Abstract
The major unifying clinical manifestations of disorders of the neonatal motor system are hypotonia and weakness, which do not necessarily occur with similar severity. In this chapter, all disorders, except those related to the involvement of muscle, are reviewed, principally in terms of clinical features, results of pertinent laboratory studies, pathological features, pathogenesis and etiology, and management.
Keywords
spinal muscular atrophy, infantile botulism, familial dysautonomia, myasthenia gravis, Prader-Willi syndrome, congenital neuropathies, Pompe disease, congenital myasthenic syndromes
An effective means of attaining an understanding of the major disorders of the neonatal motor system is to organize the approach to these disorders on the basis of the major affected anatomical site within the motor system. Thus in this chapter and in Chapter 33 , we review disorders of the neonatal motor system according to the following specific anatomical levels: levels above the lower motor neuron and at the lower motor neuron, the peripheral (and cranial) nerve, the neuromuscular junction, and, finally, the muscle.
The major unifying clinical manifestations of the disorders are hypotonia and weakness, not necessarily occurring with similar severity, as discussed later. In this chapter, all disorders, except those related to the involvement of muscle (see Chapter 33 ), are reviewed, principally in terms of clinical features, results of pertinent laboratory studies, pathological features, pathogenesis and etiology, and management.
Levels Above the Lower Motor Neuron
Disorders leading to hypotonia and weakness that are secondary to the involvement of anatomical levels above the lower motor neuron are summarized in Box 32.1 . These disorders are best discussed here as a group, because most are reviewed in detail in other sections of this book. Although the group is rather diverse, three features are generally useful in establishing the locus of the hypotonia at an anatomical level above the lower motor neuron. First, in these so-called central disorders, hypotonia is usually more severe than weakness, and indeed some affected infants, although floppy , exhibit strong movements when stimulated. Second, tendon reflexes are usually preserved, although it is unusual to observe the hallmark of central hypotonia, as seen after the first weeks and months of life: hyperactive tendon reflexes. Thus, as with weakness, hypotonia is more marked than is involvement of the tendon reflexes. Third, other signs of central involvement are frequently present; particular note should be made of seizures.
Congenital (Nonprogressive) Encephalopathies
Hypoxia-ischemia a
a The two most common causes.
Intracranial hemorrhage
Intracranial infection
Metabolic
Multiple (see text)
Endocrine
Hypothyroid
Trauma
Developmental disturbance
Cerebral a (e.g., Prader-Willi syndrome, neuronal migration disorders)
Cerebellar
Degenerative (Progressive) Encephalopathies
See Chapter 29
Spinal Cord Disorders
Trauma
Developmental
Congenital Encephalopathies
For the purposes of this section, congenital encephalopathies are defined as those with onset before or during the perinatal period and affecting principally cerebrum, brain stem, or cerebellum (see Box 32.1 ). In contrast to degenerative encephalopathies, these disorders are not progressive, although worsening may occur if infectious, metabolic, or endocrine disturbance is not corrected.
Hypoxia-Ischemia
Hypoxic-ischemic encephalopathy is by far the most common cause of hypotonia in the newborn period. Other features of this disorder are described in Chapters 16 and 20 . In patients with minor degrees of this encephalopathy, hypotonia may be the principal neurological abnormality.
Intracranial Hemorrhage and Infection
Intracranial hemorrhage (see Chapter 22 , Chapter 23 , Chapter 24 ) and intracranial infection (bacterial and nonbacterial; see Chapters 34 and 35 ) are relatively uncommon causes of hypotonia in the absence of other features that distinguish these disorders.
Metabolic Disorders
The metabolic disturbances that can result in hypotonia in the newborn period are extremely diverse. Abnormal increases or decreases in electrolyte levels, acidemia, hypoglycemia, increases in divalent cation levels, severe hyperbilirubinemia, aminoacidopathies (including syndromes with hyperammonemia), organic acid disturbances, sepsis, and intoxication of the fetus by administration (usually intrapartum) of analgesics, sedatives, or anesthetics to the mother are the most common of these metabolic disturbances (see Chapter 25 , Chapter 26 , Chapter 27 , Chapter 28 ).
