Stage
Physical characteristics
1
Prepubertal
2
Breast budding, elevation of both breast and nipple as a small mound
3
Continued enlargement of both breast and areola without separation of their contours
4
The areola and nipple form a secondary mound projecting above the contour of the breast
5
Adult shape, areola and nipple recessed to the contour of the breast
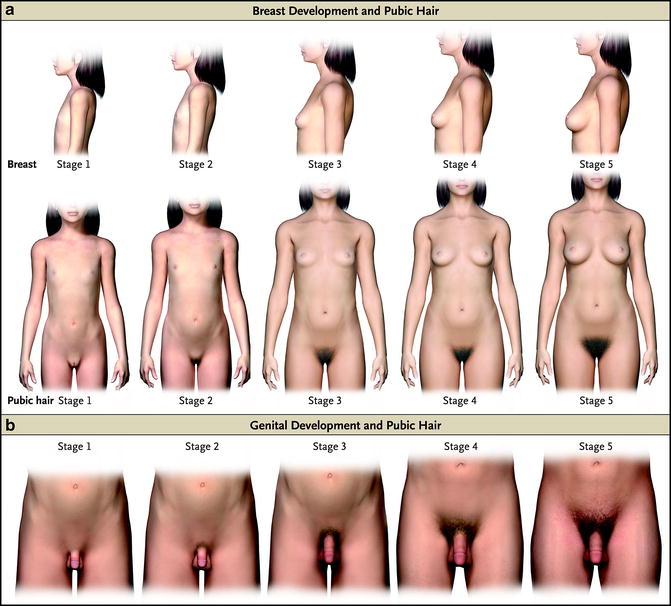
Fig. 1
Stages of sexual maturation according to Tanner. The top two rows illustrate stages of breast and pubic hair maturation in girls. The bottom row illustrates stages of genital and pubic hair maturation in boys. See Tables 1, 2, and 4 for additional details. Used with permission from Carel JC, Leger J. (2008). Precocious puberty. N Engl J Med, 358:2366–77. Copyright Massachusetts Medical Society
Table 2
Pubic hair maturation in males and females
Stage | Physical characteristics |
---|---|
1 | Prepubertal |
2 | Sparse growth of long, lightly pigmented hairs at the base of the penis in males or the mons veneris/labia majora in females |
3 | Additional darkening and coarsening of hair, spreading over the pubic symphysis |
4 | Adult in character but has not spread to the lower abdomen in males or to the medial surface of the thighs in males and females |
5 | Adult in distribution, extension to the lower abdomen in males and/or the medial surface of the thighs in males and females |
Marshall and Tanner (1969, 1970) have noted the mean ages and variability of reaching the individual stages of sexual maturation (Table 3) for girls and boys. They have also shown the mean duration to pass through some significant maturational stages (Table 3) and the mean age for menarche. Although present data may indicate an earlier beginning of the stages of pubertal maturation and menarche (Rosenfield, Lipton, & Drum, 2009), the process remains similar in terms of time between these various stages. The exception occurs in the very youngest of girls (Rosenfield et al., 2009), in whom the time from breast Tanner stage 2 to menarche is prolonged, so that although starting breast maturation considerably early, menarche is but several months earlier than previous data indicate.
Boys | Girls | ||||
---|---|---|---|---|---|
Mean | 95% CI | Mean | 95% CI | ||
G 2 | 10.1a | 9.6–10.6 | B 2 | 10.4 | 10.1–10.7 |
G 3 | 12.4 | 12.0–12.7 | B 3 | 11.8 | 11.5–12.0 |
G 4 | 13.5 | 13.2–13.8 | B 4 | 13.3 | 13.0–15.9 |
G 5 | 15.9 | 15.3–16.4 | B 5 | 15.5 | 15.0–15.9 |
PH 2 | 12.0 | 11.7–12.3 | PH 2 | 10.6 | 10.3–10.9 |
PH 3 | 12.6 | 12.3–13.0 | PH 3 | 11.8 | 11.5–12.1 |
PH 4 | 13.5 | 13.2–13.8 | PH 4 | 13.0 | 12.7–13.3 |
PH 5 | 15.7 | 15.3–16.0 | PH 5 | 16.3 | 15.9–16.9 |
G 2–G 5 | 5–6 | B 2–B 5 | 5 | ||
Menarche | 12.55 | 12.31–12.79 |
There is also great variability in the timing of peak height velocity (PHV), which is an excellent biological anchor of pubertal maturation, in terms of stages of breast or genital maturation or in terms of the progression of pubic hair (Figs. 2 and 3). Thus, the mid-pubertal growth spurt may occur at virtually any of the mid-pubertal breast or genital stages or pubic hair stages. Similarly, there is variability in the timing of pubic hair maturation in all of the stages of genital or breast maturation (Fig. 4).
