Study
Type of study
No of patients
Type of stroke
Total seizures
Early seizures
Late seizures
Epilepsy
Louis and McDowell [10]
R
1000
Isc
7.7%
3.3%
2.7%
3% early, 81% late
Black et al. [38]
R
827
Isc, hem
10%
57%a
43%a
Gupta et al. [35]
R
90
Isc
33%a
1 year 40%a
2 years 24%a
39%a
Sung et al. [36]
R
118
Isc
5.9%
11%8
66%a
35% earlya
90% latea
Lancman et al. (6)
R
230
Isc, ICH
10%
5.4%
2.7%
Shinton et al. [11]
P
230
Isc, ICH, SAH
5.7%
Kilpatrick et al. [9]
P
1000
Isc, ICH, SAH
4.4%
32% earlya
10% without earlya
Lo et al. [16]
R
1200
Isc, ICH, SAH
2.5%
Milandre et al. [12]
R
2016
Isc, ICH
3.9%
1.4%
2.5%
1.7%
Daniele et al. [40]
R
4425
Isc, ICH
Isc 4.7%
ICH 5.7%
Isc 2.6%
ICH 3.6%
Isc 2.1%
ICH 2.1%
Arboix et al. [41]
P
1099
Isc, ICH, SAH
2.5%
Bladin et al. [7]
P
1897
Isc, ICH
Isc 8.6%
ICH 10.6%
Isc 4.8%
ICH 7.9%
Isc 3.8%
ICH 2.6%
Isc 2.1%
ICH 2.6%
Vespa et al. [50]
P
109
Isc, ICH
Isc 6%
ICH 28%
So et al. [54]
R
535
Isc
6%
1 year 3%
5 year 7.4%
10 year 8.9%
Giroud et al. [5]
P
1640
Isc, ICH, SAH
Ath 4%
Card 16.6%
Lac 1%
TIA 1.7%
5.4%
Berges et al. [24]
P
3205
Isc, hem
5%
1.8%
3.2%
50%b
Burn et al. [8]
P
675
Isc, ICH, SAH
Isc 4.2%/9.7%
ICH 19.9%/26.1%
SAH 22%/34.3%
2%
P
1195
Isc, ICH
4.1%
Isc 3%
ICH 8%
3.2%
Labovitz et al. [56]
P
904
Isc, ICH, SAH
Isc 3.1%
ICH 7.3%
SAH 8%
Devuyst et al. [57]
P
3628
Isc, ICH
1.02%
Isc 0.7%c
ICH 3.97%c
Neau et al. [113]e
R
65
Isc
10.8%
Lamy et al. [22]f
P
581
Isc
2.4%
1 year 3.1%
3 years 5.5%
2.3%
Cheung et al. [42]
R
994
Isc, ICH, SAH
3.4%
Isc 3.3%
ICH 4.1%
SAH 0%
0.7%
Cordonnier et al. [116]
P
202
Isc, ICH
5.4%
Isc 4.5%
ICH 12%
6.9%
Isc 6.8%
ICH 8%
Lossius et al. [43]
P
484
ICH
5.7%
Benbir et al. [45]
P
1428
Isc, ICH, CVT
3.6%
Isc 2.7%
ICH 12.8%
CVT 26.6%
Misirli et al., [46]
R
1880
Isc, ICH
10.6%
4.1%
6.5%
Szaflarski et al. [58]
P
6044
Isc, ICH, SAH
3.1%
3.1%
Isc 2.4%
ICH 7.9%
SAH 10.1%
Basic-Baronica et al. [47]
R
3542
Isc, ICH
1.8%
Isc 1.43%
ICH 3.76%
Alberti et al. [48]
P
638
Isc, ICH
4.9%
Isc 4.8%
ICH 16.3%
Chiang et al. [51]
R
143
Isc
9.1%
Strzelczyk et al. [136]
P
264
Isc, ICH
10.9%
4.5%
6.4%
3.8%
Denier et al. [87]
P
328
Isc
4.3%
Krakow et al. [52]
P
58,874
Isc, TIA, ICH
Isc 2.2%
TIA 0.7%
ICH 5.1%
Mecarelli et al. [99]
P
232
Isc, ICH
6.5%
Procaccianti et al. [53]
P
2053
Isc, ICH
3.2%
Couillard et al. [49]
R
400
Isc
4%
2.5%
1.5%d
Pezzini et al. [88]
P
516
Isc, ICH
3.9%
Huang et al. [21]
P
10,261
Isc
3.6%
1.53%
2.03%e
Zou et al. [14]
M
102,008
Isc, ICH
6.93%
Isc 6%
ICH 10%
5%
Status Epilepticus
SE may be an additional risk factor for increased mortality and morbidity after stroke, through systemic metabolic changes, increased risk for herniation secondary to elevated intracranial pressure, cardiac arrhythmias leading to sudden death or increased risk of aspiration pneumonia [56]. Thus SE is an unquestionable reason for ICU admission.
Acute stroke is the third most common cause for SE [59], accounting for approximately 20% of all SE cases [60–62]. In a retrospective study, 8% of all initial post-stroke seizures presented as SE (6% focal and 2% generalized) [35]. SE was more common with early (14%) than late (5%) onset seizures, but did not occur as recurrent seizures. This was demonstrated in the largest pre-CT retrospective study of seizures following non-embolic cerebral infarction, that found the majority of SE attacks to occur in the acute post-stroke phase [10]. In another retrospective study, SE occurred in 13% of 118 patients with thrombotic stroke and was generalized tonic-clonic in 60%. This latter study reported only one patient with SE as the initial stroke manifestation and another three with SE in the acute stroke phase: 60% of patients had SE as late seizure manifestation post infarction [36]. Milandre et al. found that 14% of their patients with initial post-stroke seizures were in SE [12] and Lo et al. that 10% of early seizure patients were in SE [16]. Within the first 7 days following an ischemic stroke, SE occurs in 0.9% of patients in the NOMASS-population-based study, and represents 18.9–27% of first-time or early seizures after stroke, respectively [56, 63].