Other rarer metabolic disorders should also be considered. An important category encompasses disturbances in central neurotransmission, the most common of which is aromatic l-amino acid decarboxylase deficiency (see Chapter 29 ). Neonatal onset occurs in more than 50% of cases, and hypotonia and feeding difficulties are prominent early findings. Diagnosis is suggested by the finding of reduced catecholamine metabolites in cerebrospinal fluid (CSF), and it is established by the demonstration of markedly reduced plasma aromatic l-amino acid decarboxylase activity. Subsequent findings include hypokinesia, oculogyric crises, movement disorders, and autonomic disturbances.
Endocrine Disorders
Hypothyroidism is an important endocrine disorder that may produce neonatal hypotonia. A large tongue, temperature instability, feeding problems, constipation, hoarse cry, dry mottled skin, prolonged jaundice, and delayed skeletal maturation should suggest hypothyroidism. Neonatal screening of filter paper blood samples has proved superior to clinical recognition and is of considerable value in early detection of hypothyroidism. It is critical to identify and to treat hypothyroidism promptly because of the deleterious effect of the thyroid deficiency on brain development (see Chapter 5 , Chapter 6 , Chapter 7 , Chapter 8 ).
Trauma
Trauma may result in hemorrhagic and nonhemorrhagic lesions of brain, which are uncommon causes of hypotonia, particularly in the absence of other features that dominate the clinical syndrome. Details are provided in Chapter 22 , Chapter 23 , Chapter 36 .
Developmental Disturbance
Aberrations of brain development are relatively frequent findings among the neonatal causes of hypotonia referable to anatomical levels above the lower motor neuron. Cerebrum and cerebellum are the principal sites of involvement in these cases. Developmental disturbances of cerebrum, which may result in striking hypotonia, are reviewed in Chapter 6 , Chapter 7 , Chapter 8 . Disturbances of neuronal migration are the most prominent cerebral causes. Important examples of cerebral hypotonia are the cerebrohepatorenal syndrome of Zellweger, the oculocerebrorenal syndrome of Lowe, and Prader-Willi syndrome (PWS). The cerebrohepatorenal syndrome of Zellweger is discussed in Chapter 6 , along with disorders of neuronal migration.
The oculocerebrorenal syndrome of Lowe is an X-linked recessive disorder, characterized by ocular abnormalities (cataracts and congenital glaucoma), marked hypotonia, cryptorchidism, and renal abnormalities (proteinuria and generalized aminoaciduria). Subsequent development is markedly retarded. The cerebral abnormality has not been defined clearly.
PWS is characterized by striking neonatal hypotonia, accompanied by diminished deep tendon reflexes, poor feeding, weak cry, and often a history of fetal inactivity. Careful attention to the clinical and other features described in Box 32.2 allows diagnosis in the neonatal period before development of the complete syndrome of hyperphagia, morbid obesity, short stature, and cognitive impairment. The intelligence quotient is less than 70 in 85% of cases, most commonly (75%) in the 40 to 69 range, and is always less than 84. Normal neuromuscular studies such as muscle biopsy and the associated later clinical features (e.g., cognitive impairment) support the conclusion that the hypotonia is on a central basis. The limited neuropathological studies thus far conducted indicate frequent abnormalities of gyral development, hypoplasia of corpus callosum, and minor cerebral, brain stem, and cerebellar migrational anomalies. A careful magnetic resonance imaging (MRI) study also showed gyral anomalies reminiscent of polymicrogyria. Two of the proteins deficient in PWS (i.e., NECDIN and MAGEL2) are critically involved in neuronal differentiation and axonal outgrowth. PWS results from three main molecular mechanisms: paternal deletion, maternal uniparental disomy (UPD) 15, and imprinting defect (ID). In addition to NECDIN and MAGEL2, the PWS critical region on chromosome 15 also includes another three paternal-only origin expressed genes that encode MKRN3 and SNURF-SNRPN proteins. The adjacent UBE3A and ATP10A genes are expressed only in the maternally derived chromosome and are involved in the pathogenesis of Angelman syndrome (AS). The diagnosis is established by detection of the chromosome 15 deletion by fluorescent in situ hybridization (FISH) or by chromosomal microarray (CMA) analysis in the 65% to 75% of patients so affected. The approximately 20% to 30% of patients with UPD have normal deletion studies but an abnormal DNA methylation test. The risk of recurrence in large interstitial 5 to 6 Mb 15q11.2–q13 deletion and pure maternal UPD (without predisposing parental translocation) is less than 1%. The 2% to 3% of cases associated with an ID are detected by DNA methylation studies; recurrence risk in this small group could be as high as 50% if the father also has an imprinting center (IC) deletion. ID without IC deletion carries less than 1% recurrence risk. Advanced DNA methylation techniques such as MS-MLPA (methylation-specific multiplex ligation-dependent probe amplification) can detect deletions, UPD, and ID in more than 99% of PWS patients; this technique can distinguish between deletion and UPD but will not distinguish UPD from ID. Various other probable disturbances of cerebral development (e.g., eponymic syndromes and chromosomal aberrations), some alluded to in Chapter 1 , Chapter 2 , Chapter 3 , Chapter 4 , Chapter 5 , Chapter 6 , Chapter 7 , Chapter 8 , may cause neonatal hypotonia, but more distinguishing features usually dominate the clinical syndrome.