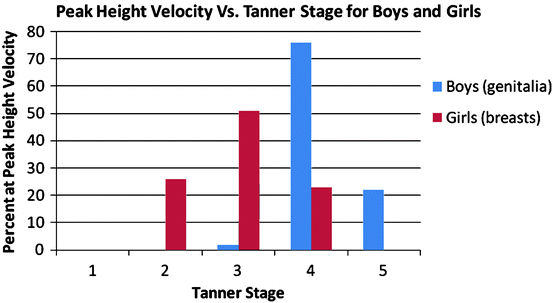
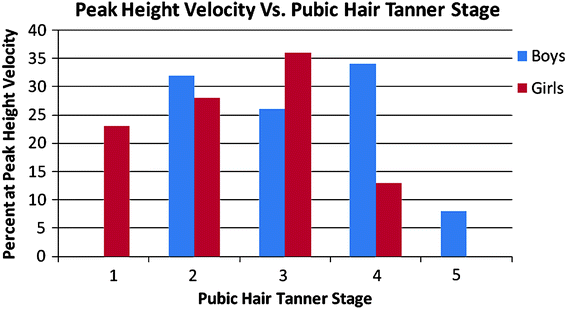
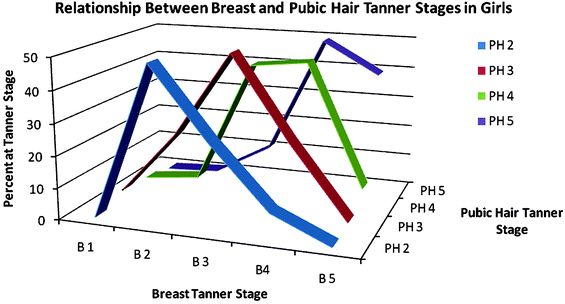
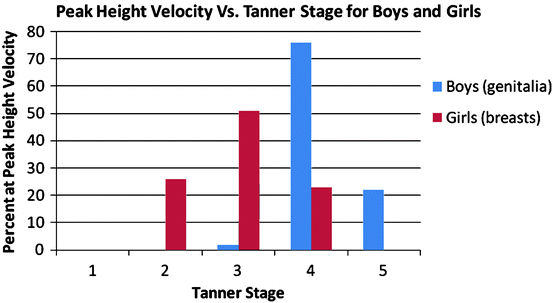
Fig. 2
Relationship between peak height velocity and breast and genital maturation in boys and girls, respectively. In girls, peak height velocity occurs at a median Tanner stage of 3, whereas in boys it occurs later, at a median Tanner stage of 4. Data are from Tanner, Whitehouse, Marubini, and Resele (1976)
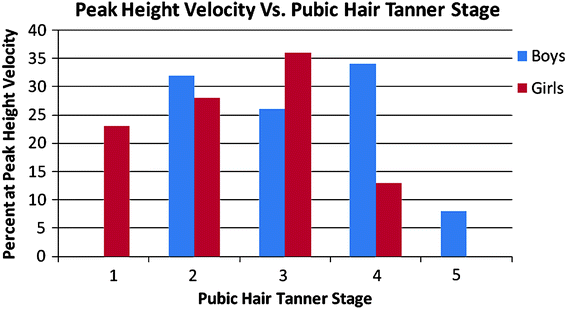
Fig. 3
Relationship between peak height velocity and pubic hair maturation in boys and girls. Unlike breast and genital maturation, there is a wide variation in the degree of pubic hair maturation at the time of peak height velocity, although boys tend to be further advanced than girls. Data are from Tanner et al. (1976)
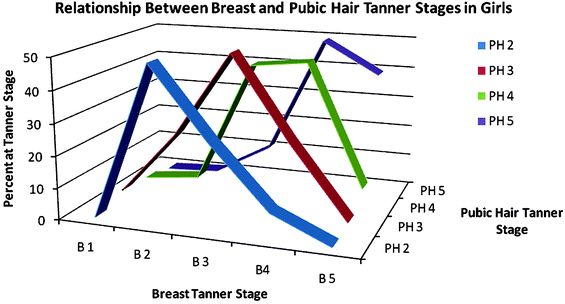
Fig. 4
Relationship between breast and pubic hair maturation in girls. Although there is a general concordance between breast and pubic hair maturation, significant variability exists. Data are from Tanner et al. (1976)
Boys
Similarly, genital maturation in boys is divided into five stages from stage 1 (prepubertal) in which the penis, testes, and scrotum are about the same size and proportions as in early childhood to stage 5 (fully mature, see Table 4 and Fig. 1). In a manner similar to that in girls, pubic hair is divided into stages 1 (none) to 5 (dark, coarse mainly in the triangular distribution but may spread to the medial thighs or vertically). Adolescent boys may attain adult height and mature sexual maturation years before acquiring their adult body composition.
Table 4
Genital maturation in males
Stage | Physical characteristics |
---|---|
1 | Prepubertal |
2 | Enlargement of the testes and scrotum, thinning and reddening of the scrotal skin, penis remains prepubertal |
3 | Further growth of testes and scrotum, enlargement of the penis in length and width |
4 | Further growth of testes and scrotum with pigmentation of the scrotal skin, further enlargement of the penis with maturation of the glans |
5 | Testes, scrotum, and penis are adult in size and shape |
Testicular maturation (size) is conveniently measured by comparison to a Prader orchidometer, which is a set of ellipsoids roughly of the size and shape of testes from prepubertal to fully adult (Fig. 5). Other available methods include rulers (semimajor and semiminor axes), calipers, and ultrasound. The normal prepubertal testis is 1–2 mL and the fully mature one 15–25 mL. One considers puberty to have begun when the testes are >3 mL. There is a rough correlation between testicular size and the Tanner stages for genital and pubic hair maturation. The common practice is to denote each parameter individually.
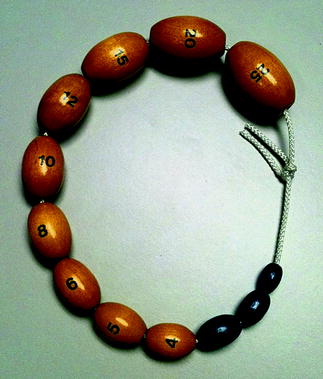
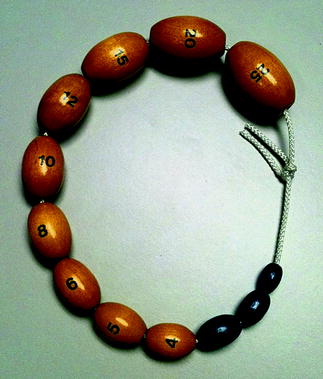
Fig. 5
The Prader orchidometer is a series of calibrated ellipsoid beads ranging from 1 mL to 25 mL in volume. Testicular volumes up to 3 mL (blue beads) are considered prepubertal. Normal adult testicular volume is 15–25 mL
Pubertal Growth Spurt
The pubertal growth spurt can be divided into three stages: the stage of minimal growth velocity just before the spurt (takeoff velocity), the stage of most rapid growth or peak height velocity (PHV), and the stage of decreased velocity and cessation of growth at epiphyseal fusion. Boys reach PHV approximately 2 years later than girls and are taller at takeoff; PHV occurs at stages 3 to 4 of puberty in most boys and is completed by stage 5 in more than 95 % of boys (Largo, Gasser, Prader, Stuetzle, & Huber, 1978; Tanner, Whitehouse, Marubini, & Resele, 1976). The mean takeoff age is 11 years, and the PHV occurs at a mean age of 13.5 years in boys. The total height gain in boys between takeoff and cessation of growth is approximately of 31 cm (Abbassi, 1998). The mean height difference (boys taller than girls) between adult height men and women is 12.5 cm.
