Fig. 7.1
Descending pathways from a variety of motor-related cortical areas to spinal motoneurons and location of lesions made in the previous studies. Connection from the midline-crossing axons from the contralateral corticospinal tract and commissural interneurons (coINs) was not clarified before (marked as “?” in this figure). CMA cingulate motor area, DLF dorsolateral funiculus, coin commissural interneurons, M1 primary motor cortex, MC motor-related cortices, MN motoneuron, PM premotor cortex, PN propriospinal neuron, RSN reticulospinal neuron, RuSN rubrospinal neuron, S1 primary somatosensory cortex, SMA supplementary motor area
7.2.1 Primary Motor Cortex (M1)
Effects of lesion of the primary motor cortex (M1) were investigated in a large number of studies [23–27]. Among them, the ability of finger dexterity was investigated in detail in a most recent study by Murata et al. [28]. In this study, following mapping of the somatotopical organization of the M1 with intracortical microstimulation (ICMS) technique, the hand region was destroyed by injection of ibotenic acid. Following the lesion, digit movements were severely impaired, but with daily rehabilitative training, the ability of precision grip recovered in 3–4 month period. Interestingly, the recovery course took two steps; initially, the monkey tended to make “alternate grip” with which a small spherical food pellet was grasped between the tip of the index finger and the point around the metacarpophalangeal joint or interphalangeal joint of the thumb. However, gradually the monkey changed the strategy to grasp between the pads of the index finger and tip (distal to the interphalangeal joint) of the thumb (“precision grip”) and the alternative grip disappeared. But if the monkey was not sufficiently trained, they continued to use the alternative grip. In some of these monkeys, the brain activity during the recovery course was observed by using the positron-emission tomography (PET) and it was found that the activity of the ventral premotor cortex (PMv) on the lesioned side and the M1 area surrounding the lesion was enhanced during the recovery course [29]. Possible change in the somatotopic organization of the M1 and PM after the M1 lesion was studied by Liu and Rouiller by using ICMS [23]; however, any visible motor response was induced from the lesioned M1. On the other hand, inactivation of the PM on the lesioned side with muscimol suppressed the recovered dexterous movements, which suggested contribution of the PM to the recovery [29]. Based on the above results, a question may arise on how the PM contributes to the recovery. One possible explanation is the PM influences the motor function via the M1 as shown in the normal monkey [30] in the “hierarchical order” with the M1. Another possibility is that the PM directly influences the motor control via its direct projection to the subcortical centers and the spinal cord [31, 32] in the “parallel order” with the M1. At this moment, we have no clear answer to this question.
7.2.2 Brainstem Pyramid
“Pyramidotomy” at the level of the brainstem pyramid has been tested in a large number of studies (e.g., [33–35]). In these studies, dexterous hand movements were permanently impaired. In Lawrence and Kuypers study [35], the authors hypothesized that the recovered movements (less dexterous) must be controlled by the descending pathways mediated by the brainstem nuclei such as the red nucleus and pontomedullary reticular formation. Additional lesion of the lateral brainstem pathway (namely the cortico-rubrospinal pathway) and ventromedial brainstem pathway (cortico-reticulospinal pathway) revealed that interruption of the former resulted in the impairment of hand movements, while interruption of the latter pathway resulted in a severe impairment of movements in the proximal extremity. These findings suggested that the recovery of hand movements after the pyramidotomy was chiefly compensated by the cortico-rubrospinal pathway. In humans, Bucy et al. [36] reported the results of transection of the cerebral peduncle in patients with dyskinesias and showed recovery of limb movements. Bucy discussed that such recovery was due mainly to the subcortical pathways.
On the other hand, more recently, Baker and colleagues investigated the role of the cortico-reticulospinal pathway for recovery from lesion of the brainstem pyramid. They showed that the amplitude of the ecitatory synaptic potentials evoked by electrical stimulation of the reticulospinal tract in the motoneurons of hand muscles was facilitated [37], which suggested plasticity enhancing the transmission through the reticulospinal tract. Based on these findings, it was proposed that not only the cortico-rubrospinal tract but also the cortico-reticulospinal tract is responsible for the recovery of hand movements after stroke [38].
