Type of cancer
%
Breast cancer
Lung carcinoma
Melanoma
Gastrointestinal tract cancer
Adenocarcinoma of unknown primary
12–34
10–26
17–25
4–14
1–7
Table 5.2
Frequency of leptomeningeal metastatic involvement by type of cancer
Type of cancer | Frequency of secondary LM (%) |
---|---|
Melanoma Small cell lung cancer Breast carcinoma Non-small cell lung cancer Head and Neck | 22–46 10–25 5 1 1 |
Although non-small cell cancer lung (NSCLC) and melanoma show a tropism for the leptomeninges (11% and up to 23% respectively) [18, 19], the incidence of LM secondary to breast cancer (BC) is higher, notwithstanding an overall 5% rate of spread to the CSF and leptomeninges [1, 5, 20]. Negative estrogen receptor (ER) , negative progesterone receptor (PR), triple-negative status, and an infiltrating lobular component are all risk factors for LM in BC patients [21–27]. In HER2 positive patients, opposite to the well-established increased incidence of intraparenchymal brain metastases, LM is relatively infrequent with an incidence of 3–5% [28]. According to a recent retrospective study, the occurrence of pulmonary metastases is associated with development of LM in breast cancer [29]. Another retrospective series concluded that breast cancer patients undergoing resection of a brain metastasis had a high risk of leptomeningeal disease [30]. Progressive systemic disease and age younger than 40 years at the time of brain metastasis treatment have also been associated with a higher risk of developing LM in BC patients [31].
Risk factors predisposing metastatic spread to leptomeninges include piecemeal surgical resection of parenchymal cerebellar lesions, and resection of supratentorial BM resulting in a breach of the ventricular system [31–37]. In both situations, direct spillage of tumor cells into the CSF and subsequent dissemination of malignant cells is the presumed mechanism. Improvement in systemic therapy resulting in prolonged survival of patients is another factor that contributes to the increased incidence of LM. By example, the incidence of LM in melanboma has increased since the introduction of recently approved drugs such as anti-cytotoxic T-lymphocyte antigen (CTLA)-4 and PD-1 antibodies and BRAF inhibitors that have improved overall survival (OS) and control of extracranial disease [16]. The poor central nervous system (CNS) penetration of targeted therapies such as antibodies (e.g., trastuzumab in BC) is another factor contributing to the increased incidence of LM [38–41]. The meninges and cerebrospinal fluid (CSF) compartment is a pharmacological sanctuary for many cytotoxic agents that have limited ability to cross an intact blood–CSF barrier.
Pathogenesis
There are many ways by which malignant cells can spread to the leptomeninges: (1) hematogenous spread , mostly by arterial contamination or via the venous plexus of Batson in pelvic cancers; (2) direct dissemination from adjacent BM or primary brain tumors; (3) through centripetal migration, along perineural or perivascular spaces. The latter mechanism is mostly observed in head and neck cancers, and in breast and lung cancers, when there are known vertebral and paravertebral metastases [42–44].
Once circulating tumor cells (CTC) have breached the subarachnoid space (SA) , CTC spread through CSF, resulting in diffuse and multifocal seeding of the leptomeninges. The base of brain (especially the basilar cisterns) and dorsal surface of the spinal cord (especially the cauda equina) are predominantly affected [45, 46]. The CSF outflow may be obstructed by ependymal nodules or tumor deposits leading to a communicating hydrocephalus. Obstruction of CSF corridors affects predominantly the IVth ventricle, basal cisterns, cerebral convexity, and spinal SA.
Diagnosis
It is important to diagnose LM in its initial stage, when neurological impairment is minimal, the patient performance status (PS) is maintained, and the tumor burden is low as these factors are correlated with response to treatment and OS.
Clinical Features
LM typically presents with pleomorphic neurological signs and symptoms. The clinical manifestations of LM typically reflect the simultaneous involvement of three distinct neurologic compartments: (1) the cerebral hemispheres; (2) the cranial nerves; and (3) the spinal cord and exiting roots (Table 5.3).
Table 5.3
Most frequent symptoms and signs in patients with LM from solid tumors
Symptom | Initially (%) | At any time (%) | Sign | Initially (%) | At any time (%) | |
---|---|---|---|---|---|---|
Cerebral | Gait difficulty Headache Mental change Nausea and vomiting | 46 38 25 12 | 68 40 30 20 | Extensor plantar Impaired mental state Seizures Papilledema | 50 50 14 12 | 66 50 15 12 |
Cranial nerve | Diplopia Visual Loss Hearing Loss Dysphagia | 8 8 6 2 | 20 12 9 4 | Occular muscle palsy Facial weakness Hearing Loss Trigeminal Neuropathy | 30 25 20 12 | 38 26 20 14 |
Spinal | Pain Back pain Radicular pain Paresthesia Weakness | 25 18 12 10 22 | 40 50 25 42 50 | Lower motor neuron weakness Reflex absence/decrease Dermatomal sensory loss Nuchal rigidity | 78 60 50 16 | 78 76 50 17 |
Headache and mental status changes are the most frequent symptoms linked to cerebral hemispheres involvement, followed by confusion, dementia, seizures, and hemiparesis. Diplopia is the most frequent symptom, caused by cranial nerves impairment with a predominant involvement of the VIth cranial nerve followed by cranial nerve III and IV. Trigeminal sensory or motor dysfunction, cochlear impairment, and optic neuropathy are less common. Weakness (mostly of the lower limbs), dermatomal, segmental, or radicular sensory impairment, and pain reflect spinal involvement. In contrast to infectious meningitis, the occurrence of nuchal rigidity is unusual (15% of patients) [2, 5, 6, 16]. Symptoms of increased intracranial pressure such as syncope, headache, nausea, and vomiting may occur when the CSF flow obstruction occurs and results in hydrocephalus. The incidence of seizures is low (<10%) in LM.
Diencephalic storm or paroxysmal sympathetic outflow has been reported in LM. This may manifest as episodic hyperhidrosis, hypertension, tachypnea, tachycardia, and abnormal posturing. The occurrence of diencephalic storm has been reported in many other neurologic conditions, including hydrocephalus. The differential diagnosis includes seizures, pheochromocytoma, drug withdrawal, thyroid storm, hypertensive crises, and sepsis or anxiety attacks [47].
The clinician should maintain a high index of suspicion in order to diagnose as early as possible the presence of LM. The presence in a cancer patient of neurological signs and symptoms reflecting multifocal neuraxis involvement is highly suggestive of LM. Nevertheless, isolated neurological syndromes may also be seen in patients with LM. The differential diagnosis at initial presentation of LM consists of any underlying cause of chronic meningitis such as tuberculosis, fungal infection, or sarcoidosis. Metabolic and toxic encephalopathies must also be excluded [5, 48].
In known LM, the appearance of new neurological manifestations may reflect progression of LM but other causes must be considered including BM, which can coexist with LM in 30–40% of cases, chemotherapy or radiation related neurological complications, and less commonly paraneoplastic syndromes.
CSF Examination
Cytology
Most cases of LM (90+%) manifest abnormal CSF [49]. Raised opening pressure (>200 mm of H2O), increased leukocytes rate (>4 mm3), elevated CSF protein (>50 mg/dl), and hypoglycorrachia (<60 mg/dl) have been reported in respectively 46, 57, 76, and 54% of LM. Nevertheless, these abnormalities are nonspecific. The presence of malignant cells in the CSF shown by cytological analysis is the mainstay of the diagnosis [49, 50]. The sensitivity of a first lumbar puncture is only 45–55%.