Hypothalamic-Pituitary-Gonadal Axis
In physiologically mature individuals, the secretion of gonadal steroids (testosterone in boys and estradiol in girls) is controlled by the gonadotropic hormones, luteinizing hormone (LH), and follicle-stimulating hormone (FSH), secreted by the pituitary gland. LH and FSH secretion are themselves regulated by gonadotropin-releasing hormone (GnRH) which is produced in the hypothalamus. In the adult, GnRH is secreted in tightly regulated bursts (pulses) every 60–120 min throughout the day (Knobil, 1980). This pulsatility is required for normal gonadotropin secretion and gonadal steroid production. In the circulation, estradiol and testosterone travel not only to target organs but also to the CNS, where they act to suppress production of GnRH, LH, and FSH, thus establishing a negative feedback loop.
Maturation of the HGP Axis
In the prepubertal child, the situation differs markedly from the adolescent and adult. During early infancy, the HPG axis is active, but it then becomes suppressed until the approach of puberty. This suppression is known as the juvenile pause. During this stage, GnRH and gonadotropin secretion are highly suppressed by the low concentrations of circulating gonadal steroids (Sisk & Foster, 2004). With currently available assays, the serum concentrations of testosterone and estradiol are usually unmeasurable, but they circulate at levels below those necessary for the physical changes of puberty. However, estradiol levels are sufficient to add to the growing difference in fat mass in boys and girls between the ages of approximately 6 years and the onset of the external signs of pubertal maturation. The heightened negative feedback keeps sex hormone concentrations low and prevents pubertal maturation, although GnRH continues to be produced in small, irregular, and widely spaced pulses (Sisk & Foster, 2004). Gonadotropins are also secreted, again at very low rates that may be difficult to detect in routine clinical laboratory assays.
In late childhood, before any physical evidence of puberty is present, the intensity of the negative feedback diminishes. Although the mechanism for this process is poorly understood, GnRH pulse amplitude and frequency both increase as the sensitivity to sex steroids decreases (Dunkel, Alfthan, Stenman, Tapanainen, & Perheentupa, 1990). The increased GnRH pulsatility first occurs primarily at night, with resulting diurnal variation in gonadotropin secretion and waxing and waning levels of sex hormones over the course of the day (Fig. 6). The increasing circulating sex-steroid levels directly lead to the physical and behavioral alterations noted during pubertal maturation. As puberty progresses, GnRH pulses become more consistent throughout the day, and the troughs and peaks in sex hormone concentrations tend to even out somewhat. However, the diurnal testosterone variation in boys persists into adulthood, although with a smaller day/night difference (Manasco et al., 1995). For this reason, measurement of testosterone in adolescent and young adult males is best performed in the AM, when levels tend to be at their highest. In girls, serum estradiol concentrations increase from <5 pg/mL (∼18 pmol/L) before puberty up to >100 pg/mL (∼370 pmol/L) in a post-menarchal girl, varying greatly over the menstrual cycle. Testosterone concentrations in prepubertal males generally are below 10 ng/dL, (∼0.3 nM) and in young adults they are above 300 ng/dL (∼10 nM).
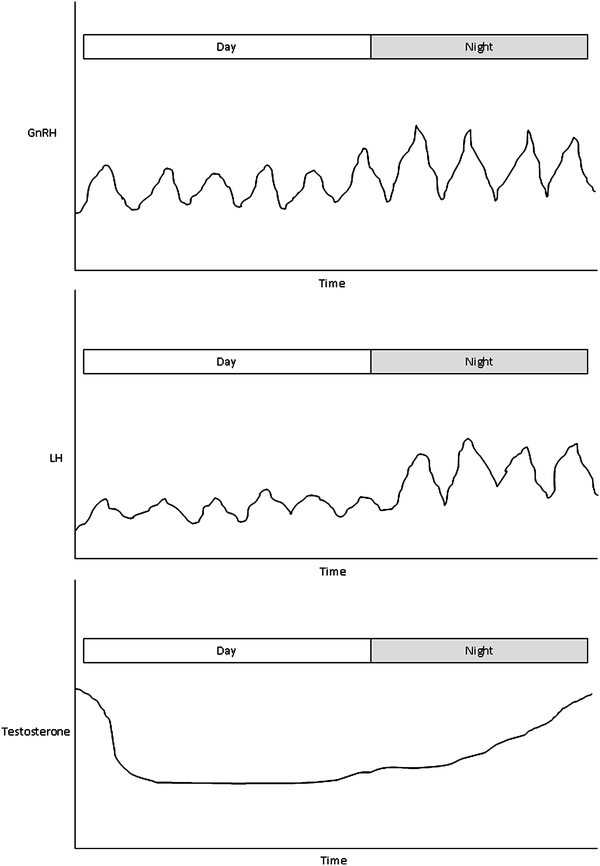
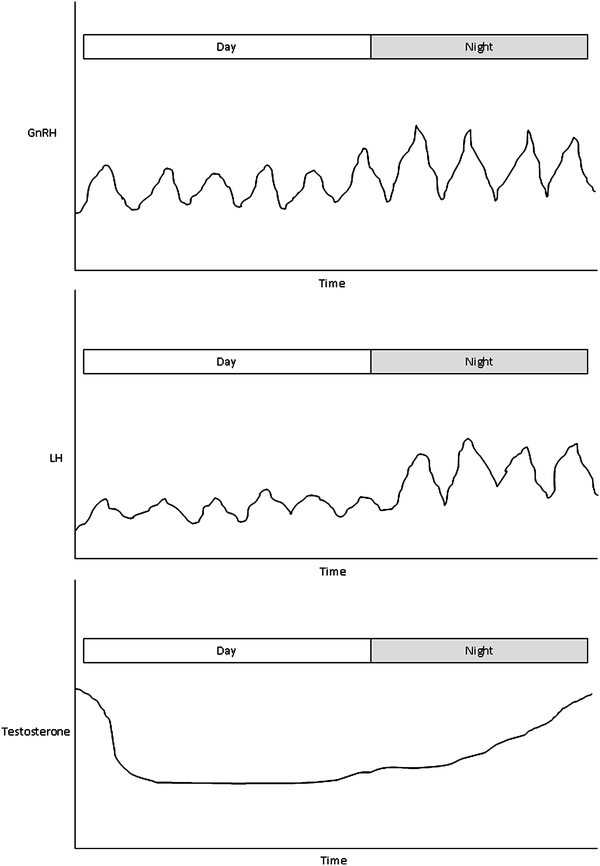
Fig. 6
GnRH, LH, and testosterone secretion in the early to mid-pubertal adolescent boy. GnRH is secreted in a pulsatile manner, with parallel pulses of LH release. The pulse amplitudes of both GnRH and LH increase at night, resulting in a gradual rise in serum testosterone concentrations as the night progresses. Testosterone peaks in the morning, and levels decline during the day
Establishment of the Menstrual Cycle
During the prepubertal years, the ovaries gradually increase in size, and the number of small follicles increases (Bridges, Cooke, Healy, Hindmarsh, & Brook, 1993; Peters, Byskov, & Grinsted, 1978). In response to increased GnRH pulses primarily during the nighttime, gonadotropin secretion increases, particularly FSH. The increased serum concentration of FSH leads to increased follicular recruitment. Acting via its receptor, LH promotes androgen production, primarily androstenedione, from the ovarian thecal cells. FSH stimulates aromatase activity in the granulosa cells and leads to estradiol production. Before menarche, estradiol levels peak approximately 12 h after the diurnal peaks in gonadotropin secretion (Norjavaara, Ankarberg, & Albertsson-Wikland, 1996).
