The clinical features of the Lennox–Gastaut syndrome (LGS) have been recognized for more than 200 years. The current definition of LGS by the International League Against Epilepsy (ILAE) classification is: “LGS manifests itself in children aged 1–8 years, but appears mainly in preschool-age children. The most common seizure types are tonic-axial, atonic, and absence seizures, but other types such as myoclonic, generalized tonic–clonic seizures (GTCS), or partial seizures are frequently associated with this syndrome. Seizure frequency is high, and status epilepticus is frequent (stuporous states with myoclonias, tonic, and atonic seizures). The electroencephalography (EEG) usually has abnormal background activity, slow spike-waves less than 3 Hz and, often, multifocal abnormalities. During sleep, bursts of fast rhythms (10 Hz) appear. In general, there is mental retardation. Seizures are difficult to control, and the development is unfavorable. In 60% of cases, the syndrome occurs in children suffering from a previous encephalopathy, but is primary in other cases.”
A triad of basic elements for diagnosing LGS is typically based on this definition, and tailored by clinical experience and research:
Multiple types of seizures including tonic seizures, atypical absences, and atonic seizures
An EEG pattern consisting of interictal diffuse slow spike and wave discharges occurring at a 1.5–2 Hz frequency
Global cognitive dysfunction.
This is no consensus about the minimal necessary and sufficient criteria to diagnose LGS. Some investigators do not consider cognitive dysfunction indispensable for diagnosis, especially at onset, if the seizures and EEG pattern are typical. Others use stricter EEG criteria requiring that the diagnostic EEG pattern include bursts of generalized fast spikes (10 Hz) during nonrapid eye movement (NREM) sleep.
LGS accounts for 1%–4% of all cases of childhood epilepsy, but 10% of cases that start in the first 5 years of life. The annual incidence of LGS in childhood is 2 per 100,000 children while its prevalence ranges from 0.1 to 0.28 per 1000 in Europe and the United States. Males are affected more often than females but this gender difference is not statistically significant. There are no racial differences in the occurrence of LGS. The mean age at epilepsy onset is 26–28 months (range 1 day-14 years).
Patients are considered to have idiopathic LGS if there is normal psychomotor development before the onset of symptoms, if there are no underlying disorders or definite presumptive causes, and if there are no neurologic or neuroradiologic abnormalities. In contrast, patients have symptomatic LGS if there is an identifiable cause for the syndrome. Population-based studies reveal that 22%–30% of patients have idiopathic LGS, while 70%–78% have symptomatic LGS.
Pathologies responsible for LGS include encephalitis/meningitis, tuberous sclerosis, brain malformations (e.g., cortical dysplasias), birth injury, hypoxic–ischemic injury, frontal lobe lesions, and trauma. Infantile spasms precede the development of LGS in 9%–41% of cases. Some investigators consider cryptogenic cases in which there is no identified cause or when a cause is suspected and the epilepsy is presumed to be symptomatic as a distinct etiological category.
Cognitive dysfunction, psychiatric symptoms, and multiple seizures types are common clinical manifestations. Factors associated with cognitive dysfunction are symptomatic LGS, a previous history of West syndrome, onset of symptoms before 12–24 months of age, and higher seizure frequency. A significant correlation exists between age of onset of seizures and mental deterioration, with a favorable cognitive outcome more likely to occur in patients with a later age of LGS onset. Psychiatric symptoms in young children consist of mood instability and personality disturbances, while slowing or arrest of psychomotor development and educational progress characterize the neuropsychological symptoms. Character problems predominate in older children, and acute psychotic episodes or chronic forms of psychosis with aggressiveness, irritability, or social isolation may occur.
Several types of seizures occur in LGS including tonic, atonic, myoclonic, and atypical absences, often associated with other less common types.
Tonic seizures are said to be the most characteristic type of seizure occurring in 17%–95% of children with LGS. Tonic seizures are more frequent during NREM sleep and researchers who have systematically obtained sleep tracings report a higher prevalence. Tonic seizures may be subdivided into1 axial tonic characterized by involvement of the head and trunk with head and neck flexion contraction of masticatory muscles and eventual vocalizations2 axorhizomelic tonic characterized by tonic involvement of the proximal upper limbs with elevation of the shoulders and abduction of the arms and3 global tonic characterized by contraction of the distal extremities, sometimes leading to a sudden fall or mimicking infantile spasms.