There are a few comparatively simple ways to improve the sensitivity of CSF cytological analysis such as providing a nonhemorrhagic CSF sample and by performing a second CSF assessment; the latter will increase the sensitivity to 80%. The benefit of a third CSF assessment is marginal [9]. To decrease the rate of false negative CSF analysis, samples should be obtained from a site compatible with clinical manifestations or based upon radiologic findings [51]. It has been reported that in the absence of CSF flow obstruction, the rate of discrepancy between lumbar and ventricular simultaneous assessments can reach 30% [51]. The minimal recommended volume of CSF sent for cytology should be of 10.5 ml so as to achieve a sensitivity of 97% (vs. 68% for a volume of 3.5 ml) [52]. The processing of the CSF samples should be urgent since the viability of malignant cells depends on time between sampling and laboratory assessment. Only 50% of the CTC remain viable after 30 min, and only 10% after 90 min [53]. The use of CSF fixation in dedicated tubes permits longer intervals between sampling of CSF and laboratory analysis. Nonetheless, approximately 25–30% of patients with clinical LM and normal neuraxis imaging have persistently negative CSF cytology [22, 49, 54–58].
Tumor Biomarkers
A variety of biomarkers of LM have been suggested to assist in achieving an earlier diagnosis of LM as well as to evaluate effectiveness of treatment [22, 57–66]. These markers include nonspecific biomarkers , such as alpha-glucuronidase, lactate dehydrogenase, beta 2-microglobulin, carcinoembryonic antigen, and others that are organ-specific such as CA 15-3, CA 125, CA 19-9, CA724, AFP, NSE, Cyfra 21-1, and EGFR. Two recent studies have reported that increased CSF levels of CA 15-3 in BC-related LM, and the combination of CEA and Cyfra 21.1 in NSCLC patients with LM may assist in the diagnosis of LM [57, 58]. Proangiogenic molecules (vascular endothelial growth factor [VEGF], uPA, and tPA) have been assessed as potential biomarkers for LM. Most series report elevated levels of CSF VEGF in patients with LM, with very variable sensitivity (51.4–100%) and specificity (71–100%) [60, 62, 67–70]. Combinations of different CSF tumor markers may improve the sensitivity though this requires validation [67]. Profiling CSF proteins (using mass spectrometry and multiplex immunoassays), especially those involved in the metastatic process, may be useful for diagnosis and prognosis assessment [62, 71–73]. At present there is no agreement regarding CSF biomarker cutoff levels nor has there been standardization of these various assays. Due to inconsistencies in laboratory methodology, there is considerable variation in sensitivity and specificity of these assays that produces serious challenges for utilizing biomarkers in the management of LM.
Flow Cytometry
Unlike in hematologic LM, flow cytometry is generally not used for the evaluation of suspected solid tumor-related leptomeningeal disease. A recent study explored the contribution of flow cytometry immunophenotyping (FCI) in the diagnosis of LM in solid tumors [74]. Epithelial cells were identified by expression of the epithelial cell adhesion molecule (EpCAM). Compared with cytology, FCI improved the sensitivity and negative predictive value (79% vs. 50%; 68% vs. 52%, respectively), but had a lower specificity and positive predictive value (84% vs. 100%; 90% vs. 100%).
Circulating Tumor Cells
Techniques used for the detection of circulating tumor cells (CTC) in peripheral blood have been applied to CSF with encouraging preliminary results [75–78]. The rare cell capture technology (RCCT) has also been recently utilized in the diagnosis of LM from solid tumors [76]. The sensitivity of this method was reported as superior to conventional CSF cytology (100% vs. 66.7%), demonstrated excellent specificity (97%), and provided an earlier diagnosis that was subsequently confirmed by delayed CSF cytology.
The Cell Search technology (identification of cell surface tumor associated proteins) has been used in BC and melanoma-related LM [75, 78]. It provides a semi-automated molecular analysis that in the future may improve the sensitivity, reliability, objectivity, and accuracy of detecting CSF tumor cells compared to CSF cytology [75]. These techniques are not yet available in daily practice and further evaluation of these novel technologies is ongoing. At present, the pathologic gold standard in diagnosing LM is still based on the detection of malignant cells in the CSF by cytology [50, 79, 80].
Neuroradiographic Studies
Because LM involves the entire neuraxis, imaging of the entire CNS is required. MRI with gadolinium enhancement is the radiologic technique of choice [81–84]. The standard examination should include axial T1-weighted images without contrast, fluid attenuation inversion recovery (FLAIR) sequences and 3D axial T1-weighted sequences with contrast of the brain. The spine is best evaluated with sagittal T1-weighted sequences with and without contrast and sagittal fat suppression T2-weighted sequences, combined with post-contrast axial T1-weighted images through regions of interest. Contrast enhanced T1-weighted and FLAIR sequences are the most sensitive to detect LM [85, 86].
Any leptomeningeal irritation—whether from hemorrhage, infection, or inflammation—of the SA can result in enhancement on MRI. Lumbar puncture may elicit a meningeal reaction resulting in leptomeningeal enhancement; consequently MRI should be obtained before the lumbar puncture [87]. At LM diagnosis, brain involvement may be present in 40–75%. MRI in LM may demonstrate subarachnoid, ventricular or parenchymal enhancing nodules, focal or diffuse pial enhancement, folia (Fig. 5.1a, b), ependymal (Fig. 5.2a, b), sulcal, or cranial nerve and nerve roots enhancement [88]. SA and parenchymal enhancing nodules (10–35%), diffuse or focal pial enhancement (10–20%) are the most common MRI findings. LM should be considered in the differential diagnosis of hydrocephalus in the cancer patient (Fig. 5.3a, b). Spine involvement is present in 15–25% of patients with LM (Fig. 5.4a, b). BM may be associated with LM in 21–82% [13, 56, 89]. The sensitivity of MRI in LM varies by report from 20 to 91% [10, 13, 22, 54, 56, 88–90]. A normal MRI does not exclude the diagnosis of LM. Nonetheless, in cases with a typical clinical presentation, abnormal MRI alone is adequate to establish the diagnosis of LM [49, 50, 88]. Computed tomography (CT) scan is of limited value in the diagnosis of LM [85]. The sensitivity of CT scan is estimated at 23–38%, and CT scan should be performed only in patients for whom MRI is contraindicated [22, 84].





Fig. 5.1
Gadolinium-enhanced T1 axial (a) and corresponding FLAIR T2 axial (b) brain MRI demonstrating cerebellar folia contrast enhancement and FLAIR hyperintensity in a patient with breast cancer and leptomeningeal carcinomatosis

Fig. 5.2
Ependymal contrast enhancement on gadolinium-enhanced T1 axial MRI (a) and corresponding periventricular hyperintensity on FLAIR MRI (b) in breast cancer with CSF seeding

Fig. 5.3
Gadolinium-enhanced T1 axial MRI (a) and corresponding FLAIR T2 axial MRI (b) demonstrating hydrocephalus and periventricular FLAIR signal hyperintensity in a breast cancer patient with leptomeningeal carcinomatosis

Fig. 5.4
a, b Spinal MRI. Gadolinium-enhanced T1 sagittal MRI. Perimedullary contrast enhancement in breast cancer
CSF Flow Studies
CSF flow and circulation is best assessed by radionuclide cisternography using either 111Indium-diethylenetriamine-pentaacetic acid or 99Tc macro-aggregated albumin [44, 91]. Thirty to seventy percent of LM patients have CSF flow abnormalities, mostly located at the skull base, the spinal canal, and over the cerebral convexities [92–94]. Several series have observed a correlation between the presence of CSF flow abnormalities and a shorter survival when compared to LM patients with normal CSF flow [92–95]. CSF flow can be restored to normal in 30% of patients with spinal blockage and 50% of patients with intracranial blockage by targeting the site of CSF flow obstruction with local radiation therapy (RT) [7].