A large, prospective, population-based study was conducted in Besançon, France [63]. Out of 3205 patients with first-time strokes, 159 (5%) had first-time seizures. SE occurred in 31/159 (19%) patients with post-stroke seizures. Partial SE occurred in 12 patients, NCSE in ten, generalized in six, and unclassifiable in three patients. SE occurred in 22/2742 (0.8%) patients with ischemic stroke and 22/116 (19%) patients with ischemic stroke who had post-stroke seizures. SE occurred in 9/463 (1.9%) patients with ICH and 9/43 (21%) patients with ICH and post-stroke seizures. There was no significant difference between the two etiologies of stroke (ischemic stroke or ICH) regarding the occurrence of post-stroke SE. In 4/3205 (0.12%) patients stroke initially manifested as SE and in 17/159 (11%) SE occurred as the first post-stroke epileptic symptom. In patients with SE, it manifested as the first epileptic syndrome in 17/31 (55%) patients (in 7 as “early” SE—within the first 2 weeks—and in 10 as “late” SE) and in 14 patients it followed another seizure after the stroke (in two patients it was “early” SE and in 12 patients it was “late” SE). In an average 47-month follow-up of the 16/17 surviving patients with SE as the first epileptic symptom, only 3 (19%) developed additional episodes of SE and 50% were SE or seizure-free. By contrast, all 14 patients with SE after one or more seizures had recurrences: five with SE (36%) and 9 (64%) with only seizures. Thus, SE as the first epileptic symptom was associated with a lower risk for subsequent seizures (p < 0.01). Such favorable association was not noted with early or late occurrence of SE. Fifteen out of 31 (48%) patients with post-stroke SE died. In five of them (16%, all with infarction) SE was considered the direct cause of death. There was no significant difference in mortality for patients with post-stroke seizures and post-stroke SE. Permanent neurologic deterioration after SE occurred in two patients only and transient deterioration in 13 patients. However, there was no radiological change in these 15 patients . In another large, multicenter study of 346 patients with generalized convulsive SE, mortality reached 11% in those patients with stroke as the precipitating cause [60].
Another large, retrospective hospital-based study was reported from Turkey [64]. Out of 1174 patients with first-time stroke, 180 (15.3%) developed post-stroke seizures, of which 17 (9% or 1.45% of the whole cohort) developed SE. Twelve patients manifested SE after ischemic stroke and five after ICH. There was no difference between the group with SE and the group with post-stroke seizures regarding sex, age, stroke risk factors, seizure types, EEG findings, stroke type (ischemic or hemorrhagic), topography or cortical involvement or size of the lesion. However, SE occurred more frequently among more disabled patients (Rankin scale > 3, p = 0.002). Early onset (within the first week) SE was found in 7/17 patients, of whom SE occurred as the first epileptic symptom in six (stroke begun as SE in two patients) and as SE after at least one seizure in one patient. Late-onset SE was found in 10/17 patients, of whom SE occurred as the first epileptic symptom in three. Five of seven patients with early onset SE compared to none of those patients with late-onset SE experienced recurrence of SE (p = 0.003). Mortality was not different among patients with SE (53%) compared to those with post-stroke seizures (50%). However, it was higher in those patients with early onset SE, than in those with late-onset seizures (p = 0.049). Death was the direct consequence of SE in two patients (12%). Poor functional disability was the only independent clinical factor for developing post-stroke SE and age for mortality after post-stroke SE.
In the large prospective study from Lausanne, Switzerland, SE was reported in 3/37 (8%) patients with early seizures after stroke , but in only 0.08% of all patients with ischemic infarction or ICH in this cohort [57].
In a prospective study from Turkey that evaluated 121 patients with SE, 30 (24.8%) were associated with stroke. This study does not report incidence of post-stroke SE. All stroke types were evenly distributed within the early onset group (within 2 weeks), whereas only ischemic stroke was found in the late-onset group (after 2 weeks). Posterior cerebral artery (PCA) infarcts were significantly more common within the late-onset group. NCSE was more frequent than convulsive SE in the early onset group [65].
In a retrospective study from Taiwan, 143 patients with first-time ischemic stroke were followed for a minimum of 6 years. One (0.07%) developed SE during hospitalization [51]
In a prospective study of 2053 patients admitted to a Stroke Unit, 58 (2.8%) with ischemic stroke presented with early seizures and 13 (0.6%) with SE [53].
In summary, the same methodological problems (see end of subsect. 2.1) are true for studies that report SE after stroke. From prospective studies, the best estimate is that SE occurs in 0.08–0.9% of patients after ischemic stroke .
Table 9.2 is a summary of the aforementioned studies reporting SE incidence after stroke.
Table 9.2
Studies with reported status epilepticus (SE) incidence after a stroke
Study | Type of study | No of pts | SE | Comments |
---|---|---|---|---|
Sung et al. [36] | R | 118 | 15% | 0.8% initial stroke manifestation |
Kilpatrick et al. [9] | P | 1000 | 0.4% | Pts with early seizures |
Lo et al. [16] | R | 1200 | 0.25% | Pts with early seizures |
Milandre et al. [12] | R | 2016 | 0.5% | |
Gupta et al. [35] | R | 90 | 8% | 14% of pts with early seizures 5% of pts with late seizures |
Labovitz et al. [56] | P | 904 | 0.9% | |
Rumbach et al. [63] | P | 3205 | Isc 0.9% ICH 1.9% | 0.12% initial stroke manifestation 19% pts with SE had recurrent SE, when 1st epileptic symptom 36% pts with SE had recurrent SE, when SE followed seizures |
Velioglu et al. [64] | R | 1174 | 1.45% Isc 1% ICH 0.45% | 0.17% initial stroke manifestation 71% pts with SE had recurrence, when early onset SE 0% pts with SE had recurrence, when late-onset SE |
Devuyst et al. [57] | P | 3628 | 0.08% | Excluding 6 pts with prodromal seizures |
Lamy et al. [22] | P | 581 | 0.34% | age group 18–55 years |
Chiang et al. [51] | R | 143 | 0.07% | |
Procaccianti et al. [53] | P | 2053 | Isc, ICH 0.6% |
Pathophysiology
Seizures Before Stroke: Chicken or Egg First?