As puberty progresses, a cyclic pattern emerges in estradiol secretion (Rosenfield, Cooke, & Radovick, 2008). In this setting, estradiol comes from the small antral follicles developing midway through pubertal maturation. As estradiol concentrations increase and promote endometrial proliferation, anovulatory bleeding may occur following cyclic declines in estradiol. True ovulatory cycles do not begin until after the HPG axis matures to the point at which the mid-cycle LH surge takes place.
Endocrine Regulation of the Menstrual Cycle
Before ovulation (the follicular phase), gradually increasing FSH and LH production prompt the recruitment of small follicles, which begin to secrete estradiol. With increasing stimulation, the follicle with the greatest FSH sensitivity becomes dominant and begins to produce progesterone as well as additional estradiol. Estradiol causes proliferation of the endometrial lining of the uterus. Although in boys, sex steroids consistently have a suppressive effect on GnRH and gonadotropin secretion, such is not the case for girls. In the mid-part of the menstrual cycle, increasing serum concentrations of estradiol, acting synergistically with progesterone, cause a dramatic increase in LH secretion, with levels approaching 60 IU/L. In the dominant (ovulatory) follicle, alterations in gene transcription in response to the LH surge result in the formation of inflammatory mediators and disruption of cellular integrity, causing rupture of the follicle and ovulation (Russell & Robker, 2007). After ovulation (the luteal phase), estradiol concentrations initially decline, but as the corpus luteum forms, both estradiol and progesterone levels increase, resulting in endometrial maturation. In the absence of embryonal hCG, however, the corpus luteum has a limited lifespan, and hormone production declines. With falling estradiol and progesterone levels, the endometrium becomes ischemic and sloughs off, resulting in menstrual bleeding. The high FSH levels observed during the follicular phase and at the end of the luteal phase cause the initial recruitment of another wave of small follicles, one of which will become the dominant follicle 2½ cycles later (Gougeon, 1996).
Regulation of Pubertal Timing
The timing of puberty is a complex trait, and in the general population, it has a Gaussian distribution. There are many influences on the regulation of pubertal timing, including nutritional factors, environmental influences, and genetic input. Complex traits often demonstrate a high degree of genetic regulation, and it is estimated that between 50 and 80 % of the variance of normal pubertal timing is explained by genetic factors (Palmert & Boepple, 2001). Efforts to understand the genetics of pubertal timing have led to the discovery of many genes that are clearly necessary for pubertal maturation. Many of these, when mutated, cause specific syndromes of delayed or absent puberty. However, it is not clear that these genes individually or as a group explain much of the variability of the onset of normal puberty.
In 2003, the kisspeptin/GPR54 system was discovered. It is a key regulatory system in the initiation of increased GnRH pulsatility at the onset of puberty (Seminara et al., 2003). Kisspeptin is produced by hypothalamic neurons and interacts with its receptor, GPR54, on GnRH-secreting neurons. In humans, inactivating mutations are associated with pubertal delay, and an activating mutation has led to precocious puberty (Teles et al., 2008). Exogenous administration of kisspeptin increases the pulse amplitude of GnRH. Short-term administration of kisspeptin to healthy male and female volunteers increases gonadotropin concentrations, although chronic dosing appears to induce tachyphylaxis (Dhillo et al., 2005, 2007; Jayasena et al., 2009). The kisspeptin/GPR54 system may also be involved in the negative and positive feedback effects of sex steroids on GnRH secretion. Although it is clear that kisspeptin plays a role upstream of GnRH, the factors regulating its release are not presently fully understood (Kauffman, Clifton, & Steiner, 2007).
Both inhibitory and stimulatory factors in the central nervous system are involved in the initiation of puberty. Gamma-aminobutyric acid (GABA)-secreting neurons play an inhibitory role and may be involved in the temporary suppression of the HPG axis during childhood. Suppression of GABAergic neuronal input may lead to the initiation of puberty (Terasawa & Fernandez, 2001). Additional factors may include increased glutamate stimulation of N-methyl-d-aspartate (NMDA) receptors, which are stimulatory to GnRH release, and neuropeptide Y-expressing neuron activity (Gamba & Pralong, 2006). Leptin, acting through its receptor, links nutritional status to puberty, and sufficient levels of leptin are thought to be required to initiate puberty (Farooqi et al., 2002).