Increased overall survival, less therapeutic morbidity, and decreased mortality from progressive LM have been reported in patients in whom normal CSF flow has been restored following involved-field RT, when compared to the patients with persistent CSF flow obstruction [91, 94]. Indeed, it is likely that altered CSF flow leads to heterogenous distribution of intra-CSF chemotherapy. This may result in an imbalance in drug exposure in the CSF compartments leading to under-treated areas that favor tumor progression and other regions exposed to excess chemotherapy with consequent neurological and systemic side effects.
Based on these findings, many authors recommend that intra-CSF treatment be preceded by a CSF flow study, and that focal RT be administered on the sites of blockage prior to any treatment [43, 96]. This systematic approach is recommended by the Response Assessment in Neuro-Oncology (RANO) working group on LM in any clinical trial assessing an intra-CSF therapy in LM [97].
Meningeal Biopsy
Staging
In summary, patients with suspected LM should undergo, prior to any anticancer treatment, one or two lumbar punctures to establish the presence of CTC, cranial MR-Gd, spinal MR-Gd and a radioisotope CSF flow study (the later only in instances where intra-CSF chemotherapy is to be administered). If cytology and MR are not contributive, a ventricular or lateral cervical CSF analysis may be required. If CSF cytology remains persistently negative despite compelling clinical and radiological manifestations consistent with LM, it is appropriate to commence LM-based therapy.
Differential Diagnosis
In patients with cancer, the diagnosis of LM is often straightforward. The situation is more challenging in patients without any known cancer, or if causality is uncertain (for example, the primary cancer is either in remission or presumed cured) and with a negative CF cytology. Other causes of subacute and chronic meningitis must then be excluded as discussed above [99]. Eventually, if a systemic evaluation does not identify an extraneural cancer, a primary brain tumor should be considered [100–104].
Evaluation of the Response to Treatment
New neurological manifestations must be distinguished from those secondary to parenchymal disease, from complications of intra-CSF treatment, systemic therapies or RT, from concomitant medications, from neurological or extraneurological concurrent disease, and more rarely from paraneoplastic syndromes [82]. Transient manifestations should not be assumed to be LM-related neurological progression. As mentioned earlier, CSF cytological analysis remains the gold standard for the diagnosis of cancer cells in the CSF despite a low sensitivity of a first CSF examination (45–55%), and the usual need of successive CSF samples to adequately assess cytology. The one-dimensional response evaluation criteria in solid tumors (RECIST) criteria are not adapted for the assessment of LM as the imaging characteristics of LM in general are not measurable at least as defined by current brain tumor response criteria [105].
In order to improve the methodology of future clinical trials, a RANO working group critically reviewed published the literature regarding randomized clinical trials (RCT) and trial design in patients with LM [106]. Six RCT have been published regarding the treatment of LM secondary to solid tumors. As seen in Table 5.4, there were significant differences in the response assessment, such that interpretation of the trial results is challenging. Among these RCT, only a single trial attempted to determine whether intra-CSF chemotherapy was superior to systemic therapy only. Otherwise, this fundamental question has not been prospectively answered in patients with solid cancers and LM. The methodology of the 6 RCT varied considerably regarding pretreatment evaluation, type of treatment, and response to treatment. The RCT all share several common initial assessment features including evaluation of CSF cytology. Positive CSF cytology has been required for participation in all published RCT. Additionally, a neurologic clinical assessment and a predefined performance status were required as inclusion criteria in all RCT. Nevertheless, currently in neuro-oncology there is no standard instrument to assess the neurologic examination and consequently RCT in LM have lacked a rigorous method to determine clinical disease progression [106]. Also poorly defined in the published RCT is the utility of neuroimaging assessment (e.g., how radiographic findings alter treatment and how neuroimaging is used in the response assessment independent of CSF cytology and clinical examination) [106]. The RANO LM working group has developed a series of instruments to address these deficiencies [97] that includes a standardized neurological examination, definitions of CSF cytology and a new radiographic evaluative instrument. The working group recommends that “all patients enrolling in clinical trials undergo CSF analysis (cytology in all cancers; flow cytometry in hematologic cancers), complete contrast-enhanced neuraxis MRI and radioisotope CSF flow studies (in patients treated with intra-CSF therapy only)” [97]. “Response based on CSF cytology is considered when CSF converts from positive to negative and with a second confirmatory determination. CSF cytology is not to be considered in isolation in evaluation of response of patients with solid tumors as patients with persistence of positive cytology may continue on treatment if clinically and radiographically stable or improved” [97]. The committee created a composite score to quantify MRI abnormalities recognizing that most LM lesions are non-measurable. They concluded that “radiographic assessment of LM is subjective, qualitative and graded as stable, progressive or improved. Similar to CSF cytology, radiographic disease progression in isolation, i.e., stable CSF cytology and neurological assessment, would be defined as LM disease progression” [97]. It is hoped that ultimately this work will provide a standardized approach for LM assessment with validated criteria for response to treatment, and defined endpoints for clinical trials.
Table 5.4
Randomized clinical studies in Leptomeningeal metastases
Study | Design | Response | Toxicity |
---|---|---|---|
Hitchins [166] | N = 44 Solid tumors and lymphomas IT MTX versus MTX + Ara-C | IT MTX versus IT MTX + Ara-C: RRa: 61% versus 45% Median survival:a 12 versus 7 wk | IT MTX versus IT MTX + Ara-C: N/V: 36% versus 50% Septicemia, neutropenia: 9% versus 15% Mucositis: 14% versus 10% Pancytopenia: 9% versus 10%. Blocked Ommaya: 17% Intracranial hemorrhage (Ommaya placement): 11% |
Grossman [188] | N = 59 Solid tumors and lymphoma (in 90%) IT MTX versus thiotepa | IT MTX versus IT thiotepa: Neurological improvements: none Median survival: 15.9 versus 14.1 wk | IT MTX versus thiotepa: Serious toxicity (47%) Similar between groups Mucositis and neurological AEDs more common in IT MTX group |
Glantz [182] | N = 28 Solid tumors l-Ara-Ca versus MTX | IVent l-Ara-Ca versus IVent MTX: RRa: 26% versus 20% OSa: 105 versus 78 d TTPa: 58 versus 30 d | l-Ara-Ca versus MTX: Sensory/motor: 4% versus 10% Altered mental status: 5% versus 2% Headache: 4% versus 2% Bacterial meningitis: 10% versus 3% |
Boogerd [193] | N = 35 Breast cancer Systematic therapy and involved-field radiotherapy with versus no IT MTX | Systemic therapy and involved radiotherapy with IT MTX versus no IT MTX: Improved stabilization: 59% versus 67% TTPa: 23 versus 24 wk | Systemic therapy and involved radiotherapy with IT MTX versus no IT MTX: Drug-related AEs: 47% versus 6% |
Shapiro [310] | Solid Tumors: n = 103 l-Ara-Ca versus MTX Lymphoma: n = 24 l-Ara-C versus Ara-C | IVent l-Ara-Ca versus IVent MTX/Ara-C: PFSa: 35 versus 43 d IVent l-Ara-Ca versus IVent MTX: PFSa: 35 versus 37.5 d IVent l-Ara-Ca versus IVent Ara-C: CytRa: 33.3% versus 16.7% | IVent l-Ara-Ca versus IVent MTX/Ara-C: Drug-related AEs: 48% versus 60% Serious AEs: 86% versus 77% |
Survival and Prognostic Factors
The median OS of untreated patients with LM is 4–6 weeks [3, 14, 48, 94, 107–113]. Despite aggressive treatment, LM has a poor prognosis. The survival of patients with combined treatment is usually less than 6 months with a median OS of 2–3 months [3, 10, 13, 14, 22, 48, 54, 56, 89, 90, 109–113]. Table 5.5 illustrates reported survival in patients with LM from the recent literature.