Although the vast majority of seizures follow stroke, they can also precede it. Many studies excluded epileptic patients from the analysis if they suffered a stroke and had concurrent seizures. Others, however, reported the outcomes in these patients with “vascular precursor epilepsy ” [10, 36]. In one particular study from UK, 46% of patients who developed seizures after acute stroke were epileptics, raising the possibility that epileptic patients may have more frequent seizures after a stroke than non-epileptic patients [11]. This could be explained either through recurrent or continuous focal ischemia capable of inducing an epileptogenic cortical focus or the activation of epileptiform discharges by regional hypoxia in patients with partial or primary generalized epilepsy, a mechanism not yet demonstrated in non-epileptic patients [3]. In another study from Taiwan, four patients had seizures preceding the thrombotic stroke onset from a few hours to 2–3 days [36]. In the Oxfordshire study 2% of patients had a seizure in the year before the stroke, a threefold increase compared to the general population [8]. Another case-control study of 230 patients showed an eightfold increased risk of epilepsy before stroke [66]. A more recent, population-based study from the UK matched 4709 patients who had seizures beginning at or after the age of 60 with controls and followed them for 5–7 years. In the seizures group, 10% of patients developed strokes and in the control only 4.4%. In a Cox model, the estimated relative hazard of stroke at any point for patients with seizures compared with the control group was 2.89 [67]. In addition, the previously cited large prospective study from Switzerland reported 6/3628 (0.16%) patients with seizures within the week preceding the stroke onset [57]. Three patients had infarcts and three ICH. The authors suggest that these pre-stroke seizures are caused by an initially pre-clinical lesion, which because of rebleeding, developing edema or extension or dysfunction of adjacent or remote structures (diaschisis) evolves into a clinical stroke syndrome. Because up to 11% of patients with first clinical strokes have asymptomatic cerebral infarctions on computed tomography (half of which involve the cortex) [68], it is conceivable that the preceding seizures arise from these asymptomatic lesions [36]. Two studies support this theory: in the first study, 15/132 (11.4%) patients with late age onset seizures and no history of stroke had infarcts on CT of the head versus two of age- and sex-matched controls (p = 0.003). However, 60% of these infarcts were lacunes [69]. In another study, 75/387 (19%) of patients older than 50 years with new-onset seizures had ischemic lesions on CT of the head, making cerebral vascular disease the most frequently identified cause of late-in-life-onset epilepsy [34].
Ischemic Changes in Time and Development of Seizures
The interplay between the depth and duration of ischemia and the development of epileptic epiphenomena is still unknown, but new developments have shed a light. Post-stroke seizures are associated with more severe brain ischemia as shown by positron emission tomography [70]. Seizures associated with migraine may be warning signs of an underlying cerebral infarction [71]. A causal relationship between TIAs and seizures has been difficult to establish. Repetitive involuntary movements in association with TIAs have been reported. EEG in these patients did not show epileptiform activity and the movements did not respond to phenytoin [3, 72].
Onset seizures after acute stroke have common features with acute seizures after traumatic brain injury and imply a common pathogenesis [7, 8]. In animal models, however, there may be differences. In rats, for example, non-convulsive seizures occur more acutely and intensely after permanent middle cerebral artery occlusion than penetrating ballistic-like brain injury [73].
Since there may be a free time interval between the development of late seizures after early post-stroke seizures, the pathophysiologic mechanisms may differ between early and late seizures or be evolving in time [12]. Early seizures are thought to emanate from electrically irritable tissue in the penumbra of the lesion [74, 75], due to regional metabolic dysfunction and excitotoxic neurotransmitter release, such as glutamate [76]. Dysfunction of inhibitory GABAergic circuits is another possibility. Accumulation of Ca++ and Na+ inside the cell results in depolarization of the cellular membrane and the activation of several intracellular cascades. This has been shown in animal models of stroke [77], where cortical neurons in the neocortex and hippocampus had altered membrane potentials and increased excitability [78, 79], as well as in vitro models of hippocampal neuron cultures, where a single 30 min 5 mcM glutamate exposure produced dead cells or cells manifesting recurrent epileptiform discharges and with increased intracellular calcium levels [76].
In a rat model, Nedergaard and Hansen showed that the penumbral depolarization after MCA occlusion is either spreading depression waves (from K+ or glutamate release from the core of the infarct) or ischemic depolarizations (due to blood flow fluctuations around the threshold of anoxic membrane failure) [80]. These derangements do not remain stationary, but constitute an evolving process. In a rat model of forebrain ischemia, there was a differential seizure threshold to infused pro-epileptic agents, that was changing over time [81]. Thus, it is suggested that after an initial critical period, estimated around 3 h, the progression of the penumbra tissue towards necrosis leads to lessened epileptogenic activity , which accounts for the decrease in seizure frequency between 3 and 24 h after stroke [57]. These findings are supported by another neurophysiological study using transcranial magnetic stimulation, where six out 84 patients with stroke showed a decrease in duration of the silent period in either the arm or leg of the affected side compared to the unaffected limb [82]. Five of these six patients had early or late post-stroke focal seizures and no interictal epileptiform activity on the EEG. The authors suggested that this finding was due to decreased cortical inhibitory activity related to functional or structural impairment of GABAergic interneurons.
The potential role that hypoxemia may play, in addition to the presence of a penumbra is exemplified in a large retrospective study from Belgium. Two hundred thirty seven patients with post-stroke seizures were compared with 939 stroke patients without seizures. The interesting finding from this study was that the only independent factors associated with post-stroke seizures were partial anterior circulation syndrome/infarct and presence of chronic obstructive pulmonary disease (COPD) . The authors suggested that nocturnal desaturations in patients with COPD playing an additional role to stroke [83].