Role of Energy Balance in Pubertal Maturation and the Menstrual Cycle
One must have sufficient energy to support body growth and to store energy, predominantly as fat, for longer-term energy requirements such as the menstrual cycle, pregnancy, and lactation (Loucks, 2007). Thus, energy availability is one of the factors that contribute to the regulation of timing of puberty and the menstrual cycle. Energy availability is defined as the difference between energy intake and energy expenditure, and it may be decreased by either lowering food intake or increasing caloric expenditure through exercise. Loss of energy availability leads to alterations in puberty and the menstrual cycle by decreasing the frequency of GnRH pulses, thus lowering mean serum levels of gonadotropins, decreasing ovarian follicle formation, and decreasing estradiol secretion (Gordon, 2010). This phenomenon may be mediated by loss of body fat and decreases in circulating leptin, considered permissive for normal hypothalamic-pituitary-ovarian activity. Younger women and adolescents are particularly prone to hypothalamic amenorrhea as a result of decreased energy availability. Loss of energy availability is reflected clinically by decreases in percent body fat and, to a lesser extent, body weight and BMI. In athletes, weight and BMI may not accurately reflect body fat stores due to the higher density of muscle. Restoration of energy availability results in increased body weight. Return of body weight to >90 % of ideal usually, but not always, results in resumption of menses in amenorrheic patients (Golden et al., 1997). Whether weight loss occurs from excessive exercise or by severe caloric restriction, as seen in starvation and anorexia nervosa, the hypothalamic effects appear the same.
Hypothalamic-Pituitary Axis for Growth Hormone/IGF-1
Growth hormone release is intermittent (“burst-like” or pulsatile) at all ages and is magnified in low energy states, such as malnutrition (Soliman et al., 1986) and anorexia nervosa (Argente et al., 1997; Scacchi et al., 1997). Once a regular day/night cycle is established, there is a major burst of GH release 45–90 min following the onset of deep sleep (Martha, Gorman, Blizzard, Rogol, & Veldhuis, 1992). Generally, more than 75 % of the total daily production of GH accompanies sleep during the nighttime hours. However, this amount is dampened to a variable degree by the amount of body fat and its distribution in the subcutaneous vs. abdominal/visceral regions. There are significant increases in GH release during the newborn period, possibly related to a state of transient nutritional insufficiency (see Anorexia Nervosa, below) (Wright, Northington, Miller, Veldhuis, & Rogol, 1992) and more marked increases during adolescence (see below) (Martha et al., 1992).
Changes in GH release during pubertal maturation are large. There is an approximately threefold increase in the amount of GH released, which translates into a similar increase in serum IGF-I concentrations (Martha et al., 1992; Martha, Rogol, Veldhuis, & Blizzard, 1996; Roemmich et al., 1998). These increases are tied to the rising levels of sex steroids, but toward the end of pubertal maturation the GH secretory alterations return virtually to the prepubertal state (Kerrigan & Rogol, 1992). Variation in body composition and the regional distribution of fatness also influence GH release during puberty and into adulthood (Roemmich et al., 1998; Roemmich & Rogol, 1999; Veldhuis et al., 2005; Veldhuis, Roemmich, & Rogol, 2000).
Hypogonadism and Delayed Puberty
Constitutional Delay of Growth and Puberty
Constitutional delay of growth and puberty is a very frequent variant of normal pubertal maturation. Pubertal maturation is pathological if it has not started by 14 or 15 years of age (Styne & Grumbach, 2008). However, it is unlikely that the boy or his family would wait until that time for evaluation. When a boy presents for evaluation of delayed puberty it is more likely due to perceived psychosocial issues than those of growth or sexual maturation. Therefore, the precise incidence is undefined, perhaps because a majority of the boys do not come to medical evaluation. It is likely that only a minority are referred to an endocrinologist.
Constitutional delay of growth and puberty is characterized by a slowing of the growth rate (preadolescent dip), as well as by a delay in the timing and, perhaps, tempo of pubertal maturation. The patients are typically boys who seek medical evaluation in their early teenage years as they become aware of the discrepancy in linear growth and adolescent maturation compared with their age peers. Clinically, these boys have a height age (the age at which the patient’s height would be at the 50th centile) that is delayed but often concordant with the bone age, meaning that the height potential is normal. Sexual maturation lags behind that of the peers, and there is likely to be a family history of other “late bloomers.”
The suppressed HPG axis in children with CDGP represents an extension of the physiological hypogonadotropic hypogonadism present since infancy. Without intervention, most will undergo normal pubertal maturation spontaneously and most, but not all, will nearly reach their genetically determined mid-parental height (Crowne, Shalet, Wallace, Eminson, & Price, 1990). Pubertal maturation is likely to occur several years later than that of their peers. Many adolescents suffer significant emotional distress because they differ in their appearance from their peers during these years. Androgen therapy was initially proposed for boys with CDGP to alleviate their psychological discomfort, in addition to the beneficial effects on bone mineral accrual, lean body mass, protein metabolism, and the regional distribution of body fat.
Psychological benefits of treatment were examined in the Oakland Growth Study (Gross & Duke, 1980), in which 16 early- and 16 late-maturing boys were followed from age 11 years until adulthood. Multiple behavioral and social characteristics were assessed by peer ratings, adult perceptions, and psychological projective tests. The late-maturing boys were characterized as less mature, less attractive, and more talkative than young boys. As adolescents, the late-maturing boys expressed more feelings of inadequacy and rejection, and needed more social acceptance. Many of these traits persisted into adulthood. The National Health Examinations Survey found that pubertally delayed boys had lower teacher ratings of intellectual ability, lower intellectual test scores, and lower parental expectations for educational achievement than normally maturing boys in all age groups from 13 to 17 years (Duke et al., 1982). This makes a strong case for aggressive therapy in boys with CDGP, especially older ones. Other studies have shown no significant residual effects of delayed puberty; however, retrospectively, many of those not treated wished that they had received treatment. Despite these conflicting studies, the authors recognize that the majority of boys are anxious to begin androgen therapy and are generally pleased with the results, albeit subtle, even after 3 months of therapy with 50–75 mg long-acting testosterone esters per month. Their reasons to begin therapy fall into the appearance (too young), social (not considered a peer), and athletic (cannot compete because of size and lack of strength) spheres.