Table 5.5
Median OS in the main cohorts of LM according to the primary type of tumor
Type of the primitive tumor | References | N patients | Median overall survival (Min–Max) |
---|---|---|---|
Breast cancer | Grossman [188] Clamon [311] Boogerd [196] Jayson [312] Chamberlain [48] Jaeckle [184] Boogerd [193] Regierer [313] Rudnicka [56] De Azevedo [54] Clatot [109] Gauthier [22] Lee [55] Kim [314] Lara-Medina [11] Le Rhun [185] Niwinska [125] | 52 22 58 35 32 43 35 27 67 60 24 91 68 30 49 103 149 | 14.1–15.9 weeks 21–150 days 12 weeks 77 days 7.5 months 7 weeks 18.3–30.3 weeks 9 weeks 16 weeks 3.3 months 150 days 4.5 months 4.5 months 8 months 7 weeks 3.8 months 4.2 months |
Melanoma | Chamberlain [94] Harstad [110] Raizer [14] | 16 110 40 | 4 months 10 weeks 4 months |
Lung cancer | Rosen [18] Chamberlain [190] Hammerer [315] Sudo [316] Chuang [317] Morris [13] Park [89] Lee [118] Riess [120] Xu [119] | 60 32 26 37 34 50 125 149 30 108 | 7 weeks 5 months 57 weeks 106 days 5.1 weeks 3 months 4.3 months 14 weeks 6 months 5.3 months |
The goal of LM-directed treatment is to improve or stabilize neurologic status, maintain neurological quality of life, and prolong survival. Nevertheless, deciding which patients to treat remains a challenge. The NCCN CNS guidelines (version 1.2012) have attempted to distinguish between patients reasonably considered for treatment versus those patients in whom supportive care is most appropriate [49, 50, 94, 107, 114, 115] (Table 5.6).
Table 5.6
Risk categories in patients with in leptomeningeal metastases
High-risk group | Low-risk group |
---|---|
KPS < 60% Major neurological deficits Extensive systemic disease without any efficient treatment available Bulky CNS disease LM-related encephalopathy | KPS ≥ 60% No major neurological deficits Minimal systemic disease Manageable with an effective treatment No CSF blockade |
Based on the literature, the histology of the primary cancer is known to be the prominent prognostic factor with regard to OS in LM [50, 116]. Multivariate analysis has confirmed the association between survival and primary tumor type and the better prognosis of BC compared with lung cancer or melanoma-related LM [10, 68, 117]. Breast cancer LM has a relatively good prognosis among all solid tumor-related LM, with a median OS of 3.3–5 months [22, 54–56, 109]. Modest improvement in lung cancer-related LM may in part reflect increasing use of targeted agents such as tyrosine kinase inhibitors (TKI) based on recent reports of patients with lung cancer and LM with reported median survival of 3–6 months [13, 89, 118–120]. LM secondary to melanoma continues to demonstrate the worst prognosis of all solid tumor-related LM with a median OS estimated between 10 weeks and 4 months before the era of the immunotherapy and targeted therapies [107–110].
In addition to tumor histology, multivariate analysis in series including all types of solid tumors confirms the associations between OS and the performance status (PS), the age at LM diagnosis, the presence of a LM-related encephalopathy, time from diagnosis of primary tumor to diagnosis of LC (>67 weeks), the CSF protein level, the treatment modality (administration of systemic therapy), and the presence of a short-term response to treatment [10, 68, 117, 121–124]. In one report, gender was significant in multivariate analysis likely due to an interaction between gender and tumor type [10]. Recently, the quantification of CSF EpCAM+ cells obtained with flow cytometry immunophenotyping (FCI) before any treatment has been reported to be an independent prognostic factor for OS in LM patients with a cutoff value of 8% [74].
In patients with BC and LM, multivariate analysis demonstrates an association between OS and PS as well as PS and treatment (number of prior chemotherapy regimens, receipt of combined treatment modality, coadministration of systemic chemotherapy or intra-CSF chemotherapy) [11, 22, 54, 55, 109, 122, 125]. Histological characteristics (histological grade, hormone receptor status, triple-negative status), the initial CSF protein level, the number of prior chemotherapy regimens, status of systemic disease (i.e., isolated CNS metastases or bone metastases), the presence of an early and/or combined cytological and neurological response to treatment and the initial CSF cyfra 21-1 and protein levels were also identified as significantly associated with prognosis in BC-related LM [11, 22, 54–56, 109, 122–125].
In recent retrospective series of patients with NSCLC and LM, multivariate analysis confirmed that PS, low initial CSF protein level, low initial CSF (white blood cells) WBC count were all significantly associated with a better OS [13, 89, 118]. The treatment modality (systemic therapy and intrathecal chemotherapy) and clinical improvement after intra-CSF chemotherapy were all significant predictors of increased OS [13, 89, 118, 120]. The impact of whole brain radiotherapy (WBRT) in the treatment of LM is unclear [13, 89, 118–120]. In EGFR-mutated NSCLC, EGFR inhibitors have produced durable responses in patients with LM [13, 89, 113, 118, 119, 126–130]. In patients with melanoma, multivariate analysis suggests that a history of a primary melanoma lesion originating on the trunk predicted shorter OS and that intra-CSF chemotherapy predicted longer OS [110]. Nevertheless, unlike prognostic factors present at LM diagnosis and before any treatment, the interpretation of prognostic factors related to treatment and mostly derived from retrospective series remains uncertain. All such series are single-institution retrospective studies in which treated patients are heterogeneous with respect to recognized LM-prognostic features. Because of the retrospective nature of these studies, there were no a priori determinants of treatment such that treatment was defined individually, without apparent standardization making cross-comparisons between treatment groups difficult [131]. Moreover, two series, one in NSCLC and the other in all types of solid tumor LM, report that the placement of a VPS was significantly predictive of improved OS in LM [118, 132]. These observations seem counter-intuitive and are difficult to reconcile.
Treatment
The goals of treatment include palliating neurologic symptoms and whenever possible stabilizing or improving patient neurologic function as well as prolonging survival. Since the prognosis of LM varies noticeably depending upon the primary tumor type and extent of both neurologic and systemic disease, parameters separating poor-risk from good-risk patients are helpful to determine the appropriate therapeutic approach for an individual patient. The poor-risk and good-risk patients categories are illustrated in Table 5.6. LM ideally should be diagnosed early in the disease course before the appearance of fixed and disabling neurological deficits. Early LM-directed treatment may allow maintenance of quality of life and potentially improve survival. A combined treatment approach (i.e., systemic and intra-CSF chemotherapy and site specific radiotherapy) may provide better palliation in patients with LM [133].