7.2.3 Mid-lower Cervical Segment (C4–C5) vs Upper Cervical Segment (C2)
In lower mammals, the corticospinal tract (CST) does not make direct connections with motoneurons. The shortest pathway to forelimb motoneurons in a cat, for example, is disynaptic, with a substantial portion of the disynaptic excitation being mediated by propriospinal neurons (PNs) located in the mid-cervical segments, C3–C4 (C3–C4 PNs) [39–41]. The existence of such PNs in primates has been doubted, primarily because disynaptic excitation of motoneurons was rarely observed in either intracellular recordings from motoneurons in anesthetized monkeys [42, 43] or in EMG recordings from hand muscles in awake monkeys [44] following stimulation of the CST or the motor cortex. However, it has been shown that disynaptic corticospinal excitation, which was mediated by C3–C4 PNs, became evident after glycinergic inhibition was reduced by intravenous injection of strychnine [45] and the existence of C3–C4 PNs, which receive monosynaptic pyramidal excitation and transmit the excitation to forelimb motoneurons, has been demonstrated in anesthetized monkeys [46]. The reason why the disynaptic excitation was not observed without strychnine was suggested to be that feedforward inhibition from the CST to the C3–C4 PNs was so potent that stimulation of the brainstem pyramid caused substantial disynaptic inhibition. It prevented the PNs from firing to the pyramidal stimulation; the monosynaptic excitation might be substantially curtailed by the following disynaptic inhibition [46]. The C3–C4 PNs have also been shown to exist in humans, and similarly, potential feedforward inhibition from the CST has been demonstrated in this system [47]. Thus, the existence of the C3–C4 PNs has been shown in electrophysiological experiments in anesthetized monkeys, but only after application of strychnine. As the next step, the functional significance of the PNs was needed to be clarified in behavioral studies. To study the role of the C3–C4 PNs in the control of fractionated digit movements, we investigated the effect of lateral corticospinal tract (l-CST) lesion at the C5 and C2 segments in macaque monkeys. First, selective lesion of the dorsolateral funiculus which covers the location of the l-CST was made at C5 segments. Immediately after the lesion, the monkeys showed deficit in precision grip. However, fractionated digit movements could still be observed immediately after the lesion (<2 weeks) in all the animals. In these animals, precision grip recovered in a few weeks to a few months (Fig. 7.2). Until now, we have tested the similar lesion in more than 20 monkeys and all of them showed recovery of precision grip to the success ratio higher than 90 %. In contrast, in the animals with similar lesion at the C2 level, the monkeys showed no trace of fractionated digit movements immediately after the lesion. Comparison of the hand movements immediately after the l-CST lesion at C5 and C2 suggests that the C3–C4 PNs can mediate the command for the precision grip in intact animals [48]. In these animals, longer post-lesional observation revealed limited recovery of fractionated digit movements, similar to the lesion brainstem pyramid reported by Lawrence and Kuypers [35] (unpublished observation by the same authors as Alstermark et al. 2011). All these results suggested that the PNs are involved in the control of fractionated digit movements and also their long-term recovery after the l-CST lesion at C5.