Pathologic Causes of Hypogonadism (Table 5 )
Table 5
Pathologic causes of hypogonadism
Hypogonadotropic hypogonadism |
---|
Traumatic brain injury |
Hyperprolactinemia |
Exercising adolescent and adult women (female athlete triad) |
Anorexia nervosa |
Infiltrative and inflammatory diseases of the hypothalamus and pituitary |
Pituitary |
Granulomatous |
Sarcoidosis |
Histiocytosis |
Metastasis (rare in adolescence) |
Breast |
Lung |
Gastrointestinal tract |
Hypophysitis |
Lymphocytic (especially postpartum) |
Xanthomatous |
Lipid-laden macrophages |
Hypothalamus |
Histiocytosis |
Sarcoidosis |
Infectious (multiple pathogens) |
Combined pituitary hormone deficiencies (with gonadotropin deficiency) |
Multiple causes |
Isolated hormone abnormalities |
Kallmann syndrome and normosmic idiopathic hypogonadotropic hypogonadism |
KAL1 mutation |
GPR54/kisspeptin mutation |
Fibroblast growth factor receptor 1 mutation |
GnRH mutation |
GnRHR mutation |
Hypergonadotropic hypogonadism |
Congenital hypergonadotropic hypogonadism |
Disorders of sex chromosome number |
47,XXY (Klinefelter syndrome) and its variants |
45,X (Turner syndrome) and its variants |
47,XXX |
XY and XX gonadal dysgenesis |
Complete androgen insensitivity syndrome |
Galactosemia |
Resistance to gonadotropins |
Acquired hypergonadotropic hypogonadism |
Radiation exposure |
Chemotherapy |
Trauma |
Infection |
Autoimmune oophoritis |
Central Hypogonadism
Traumatic Brain Injury
Traumatic brain injury (TBI) affects a growing number of children and adolescents, with an incidence of at least 180–250 per 100,000 children each year and with infants/toddlers and adolescents being the most commonly affected (Bruns & Hauser, 2003; Langlois, Rutland-Brown, & Thomas, 2005). Disordered pituitary function, especially for the GH and HPG axes, is prevalent in adult survivors of TBI (Agha et al., 2004; Agha, Sherlock, Phillips, Tormey, & Thompson, 2005; Aimaretti et al., 2004, 2005; Leal-Cerro et al., 2005), but has only recently been reported in children and adolescents in case-reports and case-series (Einaudi et al., 2006; Niederland et al., 2007; Poomthavorn, Maixner, & Zacharin, 2008).
Among abnormalities in pituitary function after TBI in children, growth hormone deficiency (GHD) appears the most common (Einaudi et al., 2006; Niederland et al., 2007; Poomthavorn et al., 2008). GHD not only may affect linear growth but also has been implicated in lipid abnormalities and body composition alterations in children and adolescents (Boot, Engels, Boerma, Krenning, & De Muinck Keizer-Schrama, 1997; Gleeson et al., 2007; Hulthen et al., 2001; Roemmich, Huerta, Sundaresan, & Rogol, 2001) and associated with cognitive impairments and poor recovery from TBI (Bavisetty et al., 2008; Behan, Phillips, Thompson, & Agha, 2008; Bondanelli et al., 2007; Kelly et al., 2006; Popovic et al., 2004). Knowledge of the associated features of GHD after TBI in children and adolescents may improve the ability of treating physicians to recognize and refer patients suspected of having pituitary abnormalities, especially GHD, for appropriate diagnostic evaluation and treatment.
To date, there have been few prospective studies that assess for GHD in children and adolescents who have sustained a TBI (Einaudi et al., 2006; Kaulfers et al., 2010). The former was only partially prospective and showed a much lower incidence of hypopituitarism (∼30 %) than some of the retrospective studies. The latter showed a varying incidence, 15 % at 1 month, 75 % at 6 months, but 29 % at 1 year. After 12 months only 5 % had GH deficiency. Our preliminary data indicate that GH deficiency is found in ∼16 % of children and adolescents who sustained moderate-to-severe head trauma (Norwood et al., 2010). Those with GH deficiency exhibited more rapid weight gain following injury than those with a normal GH/IGF-I axis (∼25 kg/year vs. ∼0 kg/year).
Prolactin
Abnormalities of prolactin (PRL) secretion are well-known causes of disordered puberty and reproductive function. PRL is produced in the anterior pituitary gland. It is unique among the pituitary hormones in that it is secreted tonically and undergoes inhibition by hypothalamic dopamine release, rather than being secreted only after stimulation by a trophic hormone as are most anterior pituitary hormones. Additional physiologic factors influencing PRL secretion include estradiol and thyrotropin-releasing hormone.
At physiologic concentrations, PRL acts to increase milk production in lactating women and also stimulates mammary gland development during puberty; however, PRL does not appear to influence normal breast maturation during puberty. Pathologically elevated PRL concentrations have a suppressive effect on GnRH secretion, thus decreasing LH and FSH levels and leading to insufficient production of estradiol in females and testosterone in males. In adolescents, these endocrine changes manifest as delayed or arrested pubertal maturation or primary or secondary amenorrhea in females. Males may have additional evidence of decreased testosterone, including decreased libido, erectile dysfunction, or infertility. Hyperprolactinemia is associated with gynecomastia in males, not as a result of direct stimulation of breast growth but due to hypogonadism and a decrease in the androgen/estrogen ratio. Galactorrhea, or milky breast discharge, may occur as a symptom or sign of hyperprolactinemia. This finding occurs in about 50 % of female patients and 35 % of males (Melmed & Kleinberg, 2008).
Hyperprolactinemia may be detected in a variety of conditions. Most commonly, it is a result of treatment with one of many medications. These include agents used to treat neurologic or psychiatric conditions, including anticonvulsants (phenytoin), selective serotonin reuptake inhibitors (fluoxetine), tricyclic antidepressants (amitriptyline), opiates (methadone), phenothiazines (chlorpromazine), and other medications known to decrease the actions of dopamine and serotonin (olanzapine, risperidone). Hyperprolactinemia may also occur in association with polycystic ovary syndrome, hypothyroidism, and renal failure. Central nervous system causes include conditions that prevent normal dopamine inhibition of PRL secretion, such as traumatic, neoplastic, or inflammatory injury of the pituitary stalk. Pituitary tumors may cause hyperprolactinemia, either from direct secretion of PRL from the tumor (prolactinoma) or from compression of the surrounding gland from a non-PRL-secreting pituitary adenoma.