Symptomatic Treatment and Supportive Care
Patients with low PS, neurologic deficits interfering with quality of life, encephalopathy due to extensive LM-brain infiltration, or uncontrolled systemic disease with limited therapeutic options have a poor prognosis even with aggressive LM-directed treatment. A palliative approach should be considered in such poor prognosis patients. Regardless, however, supportive care is needed in every patient with LM independent of treatment in order to palliate neurological symptoms and signs associated with LM.
Pain relief is required for headache, back, and radicular pain, and is based on the use of analgesics of increasing efficacy from acetaminophen to opioids. In addition, neuropathic pain often requires use of tricyclic antidepressants (such as amitriptyline or nortriptyline) or antiepileptic drugs (such as gabapentin, pregabalin, carbamazepine, and lamotrigine). Corticosteroids may also improve radicular pain. Focal irradiation of symptomatic sites provides often a good analgesic effect. Seizures are addressed with anticonvulsant drugs (AED) but prophylactic administration of AED is not recommended. Headaches related to edema or increased intracranial pressure can sometimes be managed with steroids, even if the contribution of steroids in the treatment of LM is modest as compared with their efficacy in brain parenchymal metastases. In instances of hydrocephalus secondary to CSF obstruction, steroids in conjunction with whole brain or skull-base radiotherapy may be effective but CSF shunting is often needed [91, 134]. Iterative lumbar punctures when there is no threatening BM may be an alternative method to relieve temporarily headache in patients declining CSF diversion. Depression or fatigue may be managed with serotonin reuptake inhibitors or stimulant medication (modafinil, methylphenidate) as clinically appropriate [45]. Finally a discussion regarding of end of life before institution of LM-directed therapy is recommended in all patients so as to realistically outline the course of disease and palliative treatment goals.
Surgery
The main surgical interventions in LM are ventriculoperitoneal shunting (VPS) for symptomatic hydrocephalus, placement of a ventricular (rarely lumbar) access device (e.g., an Ommaya or Rickham reservoir) to facilitate administration of intra-CSF chemotherapy, and meningeal biopsy. When both a VPS and Ommaya ventricular access device are needed, an on–off valve may be an option but the patient should be able to tolerate having the VPS placed in the off position so as to permit drug installation into the ventricles and time for ventricular transit and distribution into the nonventricular CSF compartments [134–136]. Complications of VPS rarely exceed 10% in recent series [135, 136] but include the potential for peritoneal tumor dissemination, device failure, device malpositioning, device exposure, infection, hemorrhage, and leukoencephalopathy [45, 135, 136].
When a ventricular access device is placed, confirmation post-implantation of correct intraventricular (IVent) placement requires a brain CT or alternatively a radioisotope CSF flow study before intra-CSF drug administration [137–139]. Hemorrhage at the time of device placement occurs in less than 1% of patients. Device infection (4–10% incidence) is due mainly to Staphylococcus epidermidis and is either a complication that occurs in relationship to surgery or results from contamination at the time of device access [133, 134, 138–140]. In instances where the ventricular device becomes infected occasionally, the device may be left in situ and treated with both intravenous and IVent antibiotics [93, 140–144]. Most often, however, device infections requires removal and if indicated, replacement of the reservoir [93, 135, 142]. A rare complication in patients with increased intracranial pressure is retrograde tracking of instilled chemotherapy along the catheter, resulting in subgaleal or intraparenchymal collections of CSF that may become symptomatic and require revision or replacement with a ventriculoperitoneal shunt [5].
Radiation Therapy
Craniospinal axis irradiation (CSI) is the only method of radiotherapy that treats the entire neuraxis and that may be reasonably considered as a single modality of treatment for LM. However, in the majority of adults CSI is rarely considered as most patients have previously had some region of the neuraxis irradiated, and as well have poor bone marrow reserve as a consequence of prior exposure to cytotoxic chemotherapy. Consequently, CSI and treatment-associated toxicities of myelosuppression and enteritis is deemed too toxic for routine use in adults with solid tumor-related LM. The role of alternative methods of CSI such as tomotherapy and proton radiotherapy , which could permit improved precision in radiation dosing and targeted volumes and consequently less hematological toxicity, has not been formally evaluated and may be an option in the future.
The majority of patients with LM receive involved-field radiotherapy to sites of symptomatic disease, bulky disease observed on MRI and to sites of CSF flow block defined by radioisotope ventriculography . Irradiation permits tumor masses not treated by intra-CSF chemotherapy (due to limited diffusion of intra-CSF chemotherapy) to receive palliative radiotherapy [145]. WBRT is most commonly administered at a dose of 30 Gy delivered in 10 fractions over 10 days. While pain relief and stabilization of neurological manifestations are regularly observed, unfortunately significant neurological improvement is exceptional. The failure of neurological improvement is due to demyelination, axonal and neuronal injury, and injury by infiltrating cancer cells, aspects that underscore the need for early treatment of LM [146]. Regardless of MRI findings (e.g., the absence of visible radiographic disease), lumbosacral irradiation is indicated in instances of cauda equine syndrome (low back pain, legs weakness, bladder or bowel dysfunction). Similarly, skull-base RT may be used for cranial neuropathies [8]. RT is also indicated to reestablish normal CSF circulation to improve therapeutic efficacy and reduce side effects of intra-CSF chemotherapy [1–6, 8, 94]. Communicating hydrocephalus is not infrequent in LM and is caused by malignant cells in the subarachnoid space that obstruct normal CSF resorption pathways. In these instances, WBRT or placement of a VPS is often required [147]. Shunting of CSF should be provided in patients with symptomatic or communicating hydrocephalus that does not rapidly respond to WBRT.
Unlike brain metastases, the impact of WBRT on OS is not clearly established in LM, even in radiosensitive cancers such as BC and NSCLC. Contradictory results have been reported that in part reflect the limited survival of patients with LM (<15% survive 1 year) [13, 89, 118–120]. In small case reports WBRT has been reported (as part of a combined modality treatment) to result in prolonged survival (from 13 to 19 months) in patients with melanoma and LM [148–152]. In these case reports, WBRT was either delivered concomitantly or prior to other treatment (intra-CSF or systemic). However, the retrospective nature of the data makes it challenging to determine the role of WBRT in the treatment of LM.
Some authors have posited that WBRT may enhance the efficacy of targeted drug therapy by either by radio sensitization or improving drug penetration into brain/CSF [148, 149]. Improved brain penetration following WBRT has been demonstrated with systemically administered trastuzumab, a monoclonal antibody a low rate of CNS penetration rate [153–155]. In addition to mechanically disrupting the BBB, radiation may hinder the efflux function of multidrug resistance proteins [149]. Indeed, the low CSF concentration of vemurafenib has been related at least partially to the active efflux by P-glycoprotein and breast cancer resistance protein [156].
Side effects of involved-field RT alone are uncommon and manifest mainly as radiation-associated fatigue. However, myelosuppression, mucositis, esophagitis, and leukoencephalopathy have been reported with more extensive radiation fields. Leukoencephalopathy (asymptomatic more often than symptomatic) may be a delayed consequence of WBRT and may be enhanced with coadministered methotrexate (MTX) (either systemic or intra-CSF). Ongoing clinical trials evaluating the safety of concomitant WBRT and intra-CSF liposomal cytarabine will determine if this is a common problem with chemoradiation or unique to MTX when combined with radiotherapy.