How much do seizures affect the evolution of the triggering stroke? The effect of seizures on the infarcted area, through hypoxia, lactic acidosis, ionic changes, and a higher metabolic demand of the brain, is thought to lead to persistent worsening of the neurologic deficits [18, 84]. In one particular study, persistent partial motor post-stroke seizures led to persistent worsening of the prior neurologic deficit in 10/48 patients, without new CT or MRI findings [84]. In a rat model of permanent occlusion of the middle cerebral artery, levetiracetam was given immediately before the surgery in one group and its vehicle to the controls. Both in drug-treated and in control rats, EEG activity was suppressed after the MCA occlusion. In control but not in drug-treated rats, EEG activity reappeared approximately 30–45 min after the occlusion and initially consisted in single spikes and, then, evolved into spike-and-wave and polyspike-and-wave discharges. In rats sacrificed 24 or 72 h after the occlusion, the ischemic lesion was approximately 50% smaller in drug-treated than in control rats, implying a neuroprotective effect of levetiracetam potentially through seizure-like phenomena suppression [85]. However, several other studies have failed to demonstrate worsening of prognosis after post-stroke seizures [9, 11, 19, 56].
Late seizures probably are due to gliosis and the development of meningocerebral cicatrix, leading to persistent hypoperfusion and anoxia, dendritic deformation and hypersensitivity or denervation supersensitivity [3, 35, 75].
Another interesting recent finding was the association of post-stroke seizures with subsequent development of dementia. In the Lille, France, large prospective stroke/dementia cohort, cognitive function before and after a stroke was evaluated longitudinally with a battery of tests. Early post-stroke seizures (within 1 week from stroke onset) were independently predicting new-onset dementia within 3 years (hazard ratio 3.81, 95% CI 1.13–12.8). The authors suggested that seizures were a marker of an underlying condition associated with an increased risk for dementia, such as more severe vascular pathology, an underlying pre-clinical degenerative disease such as Alzheimer’s or post-stroke complications [86].
Localization and Etiology of Ischemic Stroke and Seizures
The importance of cerebral cortical involvement and specific cortical areas in the generation of post-stroke seizures is exemplified in a large prospective study of 661 patients admitted to a Stroke Unit. In this study, 328 patients had MRI-confirmed cerebral infarcts (with cortical involvement in 178 of them). Early onset seizures (defined as seizures within 14 days) were all initially partial seizures and occurred in 4.3% and at stroke onset in 1.5% of patients. These seizures occurred exclusively in patients with cortical involvement. With infarcts involving the cerebral cortex, there was a higher risk of early seizures in watershed infarctions than in territorial strokes (23.1% vs 5.3%). Logistic regression analysis showed an almost four fold increased risk of early seizures in patients with watershed infarctions compared with other cortical infarcts (odds ratio, 95% CI, 4.7, 1.5–15.4). Interestingly in this study, age, sex, diabetes mellitus, hypertension, smoking, NIHSS score, and cardioembolic origin were not significant predictors for early seizures [87]. Cortical distribution of the ischemic infarction was also independently associated with seizures (odds ratio, 95% CI 5.5, 1.53–20.2) in a retrospective study from Taiwan with a long follow-up period (minimum 6 years) [51]. In a prospective study from Perugia, Italy, cortical involvement, severe and large stroke size and cortical hemorrhagic transformation were predictors for early post-stroke seizures in the univariate analysis, but only hemorrhagic transformation was an independent predictor in the multivariate [48].
In addition, neurological complications within the first week of an acute stroke may influence the risk of early seizures. In the Brescia Stroke Registry in Italy, 3.9% of 516 patients with acute stroke developed early seizures. Patients with early seizures had a higher burden of complications compared with those without (30 vs. 4.2%, for patients with >6 complications). The odds ratio and 95% CI were 1.57, 1.21–2.01 for any increase of 1 in the number of complications [88].
Although the involvement of the cerebral cortex after ischemic stroke is thought to be necessary for the occurrence of seizures, there have been several reports of seizures associated with lesions involving subcortical structures. In mice exposed to permanent occlusion of the right common carotid artery and then systemic hypoxia, generalized motor seizures were observed within 72 h. These seizures occurred nearly exclusively in animals with extensive brain injury in the hemisphere ipsilateral to the carotid occlusion, but their generation was not associated with electroencephalographic discharges in bilateral hippocampal and neocortical recordings, suggesting origin from deep subcortical structures [89]. In humans, lacunar infarcts have been implicated in the development of seizures, either through more widespread cerebrovascular disease and involvement of adjacent cortex not apparent on the CT of the head or because subcortical lesions, such as those in the caudate head, may induce seizures [5, 29]. Four out of 13 patients with seizures after acute stroke had subcortical lesions in a prospective study from Birmingham, UK [11]. Only 3 out of 273 (1%) patients with lacunar infarcts had seizures in the previously quoted large prospective study from Dijon, France [5]. In the same population, the authors reported in a different study that 11/13 (85%) patients with seizures and CT-proven lenticulostriate strokes had an associated ipsilateral posterofrontal or anterotemporal cortical ischemic lesion demonstrated by MRI. On SPECT, 13/13 (100%) patients had decreased CBF in the ipsilateral frontal area. Also, in patients with a lenticulostriate stroke, a larger size of both the subcortical and ipsilateral cortical ischemic lesions were predictive of seizures [90]. Five patients (3%) in the Oxfordshire study with lacunes had seizures [8] and another five patients with lacunes and grand mal seizures have been reported in a case series from Israel [91]. In the multicenter, prospective SASS study, 2.6% of patients with lacunes developed seizures, but 7/8 had also other identifiable reasons [7]. In a prospective hospital-based registry, 113 patients with non-lacunar subcortical infarcts were studied. Seizures occurred in 4 (3.5%) of these patients, all with striatocapsular infarcts: two within the first 24 h, one within the first month and one within the first year. Cardioembolic strokes were more common in patients with seizures [92]. In the Hong Kong study, 1.5% of patients with lacunar strokes developed seizures, contrasting with the 8.8% of those with total anterior circulation infarcts and 7% of those with partial anterior circulation infarcts [42]. In the recent Turkish study, epilepsy developed in 1.3% of patients with lacunar strokes [45]. Other large studies have not found an association between lacunes and seizures [9].