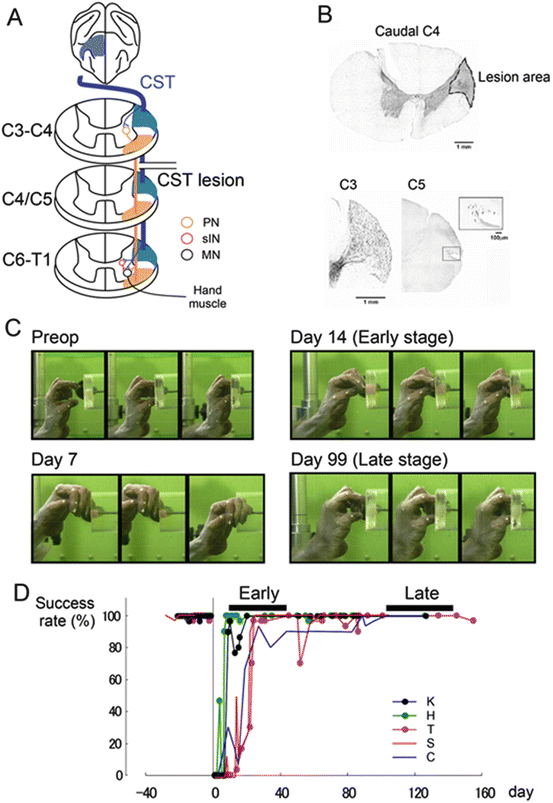
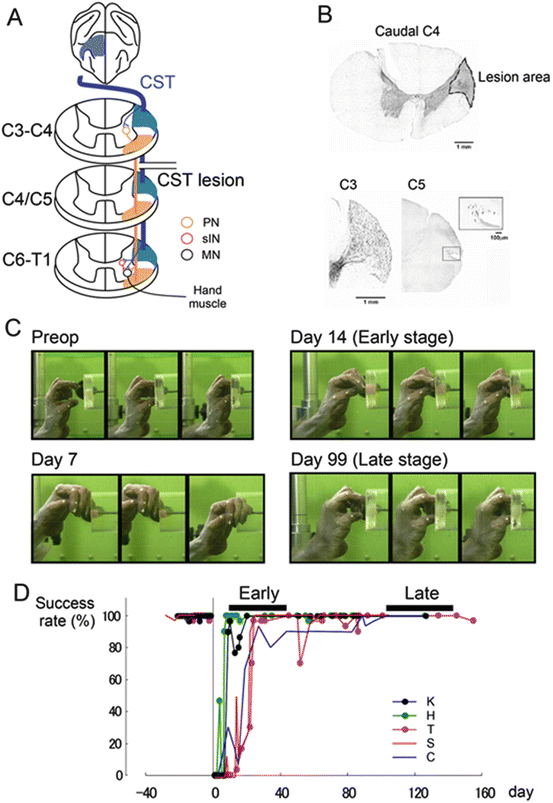
Fig. 7.2
Animal model of spinal cord injury in macaque. (a) Diagram of the presumed circuits after transection of the lateral corticospinal tract (l-CST). The direct cortico-motoneuronal (CM) connection via the l-CST was transected at the border between C4 and C5 segments of the spinal cord, which is rostral to the segments where motoneurons of hand muscles are located (“C4–C5 l-CST lesion”). A large proportion of indirect CM pathways, except for those mediated by segmental interneurons, remained intact in the present preparation. Segmental interneuron and C3–C4 PNs are indicated as a red and orange circle, respectively. (b) The extent of the l-CST lesion which showed the smallest lesion area in the recent study. The CST lesion at the caudal C4 (Nissl staining). Dotted line indicates the border between the lesioned and intact tissue. BDA-labeled CST axons at C3 (rostral to lesion). A few BDA-labeled CST axons labeled at the rostral C5 (box) (caudal to the lesion). (c) Recovery of precision grip. Representative frames showing the retrieval of a small piece of food. These images were taken at 10 frames/s. (d) Recovery time course of success ratio for precision grip in five monkeys (From Nishimura and Isa[49], reproduced with permission)
7.2.4 Lower Cervical Hemisection
Several groups investigated the effects of hemisection at the lower cervical level (C5–C7) [50–54]. These studies showed that the monkeys exhibited severe flaccid hemiparesis during the first month, and predominant recovery of the affected limb could be observed later. However, the deficit in hand dexterity remained. Recently, Rosenzweig et al. [53] showed extensive sprouting of midline-crossing axons from the corticospinal fibers descending in the intact side of the spinal cord, which may underlie the recovery of hand movements.