Chemotherapy
Chemotherapy is the only treatment aside from CSI to treat simultaneously the entire neuraxis. Chemotherapy may be administered systemically or instilled directly into the CSF. Intra-CSF administration is either intrathecal (IT) by the lumbar route or intraventricular by way of a ventricular access device (IVent).
Intra-CSF Chemotherapy
The benefit of intra-CSF IT or IVent chemotherapy in LM over systemic treatment alone has never been proven in RCT (Table 5.4) [106]. Nonetheless, recent retrospective studies suggest that intra-CSF chemotherapy may be useful in NSCLC patients. This population is rarely treated with intra-CSF chemotherapy treatment because of historic poor prognosis [13, 89]. Park reported on 48 patients with NSCLC-related LM who received intra-CSF chemotherapy with a cytological response rate of 52% [89]. The median survival was 5.5 months in cytological responders and 1.4 months in nonresponders [89].
In a series of 149 patients with NSCLC (mainly adenocarcinoma) and LM, Lee reported a significantly favorable impact of intra-CSF chemotherapy in multivariate analysis [118]. Similarly, the favorable impact of intra-CSF chemotherapy when combined with systemic therapy has been retrospectively reported in BC patients with LM [122]. In melanoma patients, intra-CSF treatment has been reported as a predictive factor of longer survival with occasional long-duration survivors in a retrospective study [110, 150, 151, 157]. However, due to the limited number of patients, heterogeneous intra-CSF treatment and retrospective nature, these results should be interpreted cautiously.
Most anticancer drugs, when given by a systemic route in patients with intact blood–brain and blood–CSF barriers, display poor penetration into the CNS that translates into a CSF exposure less than 5% of the plasma concentration. The blood–CSF barrier in LM undergoes a partial disruption only that varies from one area to another such that with few exceptions (e.g., high-dose methotrexate (MTX) discussed later for breast cancer-associated LM) systemic chemotherapy is rarely a primary treatment of LM.
The goal of intra-CSF chemotherapy is to bypass the blood–CSF barrier, and maximize CSF exposure while decreasing systemic toxicity. Thus, since the distribution volume of CSF is less than that of the plasma (140 vs. 3500 ml), a higher concentration of the active agent can be reached using a lower dose [158]. Moreover, most cytotoxic agents’ half-life is longer in the CSF than in plasma, allowing a prolonged CSF exposure that is particularly useful for cell-cycle-specific drugs such as MTX and ara-C. The majority of affected regions in LM is only a few cells in thickness and since intra-CSF chemotherapy can diffuse a relatively short distance (1–2 mm), small volume disease and cells suspended in CSF can be appropriately treated [159, 160]. However, intra-CSF chemotherapy cannot reliably treat radiographically bulky leptomeningeal disease because of limited diffusion into tumor lesions >2 mm in diameter, into the Virchow–Robin spaces, and along nerve root sleeves.
Lumbar Intrathecal or IntraVentricular Route of Administration
Since patient position has an impact on ventricular drug levels after intralumbar administration, patients should remain flat for at least 1 h following injection [161]. On only rare occasion is IT drug administration delivered through a lumbar catheter connected to a subcutaneous reservoir, as such devices frequently fail with repeated use due to the high protein content found in the lumbar cistern.
IVent administration of intra-CSF drug via an Ommaya or Rickham reservoir offers some advantages compared with IT therapy [52]. The procedure is painless for the patient and more time-efficient for the clinician. The drug is also safely administered at the correct place without the 10% risk of epidural or sudural injection observed with IT injections. It is also safe to administer IVent injection in instances of thrombopenia, thus avoiding the risk of epidural or subdural hematoma after lumbar puncture [162]. A better pharmacokinetic profile is also achieved with IVent administration, with improved and more uniform drug distribution in the entire CSF compartment [163–165]. IVent CSF drug concentration following IT injection is only 10% of that achieved after an equivalent IVent dose. Another advantage is that more frequent administrations of smaller doses of drug are an option to achieve lower peak drug concentrations and therefore limit total cumulative dose and neurological side effects. A survival benefit was suggested for IVent compared with IT chemotherapy in one randomized clinical trial [166]. IVent and IT administrations were compared in a subset analysis of a RCT comparing liposomal ara-C with MTX in 100 patients with LM [167]. Overall, intra-CSF chemotherapy was given by IVent and IT route in 72 and 28% of cases, respectively. For patients given liposomal ara-C, there was no statistically significant difference in progression-free survival (PFS) according to the route of administration. For those given MTX, the IVent route appeared superior (PFS 19 vs. 43 days) suggesting that the site of administration affects survival and is dependent upon the CSF half-life of the chemotherapy.
Techniques of Intra–CSF Administration
It is critical to avoid any variation in CSF volume in LM patients, who often are on the edge of the CSF ventricular “pressure-volume” compliance curve. If the total CSF volume is increased, severe intracranial hypertension can occur. Thus equivalent volume of CSF should be removed (so-called isovolumetric withdrawal) prior to chemotherapy administration. During the withdrawal of a large volume of CSF from the ventricles, a transient retro-orbital or frontal headache may result. The headache is often improved with administration of intra-CSF chemotherapy if given in 5–10 ml volume. No prospective trials in adults with LM have proven any benefit to concomitant use of intra-CSF glucocorticoids (hydrocortisone) in combination with intra-CSF chemotherapy.
Drugs Available for Intra–CSF Treatment
Currently, MTX, liposomal ara-C, and less often thiotepa are used in daily practice (Table 5.7). Unfortunately, these agents are not generally considered active systemically against most common solid cancers associated with LM, particularly melanoma and lung cancer. New agents including monoclonal antibodies are currently being investigated in clinical trials and are discussed below.
Table 5.7
Intrathecal chemotherapy regimens in LM
Drugs | Induction regimens | Consolidation regimen | Maintenance regimen | |||
---|---|---|---|---|---|---|
Bolus regimen | C × T regimen | Bolus regimen | C × T regimen | Bolus regimen | C × T regimen | |
Methotrexate | 10–15 mg twice weekly (Total 4 weeks) | 2 mg/day for 5 days every other week (Total 8 weeks) | 10–15 mg once weekly (total 4 weeks) | 2 mg/day for 5 days every other week (total 4 weeks) | 10–15 mg once a month | 2 mg/day for 5 days once a month |
Ara-C | 25–100 mg 2 or 3 times weekly (Total 4 weeks) | 25 mg/day for 3 days weekly (Total 4 weeks) | 25–100 mg once weekly (Total 4 weeks) | 25 mg/day for 3 days every other week (Total 4 weeks) | 25–100 mg once a month | 25 mg/day for 3 days once a month |
Liposomal cytarabine | 50 mg every 2 weeks (Total 8 weeks) | 50 mg every 4 weeks (Total 24 weeks) | ||||
Thiotepa | 10 mg 2 or 3 times weekly (Total 4 weeks) | 10 mg/day for 3 days weekly (total 4 weeks) | 10 mg once weekly (Total 4 weeks) | 10 mg/day for 3 days every other week (Total 4 weeks) | 10 mg once a month | 10 mg/day for 3 days once a month |
Methotrexate
Therapeutic CSF concentrations of 1 μM/mL or more are seen 48–72 h after a 12 mg IT dose of MTX in adults and in children aged older than 2 years [3, 165, 168–170]. Usually, MTX is administered on a twice-weekly schedule for 1 month; then the frequency of injections is reduced over a total period of 3–6 months. The optimal duration of treatment is not known, and a prolonged treatment may benefit some patients. Alternative schedules have been reported such as 2 mg of IVent MTX for 5 consecutive days every 2 weeks [169, 171, 172]. A dose-intense regimen of MTX (15 mg/day, 5/7 days, 1 week on 1 week off) has been reported in a retrospective study of BC patients with a median survival of 4.5–5 months [22, 109, 173]. Conversion from positive to negative CSF cytology with intra-CSF MTX occurs in 20–61% of patients with LM [174–176]. The clinical efficacy of different schedules of MTX in retrospective BC LM studies is illustrated in Table 5.8. This table as well reflects coadministered CNS-directed RT given as part of the LM treatment regimen, which makes the interpretation of the impact of one intra-CSF MTX regimen versus another challenging [174–177]. Achievement of a cytological response in the first month of IT MTX treatment may be predictive of increase survival (6 vs. 2 months) [177]. Regardless, OS is within a similar range irrespective of the intra-CSF MTX schedule. Considering the short survival of patients with LM and the difficulty differentiating with certainty the respective impact of radiation and intra-CSF chemotherapy, quality of life should be the priority when considering treatment of LM.