Hypotonia with diminished tendon reflexes
Poor feeding with poor weight gain
Weak cry: often squeaky , not sustained
Craniofacial characteristics
Dolichocephaly, narrow bifrontal diameter
Almond-shaped eyes
Small mouth with thin upper lip and downturned corners of mouth
Hypogonadism (male: undescended testes; scrotal hypoplasia; female: absence or severe hypoplasia of labia minora and/or clitoris)
History of fetal inactivity
Normal neuromuscular studies
Neuropathological and molecular genetic studies suggesting disturbed neuronal and axonal development
Chromosomal disturbance: deletion of the proximal long arm of chromosome 15 (15q11–q13 region) in 65%–75%; uniparental (maternal) disomy in 20%–30%; imprinting defect in 2%–3%.
Developmental disturbance of cerebellum may lead to neonatal hypotonia (see Chapter 4 ). In one carefully studied series of seven such cases, neonatal hypotonia, occasionally severe, occurred without weakness. Jerky eye movements were prominent, and truncal titubation was apparent within a few weeks. Ataxia and intention tremor appeared later in the first year. Computed tomography scans or pneumoencephalograms demonstrated a strikingly enlarged cisterna magna with symmetrical hypoplasia of the cerebellum. The combination of hypotonia, jerky eye movements, and radiographic findings was diagnostic. Pathological study showed nearly complete absence of internal granule cells, with relatively preserved numbers of Purkinje cells. I (JJV) have seen several similar cases and have been impressed by the hypotonia present in early infancy with Dandy-Walker malformation and Joubert syndrome and related disorders, all disorders of cerebellar development (see Chapter 4 ).
Degenerative Encephalopathies
Most degenerative disorders of infancy manifest after the first weeks of life. However, many of these disorders may cause hypotonia in the newborn period (see Chapter 29 ).
Spinal Cord Disorders
Disorders of the spinal cord are frequently overlooked causes of hypotonia and weakness in newborn (see Box 32.1 ). Traumatic injury is a relatively common cause, and the traumatic event may go undetected in the absence of a careful history (see Chapter 36 ). Developmental disorders (e.g., dysraphic states) are associated usually with signs restricted to lower or, less commonly, upper extremities and are recognized more readily (see Chapter 1 ).
Level of the Lower Motor Neuron
Disorders affecting the lower motor neuron are the most frequent causes of severe hypotonia and weakness in the neonatal period. The major disorders to be distinguished are listed in Box 32.3 . Of these, type 1 spinal muscular atrophy (SMA) or Werdnig-Hoffmann disease is the most common and most important.
Spinal muscular atrophy type 1 (Werdnig-Hoffmann disease; also type 0 or type 1A)
Spinal muscular atrophy variants (anterior horn cell disorders not linked to chromosome 5q, non-5q spinal muscular atrophies)
Neurogenic arthrogryposis multiplex congenita
Glycogen storage disease type II (Pompe disease)
Hypoxic-ischemic injury
Neonatal poliomyelitis (other enteroviruses?)