The reported frequency of atypical absence seizures ranges from 17% to 100%. The frequency of seizure types in most studies is based on parental seizure counts and chart reviews or is unspecified. This retrospective methodology is prone to subjective bias as, for example, a parent’s limited ability to recognize and identify atypical absences correctly. Furthermore, atypical absences are often difficult to diagnose due to their subtle onset and incomplete loss of consciousness permitting continuation of ongoing activities.
Atonic seizures, massive myoclonic seizures, and myoclonic-atonic seizures are all typical of patients with LGS but may be difficult to differentiate by clinical observation alone. Their reported frequency ranges from 10% to 56%. All can result in sudden and precipitous falls associated with craniofacial injury (“drop attacks” and “Sturzanfalle”). Movement may be limited to the head only characterized by the head falling on the chest (“head drop,” “head nod,” and “nictatio capitis”). Notably, pure atonic seizures are the exception rather than the rule as most atonic seizures have been shown to have a tonic or myoclonic component.
GTCS are reported to occur in 15% of patients with LGS while complex partial seizures occur in 5%. Status epilepticus associated with several different seizure types (absence status epilepticus, tonic status epilepticus, nonconvulsive status epilepticus) is not uncommon and often displays prolonged duration and resistance to treatment.
The interictal EEG is characterized by a slow background that may be constant suggesting an underlying encephalopathy or more variable in presentation. The hallmark of the waking interictal EEG in LGS is the occurrence of generalized slow spike-wave discharges. This pattern consist of bursts of irregular bilateral spikes or sharp waves followed by a sinusoidal 35–400 milliseconds slow waves of high-amplitude ranging from 200 to 800 μV that can be symmetrical or asymmetrical. The amplitude is often higher in the anterior region and in the frontal or frontocentral areas but may predominate in the posterior head regions. The frequency of the slow spike-wave activity is typically between 1.5 and 2.5 Hz.
Slow spike-waves are usually not activated by photic stimulation. Hyperventilation rarely induces slow spike-waves, although cognitive delay may prevent adequate cooperation. During NREM sleep, discharges are more generalized, occur more frequently, and consist of polyspikes and slow waves. There is a decrease in spike-wave activity in REM sleep and a reduction in the total duration of REM sleep during periods of frequent seizures.
Several seizure types occur in LGS including tonic, atonic, myoclonic, and atypical absences, often in association with less common types. The tonic seizure EEG is characterized by a diffuse rapid (10–13 Hz), low-amplitude activity, mainly in the anterior and vertex areas (recruiting rhythm) that progressively decreases in frequency and increases in amplitude. The atypical absence seizure EEG is characterized by diffuse slow (2–2.5 Hz) and irregular spike-waves that may be difficult to differentiate from interictal bursts. The EEG in atonic seizures, massive myoclonic seizures, and myoclonic-atonic seizures is characterized by slow spike-waves, polyspike and waves, or rapid diffuse rhythms.22,26 Simultaneous video-EEG recording and polygraphy is often required for precise diagnosis. In 95% of affected patients, all three types of seizures occur in the same patient.
Neuroimaging is an important part of the search for an underlying etiology in patients with LGS. Magnetic resonance imaging (MRI) is the preferred neuroimaging modality and computerized tomography (CT) scanning is indicated only in highly selected situations (e.g., evaluation of suspected intracranial injury and/or hematoma in a patient with head trauma resulting from an atonic and/or tonic seizure). No current indications exist for routine positron emission tomography (PET) or single-proton emission computed tomography (SPECT) scanning in patients with LGS unless a localized primary epileptogenic zone is suspected as a source for secondary bilateral synchrony. In this situation, patients with LGS may become potential epilepsy surgery candidates and further work-up is fully justified.
The EEG evaluation of patients with suspected LGS is critical, since a correct diagnosis depends on the presence of specific electrographic findings. Recording of a prolonged EEG is desirable as a routine 30-minute tracing may not capture both awake and asleep electrographic activity and thus may miss crucial findings. It is important to capture and classify each of the patient’s multiple seizure types. Video-EEG telemetry should be strongly considered as it may also help to educate parents on which patient “events” are seizures and which are nonepileptic behaviors.
The goals of LGS treatment are the same as for all epilepsy patients: improving quality of life with the fewest seizures (hopefully none), the fewest treatment side effects, and the least number of medications. As LGS may be treatment resistant, achieving all of these goals is often challenging. Medication resistance typically leads to the use of polytherapy, more drug-drug interactions, increased side effects, and a reduced quality of life. Potential treatment modalities in LGS include pharmacologic, dietary, and surgical therapies. Traditionally, pharmacologic treatment is the first approach.