Prolactinomas are the most commonly identified functional pituitary tumors, with an annual incidence of 6/100,000 and a prevalence of 10 per 100,000 in the general population (Colao & Lombardi, 1998). In the pediatric and adolescent age group, prolactinomas make up about 50 % of all pituitary adenomas (Colao, 2009). They occur far more commonly in females, especially during adolescence. Prolactinomas are divided into two classes: microprolactinomas, measuring ≤10 mm diameter, and macroprolactinomas, measuring >10 mm. Macroprolactinomas typically secrete more PRL, and serum concentrations typically are >200 ng/mL. Patients with microprolactinomas usually have PRL levels between 50 and 200 ng/mL. Serum PRL concentrations <100 ng/mL typically occur with non-tumoral causes of hyperprolactinemia, but may occasionally occur in association with small microprolactinomas.
Measurement of a serum PRL level is a standard part of the evaluation of delayed puberty, amenorrhea, or oligomenorrhea. If elevated, magnetic resonance imaging of the head should be the next step, particularly if the concentration is high or if there is no other explanation. Treatment of hyperprolactinemia is indicated if sexual or reproductive function is impaired or if galactorrhea is problematic. Treatment involves removal of the offending agent if possible. Prolactinomas are usually treated with dopamine agonists, such as bromocriptine or cabergoline. Surgical therapy is rarely indicated.
Female Athlete Triad
Adolescent girls and young women who participate in physical activities and sports, especially those that emphasize a lean physique, may be prone to disordered eating, primary or secondary amenorrhea, and low bone mineral density (osteopenia or frank osteoporosis), denoted collectively as the female athlete triad. Although core functions are maintained until the lowest levels of energy availability, some of the less immediately necessary processes, e.g., growth and reproductive function, are diminished. Thus, some aspects of the body’s adaptation to low energy availability tend to restore energy balance and promote survival but impair health.
The evidence for this disorder comes from a number of studies in young adult women (see ACSM statement for review, Nattiv et al., 2007) but clearly is relevant to the maturing adolescent, for whom there is strong evidence that the hypothalamic-pituitary-end organ axes are more susceptible to perturbation by a low energy balance, than in the more mature young woman.