Spinal Muscular Atrophy Type 1 (Werdnig-Hoffmann Disease)
Type 1 SMA or Werdnig-Hoffmann disease refers to the severe, infantile, hereditary form of anterior horn cell disease. This disorder is autosomal recessively inherited. The earliest descriptions of hereditary degeneration of anterior horn cell with onset in infancy were by Werdnig in Austria and Hoffmann in Germany from 1891 to 1900. Although the original cases described by Werdnig and Hoffmann did not have their onset in the first weeks of life, the severe, early onset form of SMA type 1 is often referred to as Werdnig-Hoffmann disease. This severe form of SMA (i.e., type 1) is defined by onset before 6 months of age, failure to develop the ability to sit unsupported, and death usually by less than 2 years of age (see later discussion). (This definition contrasts with that for SMA type 2, which is defined as onset less than 18 months of age, ability to sit unsupported, failure to develop the ability to walk, and death after 2 years of age, and with that for SMA type 3, which is defined as onset after 18 months of age, ability to stand and walk, and death in adulthood.)
The recognition of several very severe cases of SMA with clear prenatal onset, multiple joint contractures, ventilatory compromise at birth, early deficits of facial movement, bone fractures, and death by 3 months led to the recognition of an additional type, termed type 0 or type 1A. Although these cases occur rarely, it is critical to recognize that the diagnosis of SMA is not excluded by the findings of overt arthrogryposis, respiratory failure at birth, or bone fractures. SMA type 1 has been further divided into type 1B with onset of symptoms before 3 months of age and type 1C with onset between 3 and 6 months of age.
Pathogenesis and Etiology
The acute, early-onset, type 1 form of SMA (Werdnig-Hoffmann disease) as well as the rare, very severe type 0 form and the later-onset, chronic forms (types 2 and 3) are all related to a genetic defect that involves the q13 region of chromosome 5. The SMA region consists of a large (500 kb) inverted duplication containing two copies of the gene deleted (or mutated) in SMA (i.e., the survival motor neuron [SMN] gene; Fig. 32.1 ). Thus on each chromosome 5 are two copies of SMN: telomeric (SMN1) and centromeric (SMN2) copies. The deletions involve the telomeric copy. Homozygous deletions involving exon 7 of the SMN1 gene occur in approximately 95% of cases of SMA, with the remaining cases harboring a deletion in one allele and a heterozygous point mutation in the other allele (compound heterozygotes; Table 32.1 ). The nearly identical SMN2 gene, which contains a single nucleotide change in exon 7 (a C-to-T transition) that profoundly influences splicing, produces primarily 90% to 95% of a truncated protein lacking exon 7 and having a short half-life and only approximately 5% to 10% of the normal full-length protein. Because of the genomic instability of this duplicated region of chromosome 5, SMN2 copy number may increase or decrease in the presence of the deleted SMN1 gene. The importance of this phenomenon in this context is that abundant evidence in animal models and now in humans shows that the copy number of SMN2 is the most critical determinant of the severity of the SMA phenotype. a
a References .
Children with SMA carry various SMN2 copy numbers: of SMA type 1 patients, 80% to 96% carry one or two SMN2 copies (more than 70% carry two SMN2 copies) and 4% to 20% have three copies; of SMA type 2 patients, 82% have three copies of SMN2; and of SMA type 3 patients, 96% to 100% have three or four copies of SMN2 ( Fig. 32.2 ). However, the SMN2 copy number is not an accurate predictor of phenotype. Other contributing factors may include modifier genes affecting the motor neurons, and mutations in the SMN2 gene that alter the amount of full length SMN protein it produces. Even among family members who carry the same number of SMN2 copy numbers, variation is observed, suggesting that modulators of SMN2 splicing and other modifier genes may be involved.