7.2.5 Other Lesions
There is no systematic report on the effect of damage to the internal capsule in the nonhuman primate model, presumably because of difficulty in making an appropriate lesion of such a large fiber tract located subcortically. In humans, the cerebral infarction frequently occurs around the internal capsule. Fries et al. [55] studied patients with capsular or striatocapsular stroke and found that lesion of the anterior (plus caudate/putamen) or posterior limb of the internal capsule led to an initially severe motor impairment; however, it is mostly followed by excellent recovery in hand function. In contrast, lesions of the posterior limb in combination with the lateral thalamus compromised the motor outcome. They subsequently studied the trajectories of the corticofugal axons of the M1, PM, and SMA with an anterograde tracer in macaque monkeys. It was found that axons from each cortical area were differentially localized in the internal capsule; those from the M1 passed through the middle third of the posterior limb, those from the PM passed through the capsular genu, and those from the SMA passed through the anterior limb. Thus, if the lesion covers the whole capsule, the impairment should be severe; however, if a part of the capsule spared the lesion, considerable recovery can be expected. These results support the “parallel order” rather than “hierarchical order” operation of cortical motor areas, which provides the capability of substituting each other in the process of recovery.
7.3 Mid-cervical l-CST Lesion: Plasticity at Different Hierarchical Levels
As described above, the hand dexterity showed marked recovery after the CST lesion at the C4–C5 segments. We used this model to study the plasticity during the recovery of hand dexterity. Actually, the plasticity was found not only at the spinal cord level but also in higher-order structures. We will summarize the results below.
7.3.1 Spinal Cord
After recovery, terminal electrophysiological analyses were conducted to investigate the transmission from the brainstem pyramid to spinal motoneurons under anesthesia. Intracellular recordings were made from forelimb motoneurons in the C6–C8 segments, including deep radial motoneurons, and the effects of the pyramidal stimulation at the brainstem pyramid were investigated. Interestingly, disynaptic or oligosynaptic excitatory postsynaptic potentials (EPSPs) were found in about half of the motoneurons on the affected side [16]. In normal monkeys, it was necessary to administer strychnine which reduces the glycinergic inhibition to unmask such synaptic effects in motoneurons [45]. Such effects were abolished by additional l-CST lesion at C2, suggesting that these EPSPs were mediated by interneurons caudal to the C2, the PNs. These results suggested that the signal transmission through the PNs was enhanced. Physiological and neuroanatomical basis for such plasticity is still unclear.
The functional coupling between the activity of M1 and forelimb muscles was investigated throughout the recovery course by recording the local field potential in the hand and arm area of the M1 and electromyography of hand and arm muscles [17]. The cortico-muscular coherence with the peak at 17 Hz (β-band) could be recorded while the monkey was performing isometric grip with the index finger and thumb as previously reported both in monkeys and humans [56–58]. The cortico-muscular coherence disappeared completely after the C5 l-CST lesion. It did not recover 3 months after the lesion, even though the success ratio of precision grip was recovered almost completely. On the other hand, the musculo-muscular coherence at the γ-band (peak at 33 Hz) started to appear and increased in parallel to the recovery of the precision grip (Fig. 7.3). Causality of such coherent activity to the functional recovery was not demonstrated. But the time course of appearance of the musculo-muscular coherence was closely related to that of the functional recovery. These results suggested that the γ-band musculo-muscular coherence should be derived through reorganization of the subcortical structures such as the spinal cord and brainstem. Neurophysiological basis of such reorganization remains unclear.