For decades, the paucity of pediatric epilepsy evidence-based data and clinical guidance resulted in clinicians relying frequently on clinical judgment. A survey sent to 41 American pediatric epileptoligists revealed that valproate (VPA) was the initial treatment of choice followed by topiramate (TPM) as the next option and lamotrigine (LTG) as another first line choice. A similar survey sent to 57 European physicians specializing in pediatric epilepsy concurred that VPA was the agent of first choice. If there was no response to VPA, the next choice was LTG with TPM being another first line selection. Significantly no randomized controlled studies with VPA have been undertaken in patients with LGS. Covanis and collaborators reported their findings on the open-label use of VPA in 336 patients, of which 38 had “myoclonic-astatic epilepsy.” VPA reduced the number of seizures, primarily drop attacks but also atypical absences and myoclonic seizures, with seven becoming seizure free.
The gold standard for evaluation of the safety and efficacy of an anticonvulsant medication is the randomized, double-blind, placebo-controlled clinical trial. During the past two decades, seven drugs have undergone this rigorous testing to determine safety and efficacy in patients with LGS: cinromide, intravenous immunoglobulin (IVIG), felbamate (FBM), LTG, TPM, rufinamide (RFM), and clobazam (CLB). The latter five antiepileptic drugs (AEDs) successfully demonstrated efficacy against seizures in patients with LGS, whereas the first two did not.
FBM was found to be effective and well tolerated in patients with LGS in a randomized, double-blind, placebo-controlled adjunctive therapy trial34 involving 73 patients aged 4–36 years. The target FBM dose in the double-blind portion was 45 mg/kg per day (maximum 3600 mg/day). The total seizure frequency decreased by 19% in the FBM group compared with a 4% increase in seizures in the placebo group (p < 0.002). Patients treated with FBM had a 34% reduction in atonic seizures, whereas patients receiving placebo experienced a 9% reduction (p < 0.01). The responder rate (percentage of patients experiencing at least a 50% reduction) for atonic seizures was 57% for the FBM group compared to 9% in the placebo group (p < 0.001). Likewise, the percentage of patients experiencing at least a 50% reduction in total seizure frequency was 50% for the FBM group compared to 11% in the placebo group (p < 0.001). Global evaluation scores were significantly better in the FBM-treated arm. Most side-effects were of mild or moderate severity. The types and frequency of side effects were similar in the two treatment groups. Long-term efficacy was confirmed in a 12-month follow-up of patients who completed the controlled part of the study.35
Unfortunately, treatment with FBM is hampered by the risk for idiosyncratic reactions including aplastic anemia and hepatotoxicity.36,37 The most common severe FBM-associated idiosyncratic reaction is aplastic anemia, with an incidence of approximately 127 cases per 100,000 treated with FBM (approximately 1 in 4000–8000 FBM-treated patients). This compares unfavorably with an incidence of 2–6 per 100,000 people in the general population.36,38,39,40 Other reports estimate the risk of aplastic anemia in patients receiving FBM to be 1:3000, with a death rate of 1 in 10,000 FBM-treated patients.39,41 In perspective, this estimated risk is approximately 20 times greater than that for carbamazepine-associated aplastic anemia.36 Factors that increase the risk of FBM-associated aplastic anemia include: Caucasian ethnicity, adult usage, female sex, history of autoimmune disorder, positive antinuclear antibody titer, history of prior AED toxicity or allergy, prior cytopenia, and treatment with FBM for less than 1 year.36,37,42
The second most common severe FBM-associated idiosyncratic reaction is hepatotoxicity, which was reported in 18 patients receiving FBM, with an estimated incidence of 64–164 per 100,000 (approximately 1 in 18,500–25,000 FBM-treated patients).36 This suggests a similar frequency of FBM-associated hepatotoxicity and VPA-associated hepatotoxicity.36 Based on the reported five FBM-related liver failure fatalities, which occurred in approximately 130,000–170,000 exposed persons, the estimated incidence lies between 1 per 26,000–34,000 exposures. In perspective, the risk of hepatic-related fatalities in the population taking VPA is between 1 in 10,000–49,000; the highest risk is 1 in 500 in patients younger than 2 years of age.37 Although there is no evidence that routine laboratory monitoring of blood counts and liver function during FBM therapy anticipates these severe idiosyncratic reactions,36 it is suggested that careful clinical monitoring and routine laboratory testing is performed, and the drug is discontinued if no significant clinical benefit is observed after 3–6 months of therapy.