TYPE OF MUTATION | TEST APPLIED | MUTATION DETECTION RATE |
---|---|---|
Homozygous deletion of exon 7 a | SMN1 Targeted mutation analysis PCR/restriction enzyme analysis or multiplex ligation probe amplification methodologies | ~95%–98% |
Compound heterozygosity (Deletion of SMN1 exon 7 [allele 1] and an intragenic mutation of SMN1 b [allele 2]) | Targeted mutation analysis combined with SMN1 gene sequence analysis c | 2%–5% |
SMN2 copy number d | Quantitative PCR analysis and other methodologies e | N/A |
a Testing for exon 8 deletion is not necessary.
b Small intragenic deletions/insertions and nonsense, missense, and splice site mutations.
c Whole-gene deletions/duplications are not detected.
d SMN2 copy number ranges from 0 to 5.
e MLPA, long-range PCR, chromosomal microarray (CMA) that includes the SMN1 , SMN2 chromosomal segment.

The biological functions of SMN1 and its relationship with SMA have been elucidated. The SMN protein interacts with other proteins in a multimolecular complex and appears to be involved principally in RNA metabolism. The most critical function of SMN is in the assembly of the ribonucleoproteins of the so-called spliceosome, which is critically involved in the removal of introns from pre-RNA and splicing together of exons in mature RNA, for many proteins. The biological functions potentially affected are many, but most recent work suggests that axonal growth and maintenance are key roles. Spinal cord motor neurons are selectively vulnerable to decreased SMN protein. These neurons (as well as others) have long axons and many targets, particularly in large muscles, and may heavily depend on axonal mRNA transport; SMN protein plays a role in axonal mRNA trafficking. Whether the pathogenesis of SMA is due to a splicing defect caused by SMN protein deficiency, disruption of an additional axonal SMN function, an unknown function, or a combination of the above is still unknown. Absence of SMN protein in cells is embryonic lethal in mice and other organisms. Why partial SMN deficiency specifically affects motor neurons in the spinal cord and brain stem remains unclear.
Clinical Features
Onset.
SMA type 1 is clinically apparent at birth or in the first several months of life. In one large series, clinical onset was at birth in 35%, in the first month in 16%, in the second month in 23%, and from the end of the second month to the sixth month in 26%. The finding of onset before 6 months with a median age of onset of 1 to 2 months is consistent. Onset in utero is supported by the observation that in most patients with SMA type 1 presenting at birth or in the early neonatal period, decreased and weak fetal movements in the last trimester are reported by the patients’ mothers. Neuropathological data (see later) have documented prenatal onset. In particularly severely affected infants, neonatal asphyxia or respiratory distress may occur. As just noted, some particularly severely affected infants (type 0) also may exhibit early deficits of facial movement, arthrogryposis, severe diffuse weakness, and early death. In those infants whose disease is not apparent at birth, onset in the first weeks is often acute. Indeed, clinical progression to severe disability characteristically occurs over a time course measured in days to a week or so. These clinical findings have a correlate from electrophysiological studies that show marked deterioration of motor function over a 1-week to 2-week period postnatally.
Neurological Features.
The neurological features are highly consistent and striking ( Box 32.4 ). a
a References .
Hypotonia is severe and generalized. Unlike the situation with disorders of the cerebrum and other central causes of hypotonia, weakness is similarly severe. Most infants exhibit generalized weakness, but when it is possible to make a distinction between proximal and distal muscles, a proximal more than distal distribution is discernible. Only minimal movements at hips and shoulders may be elicited in the presence of active movements of hands and feet. The lower extremities are affected more severely than the upper extremities. Involvement of the axial musculature of the trunk and neck is particularly severe, and the resulting deficits are obvious with vertical suspension and pull-to-sit maneuvers ( Fig. 32.3A–C ). Muscle atrophy is also severe and generalized, although the severity may be difficult to appreciate fully in the newborn. Indeed the replacement of atrophied muscle by connective tissue may further hinder the appreciation of atrophy. Fasciculations of limbs are observed rarely, but an analogous phenomenon may be the characteristic fine rhythmic tremor of the extended fingers (polyminimyoclonus) most commonly observed in types 2 and 3 SMA. Total areflexia is the rule, although rarely some reflex response, albeit depressed, can be elicited.Hypotonia: severe, generalized ( floppy infant )
Weakness: severe, generalized, or proximal > distal
Areflexia
Characteristic posture
Bell-shaped thorax, paradoxical or see-saw breathing
Weak cry
Difficulty sucking and swallowing
Relatively preserved facial movements
Normal extraocular movements
Preserved sensory function and sensorium
Normal sphincter function

The pattern of weakness of limbs leads to a characteristic posture, characterized particularly by a frog-leg posture with the upper extremities abducted and either externally rotated or internally rotated ( jug handle ) at the shoulders ( Fig. 32.4 ). The chest is almost always anteriorly collapsed and bell shaped, with an associated distention of the abdomen and intercostal recession during inspiration (see Fig. 32.4 ), known as paradoxical breathing. These features relate to weakness of the intercostal muscles and the relatively preserved diaphragmatic function. Contractures of the limbs, usually only at wrists and ankles, are evident in 10% to 20% of infants with onset in utero or in the first month of life.