Other subcortical or white matter changes have been implicated in post-stroke seizures. Ten percent of patients with CADASIL have seizures, usually related to ischemic stroke development [93]. In a case report, a patient with new-onset focal epilepsy showed on MRI FLAIR sequence white matter hyperintensities on both hemispheres with confluent lesions at the right parieto-occipital junction, with juxtacortical components. The authors suggested that these white matter lesions closer to the cortex may be the cause of seizures, like in multiple sclerosis [94].
Seizures associated with embolic infarcts may be due to commonly seen hemorrhagic conversion, since iron deposition in rat brain tissue is known to be epileptogenic [95]. The same way, iron deposition may play a significant role in seizure development after ICH, although this mechanism does not adequately explain the early onset frequently seen. In addition, thrombin is thought to play a significant role in seizures after ICH [96].
EEG Findings
In the ICU, EEG allows evaluation of post-stroke neurological symptoms [75], such as focal weakness (Todd’s paralysis ) or coma (due to NCSE) [97], which may be due to seizures rather than be permanent deficits from stroke and may be treatable. Normal EEG after post-stroke seizures has been reported in 4–15% of cases [9, 12, 16, 35]. As with ICH [50], the use of CEEG monitoring in the ICU has revolutionized the detection of seizures post-ischemic stroke, many of which are subclinical. [50]. In a study from Switzerland, which evaluated 100 patients admitted to a stroke unit with acute stroke (ischemic in 91 and hemorrhagic in nine patients), CEEG was performed for a mean duration of 17 h 34 min. Epileptic activity occurred in 17 patients and consisted of repetitive focal sharp waves in seven, repetitive focal spikes in seven, and PLEDs in three. Although clinical seizures occurred in three patients before CEEG (only one had repetitive focal spikes on CEEG), electrical seizures were only recorded in two patients without clinical seizures. On multivariate analysis, stroke severity (higher NIHSS) was the only independent predictor [98].
Abnormalities on the EEG may have a differential predictive value regarding the development of seizures after cerebral infarction. Holmes found that 98% of post-stroke patients with sharp waves, spikes and PLEDs on the EEG developed seizures, but did not correlate these findings with seizure recurrence [13]. Twenty six percent of patients who developed post-stroke seizures had PLEDs in this study compared to only 2% of those who never had one. The prognostic significance of post-stroke PLEDs has also been emphasized in another retrospective study, which noted that all four patients with PLEDs on the initial EEG had recurrent seizures [35]. Conversely, in patients with post-stroke seizures, focal irritative abnormalities (electrographic seizures, epileptiform abnormalities, PLEDs) can be found on the average in one fourth of cases and focal slowing in two thirds [12, 16, 35]. Epileptiform abnormalities on EEG performed within the first 24 h (“unless the patient was critically ill”) were found in 14% of patients with peri-stroke seizures in a large recent prospective study [57]. In another study of 232 patients with stroke (177 with ischemic stroke) with EEG performed within 24 h after stroke onset, sporadic epileptiform focal abnormalities were found in 10% and PLEDs in 6%. SE was recorded in 71.4% of patients with PLEDs. At the multivariate analysis, only early epileptic manifestations were independently associated with PLEDs. These specific patterns, such as PLEDs, that are closely related to early seizures, could be detected with EEG monitoring only [99]. The specificity of the test is poor, since similar findings are not uncommon in patients with stroke without seizures [12]. The frequency of irritative abnormalities can be influenced by the timing and the repetition rate of the test. This may explain why in a prospective study from Denmark, epileptiform abnormalities were reported in only 2/77 (2.6%) patients with supratentorial strokes [26]. EEGs were obtained in all patients within the 1st week after the stroke and repeated at 3 and 6 months. All seven patients who developed epilepsy in this series had focal delta and theta activity on the EEG . One patient had epileptiform activity at 3 months post-stroke, before he developed epilepsy and another on the first EEG recorded 6 days after stroke and 5 days after the first seizure. The authors conclude that EEG was not helpful in determining the risk for developing epilepsy .
Figure 9.1 shows a patient who was in status epilepticus after an old ischemic stroke.




Fig. 9.1
81-year-old AA woman with history of old stroke and left side residual hemiplegi a was admitted to the Neuro-ICU for altered mental status. Continuous EEG exhibited 162 electrographic seizures during the first day of recording, emanating from the right hemisphere. She was treated with IV valproic acid and IV phenytoin. Two days later the EEG showed only interictal activity and after an additional 2 days only mild to moderate encephalopathy, while her mental status was improving. CT of the head showing an encephalomalacic area on the right fronto-parietal area from the old ischemic stroke (white arrow). Continuous EEG showing the beginning (B.1), evolution (B.2), and end (B.3) of a single electrographic seizure (without clinical correlate other than diminished mental status). The rhythmic, high amplitude theta/delta activity starts over P4, P8, and O2, but later spreads to the whole right hemisphere
Figure 9.2 shows another patient who developed a stroke, followed by seizures while driving.




Fig. 9.2
An 84 AA diabetic woman was admitted to the Neuro-ICU after a motor vehicle accident (she was the driver) without head trauma or loss of consciousness. During her transfer she was found hypoglycemic and developed generalized tonic-clonic seizures. An MRI showed a right occipital stroke and an EEG several seizures emanating from the right temporal-occipital areas. She was loaded with phenytoin and levetiracetam IV and the seizures stopped. Diffusion-weighted imaging of the MRI showing the R inferior temporo -occipital stroke (white arrow). EEG showing the beginning from T4 to T6 of a seizure (B.1). This seizure evolved over the entire right hemisphere (B.2)
Neuroimaging
Diffusion-weighted MRI (DWI) and apparent diffusion coefficient (ADC) changes have been well described during acute ischemic stroke. In the acute phase, because of energy substrate depletion and Na+/K+—ATPase pump failure, ionic changes lead to cytotoxic edema and decreased diffusion of water. On DWI ischemic brain appears brighter (high signal) than normal brain, while it is darker on ADC maps (low ADC = decreased signal). Later, when the cells die and cellular membranes are disrupted these changes reverse (decreased DWI signal and increased ADC signal).