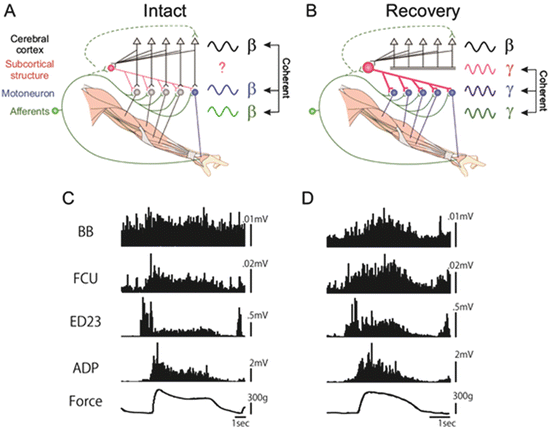
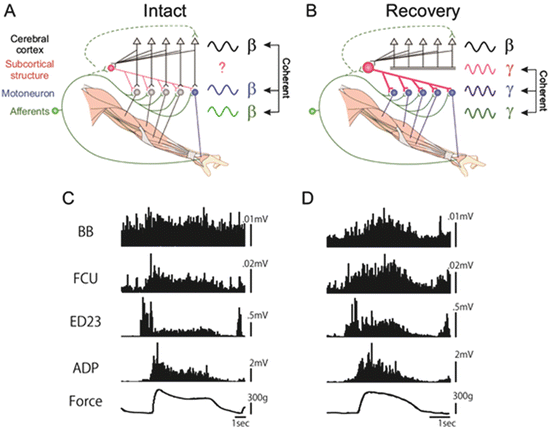
Fig. 7.3
(a and b) Schematic drawing of disappearance of the cortico-muscular coherence and appearance of the musculo-muscular coherence during recovery after the l-CST lesion. (c) EMG recordings before lesion. (d) EMG recordings recorded on day 91 after the lesion. FCU flexor carpi ulnaris, ED2,3 extensor digitorum 2,3, ADP adductor pollicis (Modified from [15])
7.3.2 Cerebral Cortex
Brain imaging with PET with H2 15O was performed during reach and precision grip movements in three macaque monkeys (Fig. 7.4). In normal animals, high activation was observed in the sensorimotor cortex and frontoparietal stream on the contralateral side to the moving arm [18, 59]. After the lesion, during the early stage of recovery (1 month after the lesion) when the success ratio of precision grip recovered above 80 %, activation was increased in the hand representation areas of the bilateral sensorimotor cortex. In contrast, at the late stage of recovery (3–4 months after the lesion), when the recovery was more stabilized, the activation was enhanced in the contralesional sensorimotor cortex. In addition to the sensorimotor cortex, high activation was observed in the bilateral ventral premotor cortex (PMv); however, the increased activity in the ipsilateral M1 disappeared. Thus, the activation area changed depending on the recovery status. To demonstrate the causal relationship between the higher activation in each area detected by the PET and the functional recovery, reversible inactivation of these cortical areas (bilateral M1 and bilateral PMv) by microinjection of muscimol, a GABAA receptor agonist, was tested at preoperative, early recovery, and late recovery stages. Inactivation of contralesional M1 (coM1) caused severe deficit in reach and precision grip behavior all through the three stages. Especially, the deficit was most evident following inactivation of the coM1 during the early stage. Interestingly, the effect of inactivation of the coM1 was moderate or weaker at the late stage, suggesting that the areas such as the PMv or the M1 surrounding the digit area might have contributed to the recovery. On the other hand, interestingly, inactivation of the ipM1 caused no deficit at the preoperative stage; however, the same manipulation caused deficit in the precision grip at the early recovery stage. But at the late recovery stage, inactivation of ipM1 caused no effect. These results suggested that the ipM1, which does not play a significant role in the control of hand movements in the normal condition, is critically involved in the control of dexterous digit movements only during the early recovery stage. The results well fitted with the observation by the PET imaging. Inactivation of both contralesional PMv (coPMv) and ipsilesional PMv (ipPMv) with microinjection of muscimol caused no consistent deficit in two monkeys in both preoperative and early recovery stages. However, inactivation of ipPMv caused deficit consistently in two monkeys at the late recovery stage. These results clarified that the coM1, ipM1, and ipPMv play significant contribution to the different aspects of recovery [18]. Investigation of plasticity-related gene expression such as GAP-43 by in situ hybridization showed enhanced expression of GAP-43 in the laminae II/III of the M1 and PMv and large cells (presumably the corticofugal neurons) in M1 on both sides in the animals with C5 l-CST lesion [60]. These results suggest that the change in activation of bilateral M1 and PMv accompanies the morphological change with neurite extension in the cortico-cortical association circuits and descending corticofugal pathways originating from these areas.