(Loucks, 2006) An elegant series of experiments done by Dr. Anne Loucks and her associates has likely determined the pathophysiologic basis for at least the disordered HPG axis physiology in women with the female athlete triad and also perhaps the underlying heightened susceptibility in the adolescent athlete (Loucks, 2006; Loucks & Thuma, 2003). If the energy availability is insufficient to support physiologic processes after the energy expenditure of athletic training, then there is less energy available for cellular maintenance, thermoregulation, growth, and reproduction (Wade, Schneider, & Li, 1996).
The health-related consequences of a deficit of energy output compared to intake are potentially irreversible and the operative imperative is to prevent the consequences by early diagnosis and appropriate treatment. Except for stress fractures, the consequences are most often delayed in time, and are mediated by failure to accrue an appropriate peak bone mass, causing osteoporosis of greater severity and at younger ages than in the general population. The likely pathogenesis is that low energy availability directly impairs bone health and maturation by suppressing the hormones that promote bone formation. Indirectly, low energy availability leads to primary or secondary amenorrhea, removing estrogen’s restraint on bone resorption. Bone mineral accrual is diminished for a long enough interval to develop wide-ranging deficits in bone mineral content.
Similarly, there is significant variability in the sensitivity of the hypothalamic-pituitary-gonadal axis suppression by energy deficits, thus failing to preserve body growth and energy storage for longer-term energy requirements such as the menstrual cycle, pregnancy, and lactation (Nattiv et al., 2007). In addition, the hypothalamic-pituitary-thyroid axis is altered to preserve energy (Nattiv et al., 2007).
Treatment of the female athlete triad is often difficult. The most direct approaches are to prescribe greater caloric intake or to decrease exercise energy expenditure. These are difficult for a highly competitive athlete, likely a gymnast, dancer, or long-distance runner. The American Academy of Pediatrics Committee of Sports Medicine and Fitness, 1999–2000, has presented a series of recommendations. Those relevant to the female athlete triad are shown in Table 6.
Table 6
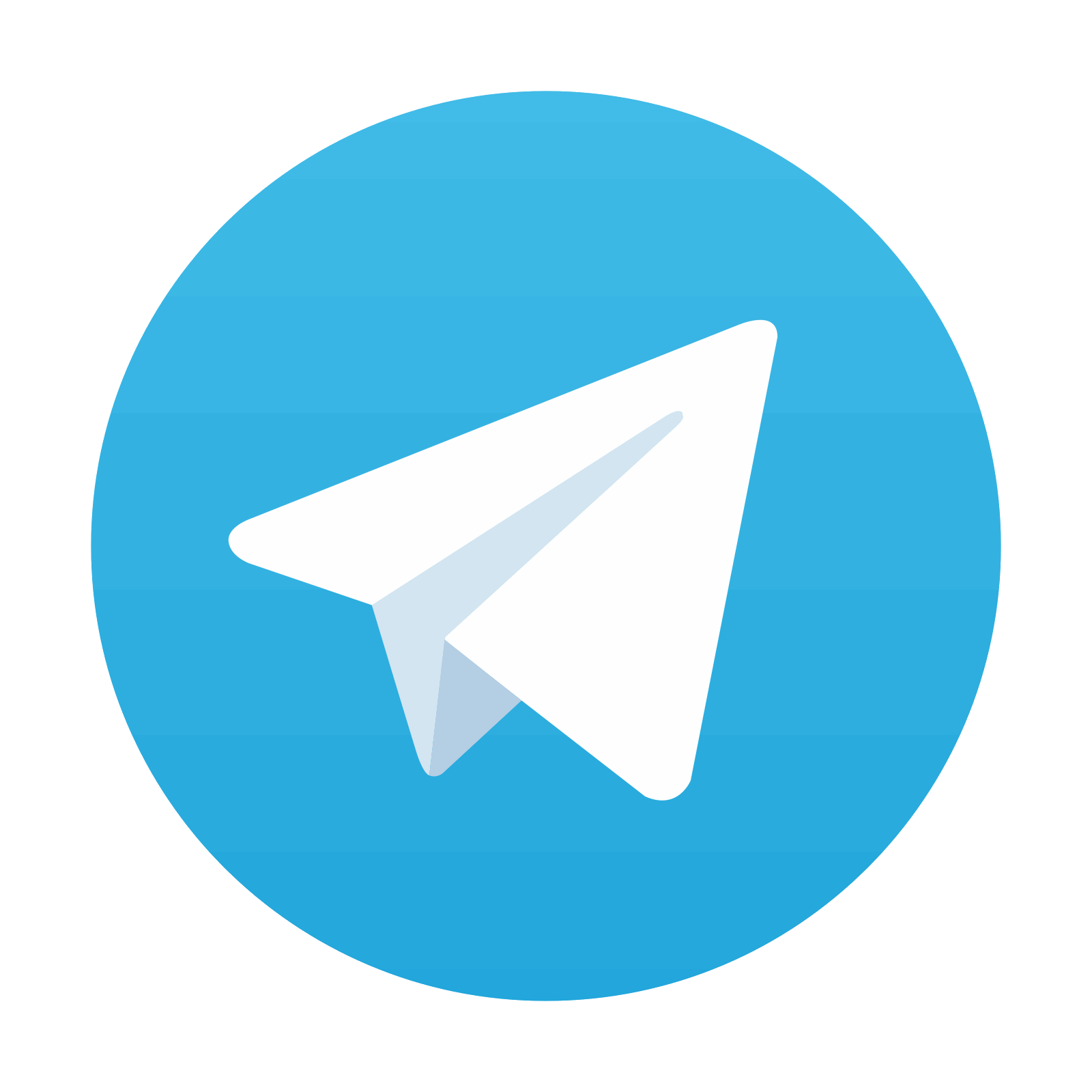
Guidelines for assuring safe and healthy sports participation for children and adolescents
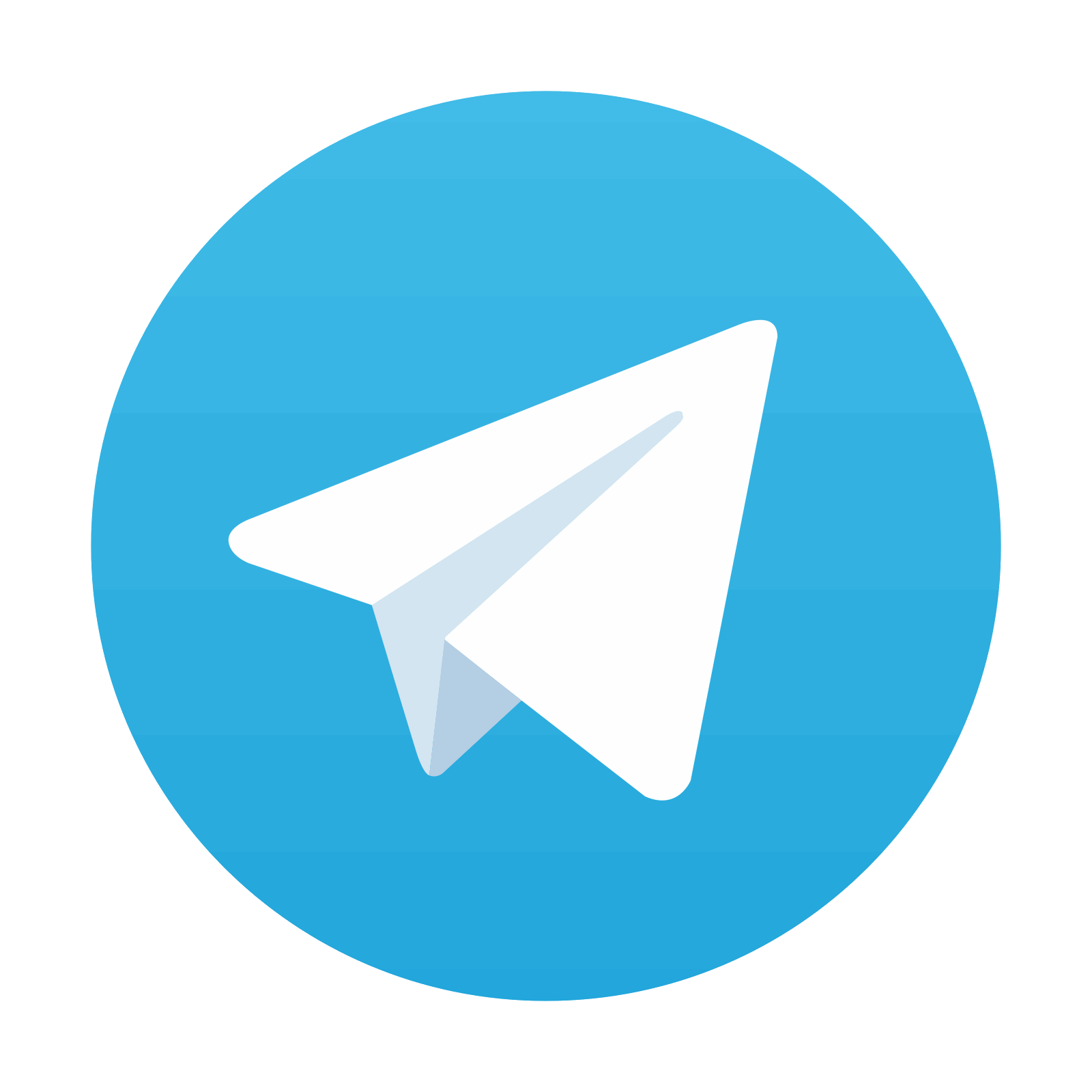
Stay updated, free articles. Join our Telegram channel

Full access? Get Clinical Tree
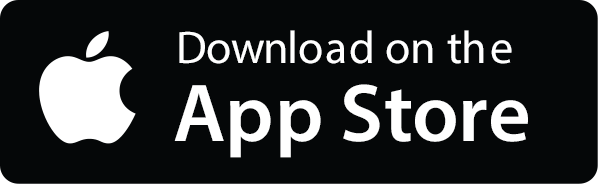
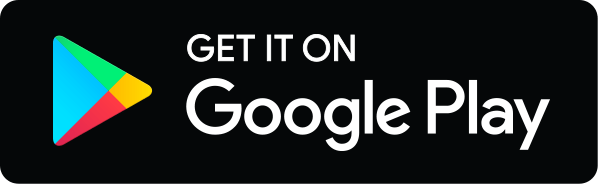