Table 5.8
Intra-CSF treatments in breast cancer
Agent/Reference | N | Other treatments | Response | Median OS (months) |
---|---|---|---|---|
Standard MTX Rudnicka [56] | 67 | Systemic: 61% RT: 64% | Clinical: NS Cyt: NS MRI: NS Overall: 76% | 4 |
Standard MTX De Azevedo [54] | 60 | Systemic: 43% RT: 36.7% | Clinical: NS Cyt: NS MRI: NS | 3.3 |
Intensified-MTX Clatot [109] | 24 | Systemic: 46% RT: 46% | Clinical: 96% Cyt: 46% MRI: NS | 5 |
Intensified-MTX Gauthier [22] | 80 | Systemic: 78% RT: 29% | Clinical: 73% Cyt: 20% MRI: NS | 4.5 |
l-AraC Le Rhun [185] | 103 | Systemic: 58.2% RT: 13.5% | Clinical: 56.8% Cyt: 30.6% MRI: 62.5% | 3.8 |
MTX is eliminated from the CSF by CSF/venous resorption and subsequent delivery into the systemic circulation. Consequently, conditions that impact CSF resorption increase intra-CSF MTX-related neurotoxicity. Similarly, renal impairment decreases the excretion of MTX and may aggravated systemic MTX toxicity. The presence of pleural or peritoneal effusions (creating a “third space effect”) accentuates accumulation of MTX, which may increase systemic MTX side effects such as myelosuppression or mucositis. The concomitant use of drugs that displaces MTX from albumin, such as aspirin, phenytoin, sulfonamides, and tetracycline, may also amplify MTX toxicity. Neurologic complications of intra-CSF MTX include aseptic meningitis, acute encephalopathy, transverse myelopathy, and delayed leukoencephalopathy. Folinic acid (leucovorin) has been suggested to mitigate systemic MTX toxicity and is often prescribed orally 10 mg every 6 h for 1–2 days after each intra-CSF MTX administration. Leucovorin does not cross the blood–brain barrier in sufficient amounts to interfere with the efficacy of intra-CSF MTX. Significant or even lethal complications may occur after an involuntary overdose of intra-CSF MTX. Recommendations have been issued for such clinical situations including drainage of CSF via lumbar puncture, ventriculostomy with ventriculo-lumbar perfusion, systemic steroids, and systemic leucovorin, and the use of carboxypeptidase-G2 (CPDG2) as an antidote are proposed. CSF MTX concentrations have been reduced by a 400-fold within 5 min of this antidote use according to some pharmacokinetic studies [178].
Cytosine Arabinoside (Cytarabine)
Cytarabine (ara-C) is initially administered at a dosage of 25–100 mg twice weekly. The 4-week induction period is followed by 4 weeks of consolidation and subsequent maintenance. The CSF half-life of ara-C is much longer than in serum because cytidine deaminase, the main catabolic enzyme of ara-C, is present at very low level in the CSF. The rapid deamination that occurs in the systemic circulation leads to a good systemic tolerance profile. A concentration time regimen (i.e., low dose intra-CSF chemotherapy given for consecutive days) has also been reported [179]. Liposomal ara-C, a depot encapsulated formulation (DepoCyt) is a useful intra-CSF agent in patients with LM secondary to solid tumors as conventional ara-C is relatively ineffective due primarily to the short half-life of ara-C (approximately 3.4 h). Intra-CSF administration of the conventional formulation of ara-C results in complete clearance of the drug from the CSF within 1 or 2 days [180, 181]. In contrast, liposomal ara-C with a half-life of 140 h provides a therapeutic ara-C concentration in the CSF for up to 10–12 days. Due to the long half-life of liposomal ara-C, intra-CSF drug administration may be once every 2 weeks. At present, liposomal ara-C is approved only for lymphomatous meningitis but is often used off-label for solid tumor-related LM.
In a RCT with solid tumor patients with LM, assessing intra-CSF liposomal ara-C versus MTX, the median time to neurologic progression was improved (58 vs. 30 days) with a small increase in toxicity but fewer visits to the hospital (75% reduction) with liposomal ara-C [182, 183]. There was no significant difference between arms for median survival (105 vs. 78 days) [182].
The liposomal ara-C regimen showed a better quality-adjusted survival regardless of the quality of life assessment based on time with toxicity and time following disease progression (range, 44–79 days). In BC patients, liposomal ara-C has shown similar cytological response rates (26–30%) and median survival (3–4.6 months) compared with other intra-CSF drugs in nonrandomized series [90, 124, 125, 184, 185] (Table 5.8). Nevertheless, the acceptable tolerance profile of liposomal ara-C and its convenient schedule of administration present some advantages compared to other intra-CSF regimens.
Liposomal ara-C has been used in melanoma-associated LM, in which occasional sustained responses (14–24 months) have been observed [148, 150, 157]. The role of intra-CSF liposomal ara-C in durable response achievement and survival in these case reports is difficult to determine as the majority of patients received systemic therapy, involved-field radiotherapy as well as liposomal ara-C. Liposomal ara-C is mainly complicated by arachnoiditis (i.e., a transient sterile chemical meningitis), but the use of oral dexamethasone (4 mg twice daily during 5 days, onset on the day of liposomal ara-C injection) decreases the occurrence of this side effect [186, 187].
In a retrospective series of 120 patients with LM and treated with liposomal ara-C, neurotoxicity included bacterial meningitis (IVent:IT treatment; 3.75%:0%); chemical meningitis (17.5%:15%); communicating hydrocephalus (3.75%:5%); conus medullaris/cauda equina syndrome (5%:5%); decreased visual acuity (5%:2.5%); encephalopathy (5%:5%); leukoencephalopathy (7.5:2.5%); myelopathy (2.5%:2.5%); radiculopathy (1.25%:5%); and seizures (1.25%:2.5%) [187]. The toxicity profile was similar in ventricular and lumbar routes. Most side effects were reversible (57% vs. 43%). Hospitalization was needed in 32.2%. The tolerance profile of liposomal ara-C was generally good; however, 12.5% of patients developed serious and permanent neurological side effects that negatively impacted quality of life [187].