Involvement of cranial nerve nuclei is less striking than of anterior horn cells, at least early in the clinical course. Thus extraocular movements are normal, and facial motility is relatively well preserved. Indeed, the pathetic picture of a bright-eyed, nearly totally paralyzed infant is characteristic of Werdnig-Hoffmann disease. Sucking and swallowing are affected early in the course in approximately half of cases. Fasciculations and atrophy of the tongue, clinical signs in anterior horn cell disorders of slightly later onset, are apparent in only about one-third to one-half of patients, with the disease occurring at birth or in the first month.
Particularly noteworthy differential diagnostic features in the neurological examination include the presence of normal sphincter and sensory functions. Both these functions would be expected to be affected with major spinal cord disease, and sensory function with peripheral nerve disease. The patient’s alert state with normal visual and auditory responses rules against a central disorder. Total areflexia and absence of ptosis, ophthalmoplegia, and facial weakness rule against a variety of primary diseases of muscle. Distinction from type II glycogen storage disease (Pompe disease) can be most difficult, but this much less common disease is usually accompanied by prominent involvement of the heart and enlargement of the tongue.
Several rare genetic variants of infantile SMA should be distinguished from the type 1 SMA just described. These SMA variants and their mode of inheritance include the association of SMA with (1) diaphragmatic SMA or SMA with respiratory distress 1 (SMARD1; autosomal recessive), (2) pontocerebellar atrophy (type 1 pontocerebellar atrophy; see Chapter 29 ) (autosomal recessive), and (3) congenital arthrogryposis, both with and without bone fractures (autosomal recessive and X-linked, respectively). None of these disorders are linked to the genetic region on chromosome 5 involved in type 1 SMA (see later discussion).
Course.
The course of type 1 SMA is a close function of the age of onset. a
a References .
Infants with clear prenatal onset of very severe disease with respiratory failure at birth, marked diffuse weakness, and early deficits of facial movement (i.e., type 0 SMA) usually die by 3 months of age. Those infants with clinical onset in the first 2 months of life have a range of median age at death of 4 to 8 months, with maximum survival rarely exceeding 12 to 18 months. Infants with clinical onsets after 2 months of age have clearly longer median and maximum survival times. Authors use various methodologies to report survival in SMA type 1 patients, as seen in Table 32.2 . Survival improves with aggressive pulmonary care and nutritional support. The very high survival rates noted in certain groups of type 1 patients (see Table 32.2 ) may be related to those factors, specifically invasive ventilation via tracheostomy and aggressive proactive care; however, these rates may also be related to the small number of patients in the reports.SURVIVAL PROBABILITY (%) | ||||||
---|---|---|---|---|---|---|
1 YEAR | 2 YEARS | 4 YEARS | 5 YEARS | 10 YEARS | 20 YEARS | |
Zerres and Rudnik-Schoneborn (1995) | — | 32 | 18 | — | 8 | 0 |
Mannaa et al. (2009) | — | 62 | 62 | — | 8 | — |
Ge et al. (2012) | 44.9 | 38.1 | — | 29.3 | — | — |
Farrar et al. (2013) | 40 | 25 | 6 | — | 0 | — |
AGE AT DEATH: MEDIAN | ||||||
(range, unless otherwise noted) | ||||||
Oskoui et al. (2007) | ||||||
1980–1994 | 7.3 m (1.0–193.5 m) | |||||
1995–2006 | 10.0 m (2.5–112.0 m) | |||||
Cobben et al. (2008) | 6 m (95% CI: 5–7 m) | |||||
Rudnik-Schoneborn et al. (2009) a | ||||||
All patients | 6.1 m (0.0–34.0 m) | |||||
2 copies SMN2 | 6.5 m (0.5–30 m) | |||||
3 copies SMN2 | Alive at 10–55 m | |||||
Ge et al. (2012) | 7.0 m | |||||
Finkel et al. (2014) b 2 SMN2 copies | 13.5 m (IQR: 81–22 m) 10.5 m (IQR: 8.1–13.6 m) |
a Age of all patients at death/permanent ventilation.