However, neuroimaging changes have also been reported after repetitive seizures or SE or NCSE [100], making interpretation more difficult when these conditions co-exist. Senn et al. reported a patient with partial status that had high DWI and ADC signal in the affected areas at the onset of seizures. Seven days later, DWI signal had regressed and ADC had further increased and 10 days later there was marked regression of all signals and signs of focal atrophy [101]. These findings contrast with those of Lansberg et al., who reported three patients with complex partial status epilepticus and decrease in ADC on the first day [102]. These differences may be due to timing of the MRI during SE. Low density changes on CT and high signals on T2-weighted MRI (that do not respect vascular territories), leptomeningeal contrast enhancement (indicative of alteration of the blood-brain barrier and vasogenic edema), local hyperperfusion on MRA and cerebral activation on functional MRI, all reversible, have been well associated with partial epilepsy and could help differentiate between ischemic stroke and seizure activity [101, 102]. Therefore, combining DWI and perfusion sequences may give more interpretable results than ADC and help distinguish the effect of seizures from that of oligemia or ischemia due to stroke. MRI shows hyperperfusion in seizing brain using gadolinium based or arterial spin labeling (ASL) sequences [103, 104]. DWI will display restricted diffusion despite increased perfusion during seizures [105]. In two patients who had partial status epilepticus associated with an old infarction and two episodes each of left hemiparesis and hemisensory disturbance without convulsion, DWI showed a hyperintense lesion in the cortex around the old infarction lesion mimicking current infarction. Although EEG failed to reveal ictal discharges or interictal paroxysmal activities in three of four episodes, perfusion images with ASL clearly demonstrated ictal hyperperfusion in the area corresponding to the cortical hyperintense lesion on DWI. The clinical deficits disappeared after appropriate anti-epileptic treatment [106]. Recent evidence suggests that DWI restriction adjacent to ongoing seizure activity may result from local cortical metabolic disturbances and more widespread cortico-cortical and cortico-thalamic synchronization abnormalities [107].
The long-term significance of these acute imaging findings is not entirely clear. DWI changes are largely reversible, but if prolonged seizures are present, may be associated with laminar necrosis and irreversible deficits [108]. One study of 26 patients with acutely seizures related MRI changes reported residual gliosis or focal atrophy in 42% of their cohort, while the remainder had reversible findings. Partial simple and complex seizures were associated with hippocampal involvement , but status epilepticus with incomplete reversibility of MRI abnormalities [109].
Seizures Post Stroke in the Young and the Elderly
Non-hemorrhagic stroke in young patients (before 45) accounts for 3–10% of all infarctions and has low mortality (1.5–8%) [110–112]. Only few studies have examined the incidence of post-stroke seizures in this younger population.
In a retrospective analysis of 65 young patients (aged 15–45) with stroke, followed for an average 32 months (range 12–59), the incidence of seizures was 10.8% and all occurred in patients with carotid artery territory infarction [113].
In a prospective, multicenter, European study (the PFO-ASA study), 581 patients with cryptogenic ischemic stroke, aged 18–55 years, were followed for seizures for an average of 38 months [22]. None of the six deaths was related to the occurrence of a seizure. Early seizures (within 1 week post-stroke) occurred in 14/581 (2.4%) of patients, 71% of them within the first 24 h. Two patients developed SE, one as an inaugural event. Rankin scale ≥ 3 (odds ratio, 95% CI, 3.9, 1.2–12.7) and cortical involvement (7.7, 1–61.1) were the only clinical and radiologic independent variables associated with early seizures. Late seizures occurred in 20/581 (3.4%) with a mean delay of 12.9 months post-stroke . Six of these patients had early seizures and 4/6 were on anti-epileptic treatment when the first late seizure occurred. Early seizures (5.1, 1.8–14.8), cortical signs (4.5, 1.6–13.1), and size of infarct larger than one-half hemisphere (9.7, 3.1–30.8) were independent predictors for late seizure development. Recurrent, unprovoked late seizures were found in 11/20 (55%) patients with late seizures and the risk for epilepsy was 2.3% within 3 years in this patient population.
In the large Canadian Stroke Network registry, seizures on stroke presentation or during hospitalization were more frequent in younger patients compared to patients with stroke without seizures. In addition to more severe strokes, older age, non-alert status on admission and higher Carlson index, seizures during hospitalization independently predicted overall mortality [21].
The incidence of seizures in the elderly is close to that in the first decade of life. With increasing age, more patients have an identifiable cause for their seizures and thus more seizures may have a focal onset [114]. Luhdorf et al. followed all patients within a definite area who developed seizures after the age of 60. The dominant cause of seizures was previous stroke in 32% of cases [32]. In a retrospective population-based study from Saskatchewan, Canada, 46/84 (55%) of patients 60 years and older with new-onset seizures had acute or remote cause identified (symptomatic). Of those, 22/46 (48% or 26% of all patients with new seizures) were due to acute or old stroke. All patients with non-life-threatening strokes had excellent prognosis, with seizures resolving in all but one patient. No information is provided about the number of elderly patients admitted to an ICU in these studies [115].
Similar findings were noted in a large , prospective, epidemiological study conducted in Southwest France [15]. The annual incidence of seizures in patients older than 60 years was 127/100,000. Cerebrovascular disease was the most frequently recognized cause in this age group and represented 36.6% of patients with spontaneous seizures and 54% of patients with confirmed epilepsy.
More recently, a prospective study from Lille, France, evaluated 202 patients with acute stroke (median age 76 years) for the presence of pre-existing dementia. Early seizures developed in 5.4% of patients in this cohort. Stroke patients with pre-existing dementia had an increased risk of late seizures, but not for early seizures. Any factor increasing the risk of seizures (drugs, metabolic changes), the authors concluded, should be avoided in these patients [116].