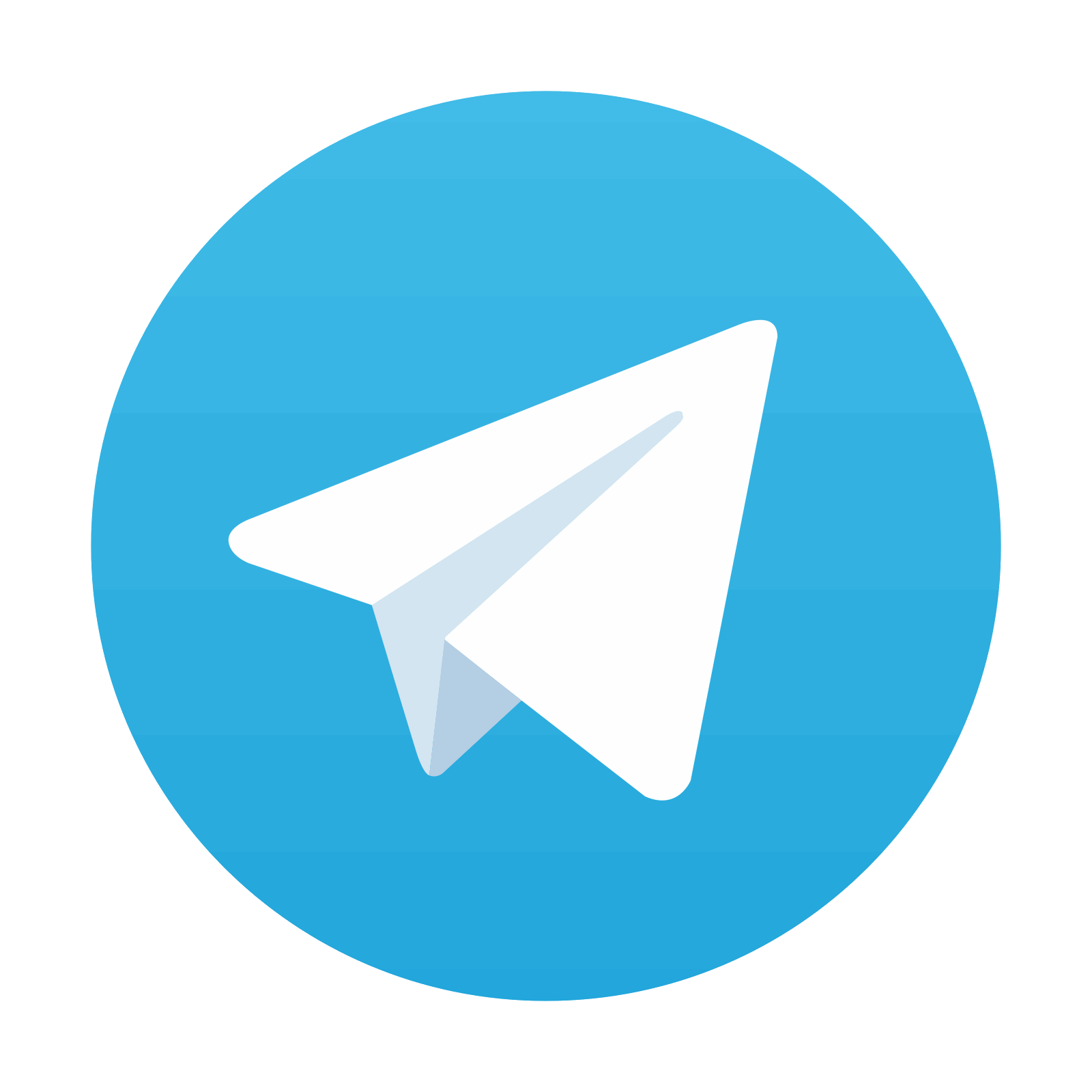
Stay updated, free articles. Join our Telegram channel

Full access? Get Clinical Tree
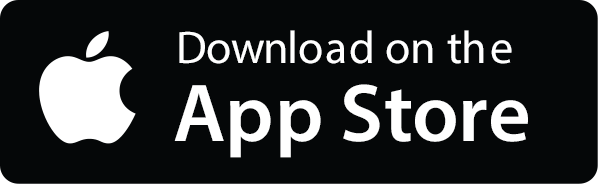
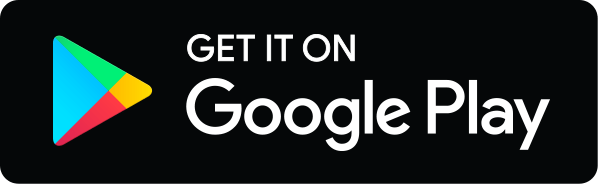