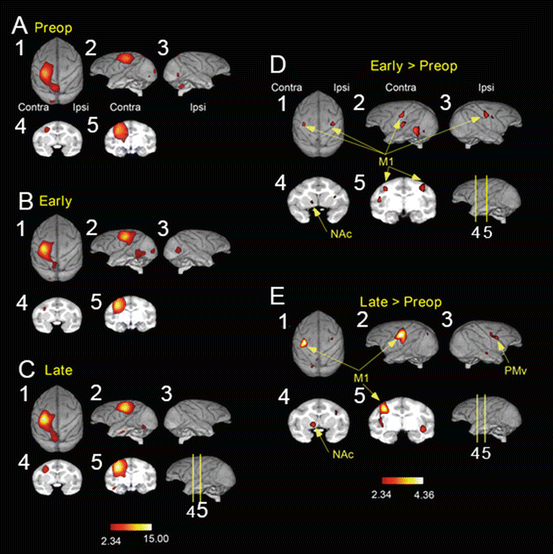
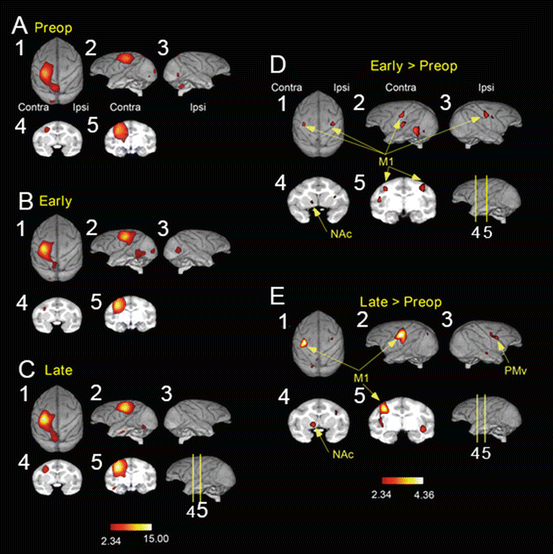
Fig. 7.4
Brain imaging of the cortical activity by PET during recovery from the C4–C5 l-CST lesion. (a) Before lesion (pre-op), (b) early recovery period (1 month, early), (c) late recovery period (late, 3–4 months), (d) difference in activity between the early recovery period and intact state (early > pre-op), and (e) difference in activity between the late recovery period and intact state (late > pre-op)
7.3.3 Further Beyond
In the brain imaging with PET, it was found that activity of the contralesional ventral striatum (VSt) including the nucleus accumbens (NAc) was increased during recovery after the C5 l-CST lesion. Higher activation was observed especially during the late recovery stage. The VSt comprises the neural network for the regulation of motivation together with the anterior cingulate cortex (ACC) and the orbitofrontal cortex (OFC). We evaluated the functional connectivity among the coM1, NAc, ACC, and OFC before the l-CST lesion and during recovery (Fig. 7.5, [19]). It was found that virtually no significant correlation could be detected in the intact animal. In contrast, significantly strong connectivity could be detected in various combinations of these areas during the recovery. Thus, these limbic structures became closely correlated with the motor cortex (coM1) not under the normal condition, but only during the recovery. It is well known that the VSt and M1 are indirectly connected with each other [61, 62]. To show the causal involvement of these limbic structures in the recovery process, manipulation of activity of the VSt at preoperative stage and during the recovery would be an interesting subject for future studies.