Treatment of Post-Ischemic Stroke Seizures
Seizures at the onset of an ischemic stroke were considered a contraindication for IV t-PA [117] and, based on prospective data, they were the reason for not administering IV t-PA in 0.9% of candidates [118]. Conversely, if stroke is treated, but eventually is not proven (usually by DWI on MRI), the initial stroke-like signs are attributed to stroke mimics. In the past, there was stricter adherence to this seizure exclusion criterion for IV t-PA, but more recent data undermined this exclusion.
There are more data now regarding the incidence, treatment, and complications of stroke mimics, including seizures. For example, stroke mimics were diagnosed in 13.4% of patients presenting to the Emergency department. Seizures were the second most common stroke mimic (19.5%). None of these patients received t-PA, mostly because of being outside the time window or because of minor deficits in the NIHSS before starting the infusion [119]. DWI MRI sequence in the setting of a new focal neurological deficit can be used to differentiate between these two conditions and was able to identify stroke mimics from ischemic stroke in another study, where seizures comprised 11.5% of former group [120]. Similarly, using MRI to exclude stroke in patients who received t-PA, seizures were diagnosed in 3.1% of 648 suspected ischemic stroke patients and comprised 47.6% of all stroke mimics. None of the stroke mimic patients deteriorated clinically post t-PA, but one developed orolingual angioedema [121]. The safety of administering fibrinolysis in patients who potentially may not have a stroke, but need to be treated emergently due to the narrow window, has been evaluated in few studies. In a retrospective study of 250 patients who received IV t-PA, 2.8% had stroke mimics (of which 71% were seizures, mostly with focal onset). There was a trend for lower NIHSS scores in mimics. Global aphasia without hemiparesis was the presenting symptom in 3 (42.9%) mimics versus 8 (3.3%) strokes. Orolingual angioedema (1.2%), symptomatic intracranial hemorrhage (5.3%), and asymptomatic intracranial hemorrhage (12.3%) complicated strokes, but were absent in mimics. After 3 months, 85.7% of mimics and 35.4% strokes had a modified Rankin Scale score of 0–1 [122]. In another large retrospective analysis of 539 patients treated with IV t-PA, 10.4% had stroke mimics. Seizures (19.6%) were one of the three most common final diagnoses at discharge for these misdiagnosed patients. No symptomatic intracranial hemorrhage was documented in any of these stroke mimics, suggesting a safe profile for administering IV t-PA in these patients [123]. More recently, in a single center study of patients who received IV t-PA for presumed stroke , 12% had stroke mimics (31.6% were seizures). None of these patients had an intracerebral hemorrhage after the fibrinolysis, but two have systemic hemorrhages [124]. Reflecting these changes in the approach towards stroke mimics and seizures at onset, is a survey of stroke clinicians from eight NIH Specialized Programs of Translational Research in Acute Stroke (SPOTRIAS) . Ninety-one percent of responders recommended fibrinolytic therapy in patients with significant deficits attributable to an acute ischemic stroke but with also a seizure at symptom onset [125].
Interestingly, seizures occurring during the period of thrombolysis with IV t-PA may represent a sign of reperfusion or hyperperfusion and may enhance the thrombolytic process [126–128]. Although in these small case series these seizures were associated with a dramatic recovery, they may also herald hemorrhagic conversion and an urgent CT of the head should be considered if clinically the patient deteriorates. In a more recent case report, cortical myoclonus during the IV thrombolysis for a right middle cerebral artery stroke was recorded using back-average EEG analysis with time-locked electromyogram of the affected contralateral limb. There was no improvement in the deficit. Interestingly this patient had additional acute renal failure [129]. An alternative mechanism of seizures during IV fibrinolysis is a direct effect of the drug on seizure generation. For example, t-PA is an endogenous serine protease associated with neuronal activity and synaptic plasticity in the brain, its expression is enhanced after seizures, and is involved in seizure propagation throughout the brain. Although mice with neuronal over-expression of t-PA are more seizure-prone than wild-type, no significant differences were found in a study that compared patients who received t-PA and those who did not in early or late seizures or in the development of epilepsy [130].
The most recent guidelines from the Stroke Council of the American Stroke Association include seizures as a relative exclusion criterion for administration of fibrinolytics. After careful consideration of risk-benefit patients may receive fibrinolysis in this situation [131]. CT angiography may be a useful modality in differentiating Todd’s paralysis from early seizure and ischemia by detection of intracranial occlusion and may contribute to decision-making for thrombolysis [132].
The same recent guidelines from the Stroke Council of the American Stroke Association mention that there are no studies to date showing a benefit of prophylactic anti-epileptic treatment after ischemic stroke and prophylactic treatment is not recommended (Class III, Level of Evidence C). Their recommendation is to treat recurrent seizures in a manner similar to other acute neurological conditions. The anti-epileptic drugs to be used should be selected by specific patient characteristics (Class I, Level of Evidence B). They also mention that little information exists on indications for the long-term use of anticonvulsant drugs after a seizure [131]. Reiterating these Guidelines, the Neurocritical Care Society Pharmacy Section issued a review of evidence-based support for seizure prophylaxis in Neurocritical Care. In this most recent special article, no additional data or recommendations beyond the aforementioned from the ASA are included for post-stroke seizures [133]. In the following paragraphs we will try to summarize the data available and have an in-depth look at the problem an intensivist faces.
As mentioned before, there are no randomized controlled trials evaluating specific treatment options in post-stroke seizures . Bladin et al. argue that such a trial would pose extensive logistic challenges and would likely be unethical [7]. Prophylactic treatment for seizures after ischemic stroke is controversial. The duration of treatment is also unknown. Should these patients be admitted to an ICU when they present with a seizure in the context of an acute or subacute stroke? Armon et al. have summarized the rationales for treating seizures at the onset of a stroke, as follows: (a) control of persistent or recurrent seizures (SE) (b) prevention of seizures within the first day of stroke (c) prevention within the first 2 weeks post stroke, and (d) prevention of recurrence of seizures after the first 2 weeks or the appearance of late seizures [3]. We suggest adapting the same rationale to the ICU setting by tailoring it to the following issues: (a) whether to treat or not a seizure that accompanies a stroke (b) whether to treat recurrent seizures or SE (c) whether to treat preventively post-stroke seizures (d) whether to prevent late seizures from occurring after an early seizure (e) whether to prevent epilepsy from developing after early or late seizures.
