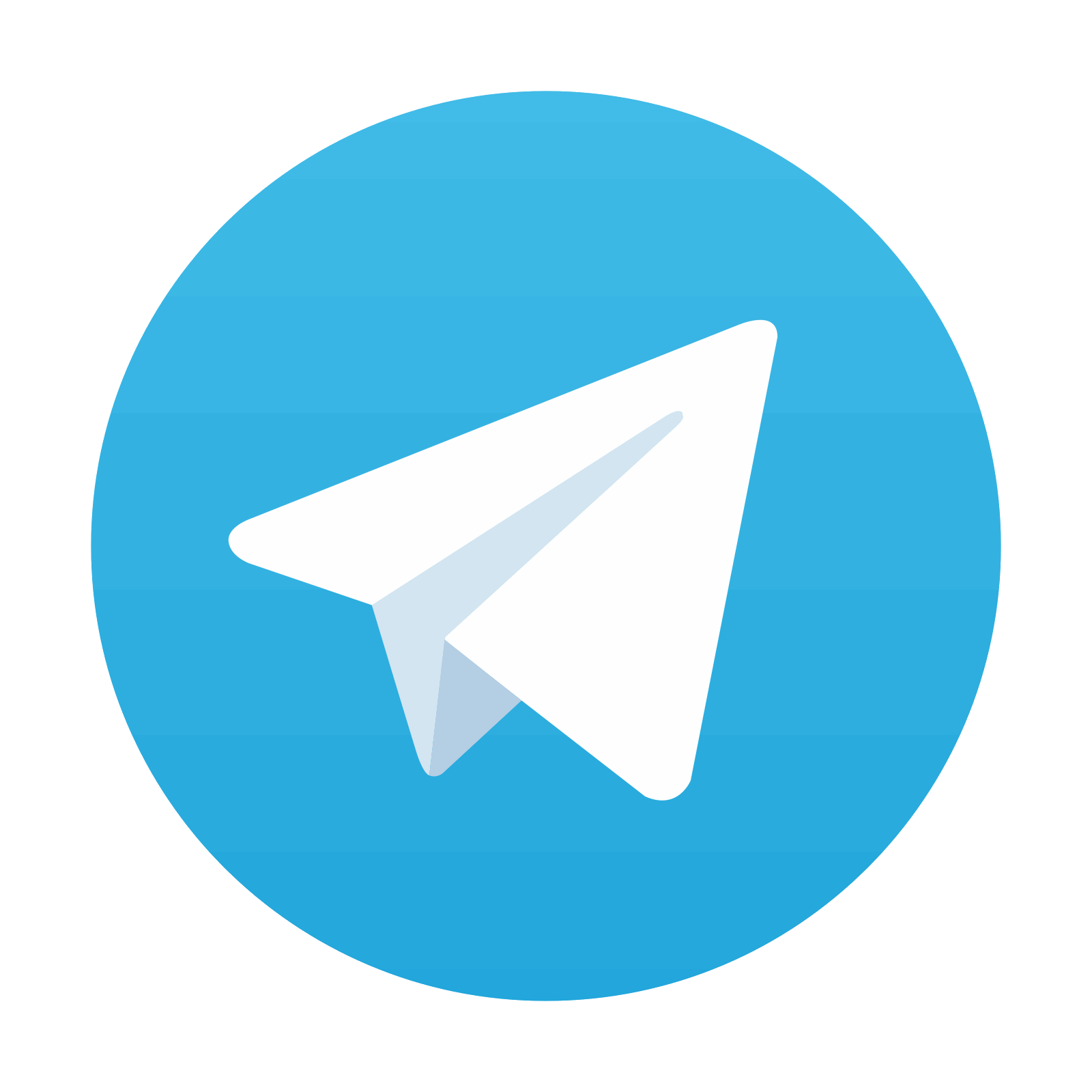
Stay updated, free articles. Join our Telegram channel

Full access? Get Clinical Tree
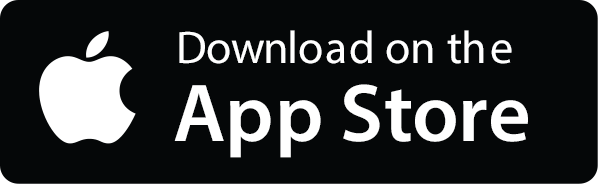
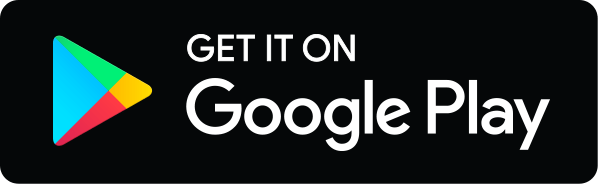