Clinicians are advised to inform pathologists when patients are under liposomal ara-C since liposomal particles may be confused microscopically with white blood cells. A randomized Phase III trial is currently ongoing in France to evaluate intra-CSF liposomal ara-C (vs. no intra-CSF therapy) in BC-related LM.
Thiotepa
Thiotepa , an alkylating agent and therefore cell-cycle-nonspecific, has the shortest half-life (approximately 20 min) of all drugs directly administered in the CSF. CSF clearance is achieved within 4 h. It is usually used as a second-line agent for BC patients, when MTX is ineffective or not tolerated. Thiotepa, unlike other intra-CSF administered drugs, rapidly crosses brain capillaries and consequently may result in meaningful systemic serum levels and associated myelosuppression. Because the half-life is short and the transcapillary movement is rapid, it has been argued that there is no pharmacological advantage to intra-CSF thiotepa. Nonetheless, a RCT has compared intra-CSF thiotepa with intra-CSF MTX in LM patients. Similar median survivals were observed: 14 weeks with intra-CSF thiotepa versus 16 weeks with intra-CSF MTX. The CSF cytological rate was 30%. Less neurotoxicity was reported with thiotepa [188]. Twenty-four BC patients with LM were treated with second-line intra-CSF thiotepa following failure of intra-CSF liposomal ara-C in a retrospective study [189]. Systemic chemotherapy was also coadministered to nine patients. The median PFS and OS with thiotepa was 3.1 months (range 3 days-2 years) and 4.0 months (range 6 days–2.5 years), respectively. The median OS from LM diagnosis was 9.5 months (range 1.3 months–2.7 years). Minimal treatment-related toxicity was observed.
Combination (Multi-agent) Intra-CSF Chemotherapy with Systemic Treatment
Multidrug intra-CSF regimens in LM patients with solid tumor are no more effective than single-drug regimens; moreover, combination therapy is more toxic and associated with a worse tolerance profile [166, 190]. A single RCT compared intra-CSF MTX with intra-CSF MTX plus ara-C plus hydrocortisone in 55 patients [166]. There was a higher rate of cytological response (38% vs. 14%) and a longer median survival (19 vs. 10 weeks) with the multidrug regimen, but these differences did not reach statistical significance and a selection bias (lower risk patients receiving multidrug therapy) cannot be ruled out [191]. In a recent retrospective cohort, the combination of IT MTX and liposomal cytarabine was assessed in 30 LM patients with different types of cancers [192]. Cytologic clearance was achieved in 33% and median OS was 7.5 months in patients with solid tumor-related LM. Considering the retrospective nature of the study, the small number of patients and the heterogeneity of tumor types, interpretation of this study is challenging. Another small randomized study addressed the question of the potential superiority of a combination of systemic and intra-CSF chemotherapy versus systemic treatment alone in LM from BC and failed to show a survival advantage for the intra-CSF chemotherapy treated cohort [193].
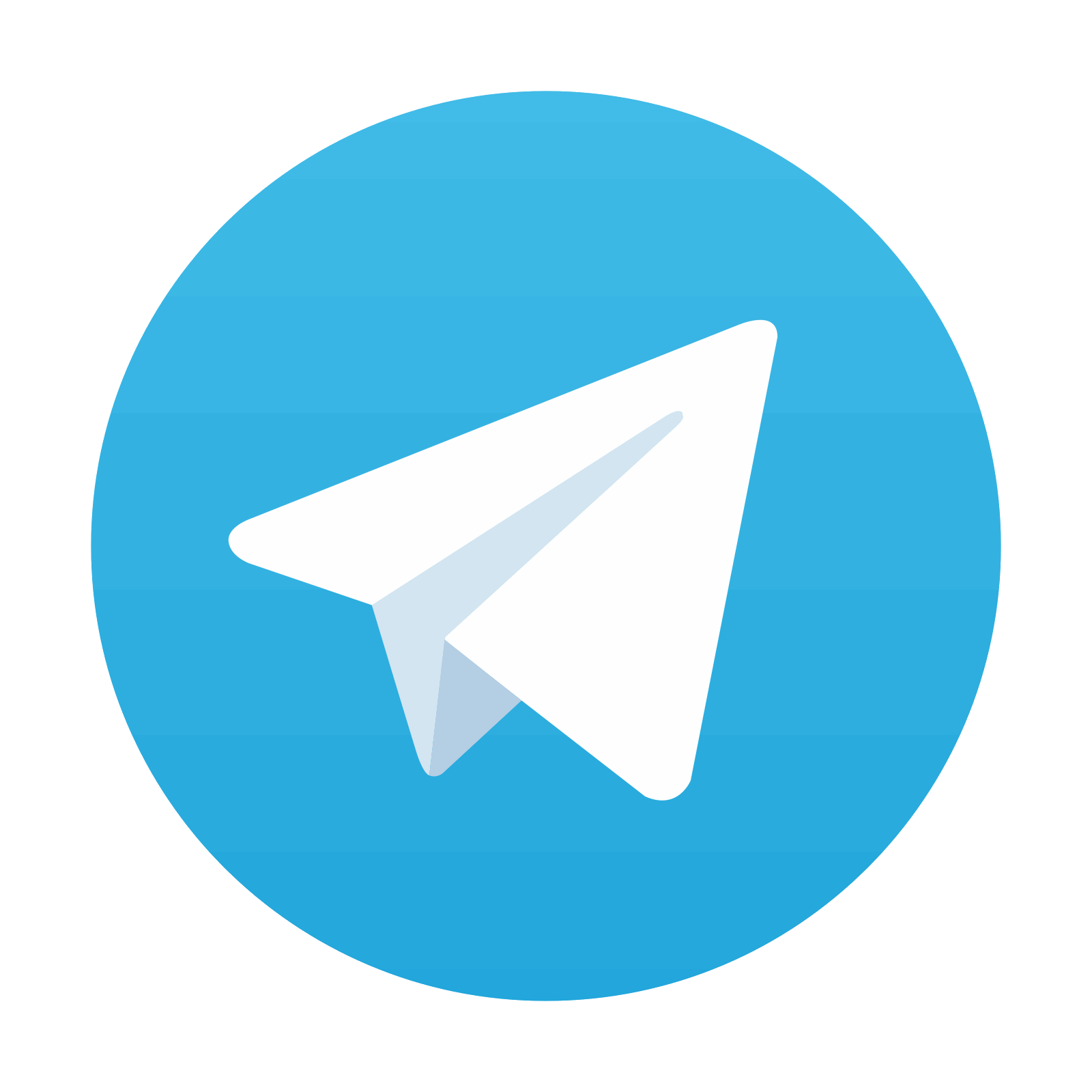
Stay updated, free articles. Join our Telegram channel

Full access? Get Clinical Tree
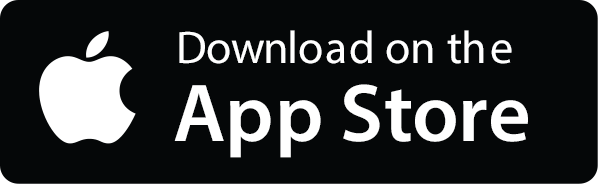
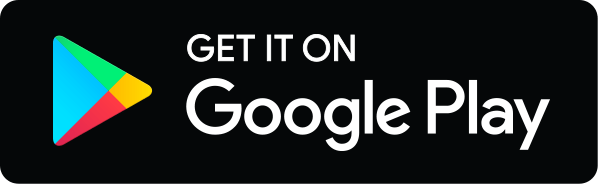