The current consensus in ischemic stroke management is to not treat patients unless they present with a first seizure or they are known as pre-existing epileptics [134]. If a patient had pre-stroke seizures, the rationale for covering with anti-epileptics is based on the prevention of pre-existing epilepsy to manifest in its usual form post stroke or as SE [3]. However, even before the occurrence of the first seizure, some subgroups of non-epileptic patients may be at higher risk for seizure development. Large infarct size, cortical involvement, watershed distribution, hemorrhagic stroke transformation, severe stroke and PLEDs on the EEG within 24 h from admission [5–7, 16, 19, 22, 35, 39, 41, 48, 53, 54, 56, 87, 99, 135] are typical situations where a physician could consider covering the patient with anti-epileptic treatment to prevent early seizures, especially if other co-morbidities make a seizure manifestation an unacceptably high risk for complications .
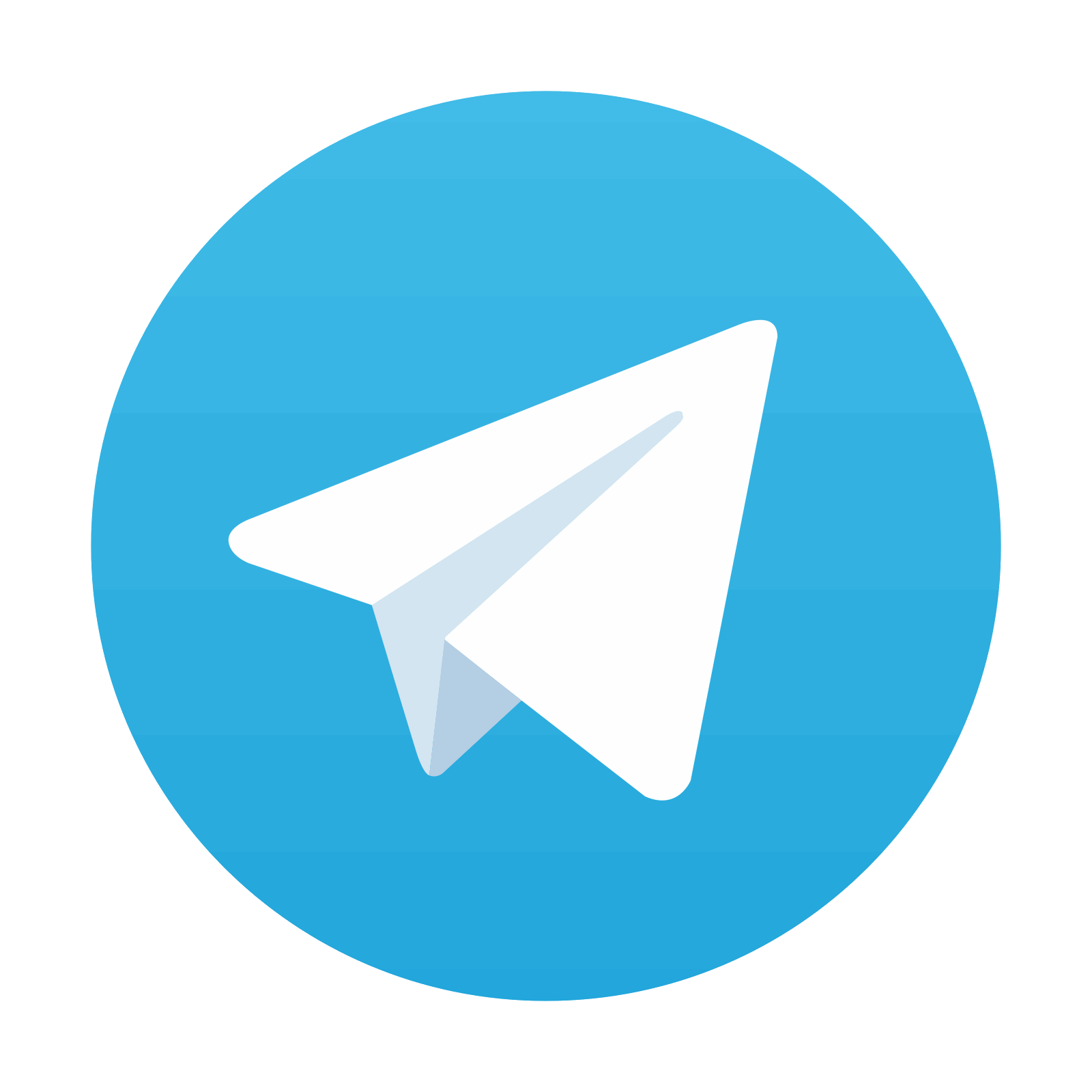
Stay updated, free articles. Join our Telegram channel

Full access? Get Clinical Tree
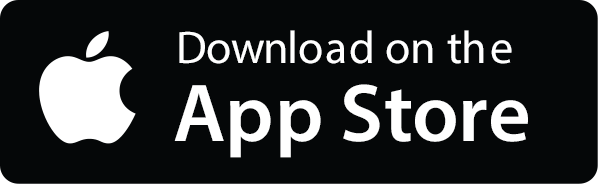
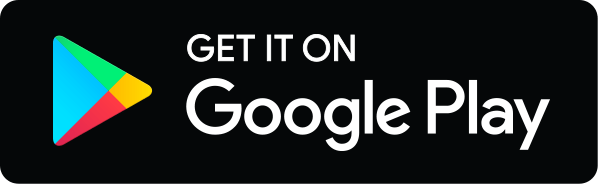