Although infants who ultimately prove to have type 2 SMA have a peak age at onset between 8 and 14 months, an occasional infant exhibits onset before 6 months and has a chronic course, including attainment of sitting or even walking, with prolonged survival. a
a References .
This portion of the type 2 SMA cases usually can be distinguished from type 1 SMA in the early stages of the disease because of a more insidious onset and slower initial deterioration. Death in type 1 SMA usually relates to intercurrent respiratory complications. These complications are caused by aspiration because of defective swallowing, hypoventilation because of impaired intercostal muscle function, and impaired clearing of tracheal secretions secondary to weak or absent cough because of weak abdominal muscles.Laboratory Studies
Serum Enzymes.
The creatine kinase (CK) level is usually normal in type 1 SMA or slightly elevated.
Electromyography.
The electromyogram (EMG) may be difficult to perform in the young infant, but with patience the major features of anterior horn cell disease are usually observed (see Table 30.4 ). Thus, at rest, fibrillation potentials are apparent. With muscle contraction, one sees a reduced number of fast-firing motor unit potentials that are usually of normal amplitude and duration because of failure to reinnervate efficiently in SMA type 1. In general, although no simple relationship exists between features on the EMG and outcome, one study showed a strong correlation between the degree of depression of the compound muscle action potential and functional outcome.
Nerve Conduction Studies.
Motor nerve conduction velocities are usually normal, although in particularly severe disease, a slight reduction may be observed. This finding suggests a disproportionate loss of anterior horn cells that give rise to the largest, fastest conducting fibers. The compound muscle action potentials (CMAPs) have very low amplitude because of significant loss of anterior horn neurons and/or axons. In contrast, the sensory nerve action potentials are normal, which underscores the motor neuron nature of the disease. Nevertheless, some patients with particularly severe cases of type 1 SMA have exhibited signs of severe motor and sensory neuropathy.
Muscle Biopsy.
The muscle biopsy of a patient with Werdnig-Hoffmann disease, with clinically apparent findings at birth or in the first month, usually exhibits advanced changes of denervation (see Chapter 30 ). Characteristically, pronounced atrophy of all fibers of both types within entire fascicles of muscle (i.e., panfascicular atrophy ) or large group atrophy occurs ( Fig. 32.5 ). The early signs of denervation (i.e., loss of checkerboard appearance, type grouping, or small group atrophy; see Chapter 30 ), features of anterior horn cell disease later in childhood, are nearly absent ( Fig. 32.6 ). This virtual absence of signs of terminal axonal sprouting and reinnervation (see Chapter 30 ) is probably indicative of the severe, fulminating process involving anterior horn cells that causes widespread elimination before such compensatory attempts can occur. A consistent increase in connective tissue is associated with the marked atrophy of muscle (see Fig. 32.6 ). Hypertrophy of predominantly type I fibers, often in clusters, is an additional characteristic feature. The finding that these hypertrophic fibers often occur in clusters suggests the possibility of reinnervation. The distribution of the histopathological findings parallels the generalized pattern of muscle weakness; the diaphragm is conspicuously less affected. Diagnosis by genetic testing has obviated the need for muscle biopsy in most cases (see later).


Other Studies.
The identification of the q11.2–13.3 region of chromosome 5 as the site of the genetic defect in Werdnig-Hoffmann disease led to the development of molecular diagnostic techniques (see “ Pathogenesis and Etiology ” earlier). Radiographs of the chest are useful in demonstrating the chest deformity, the thin ribs characteristic of severe congenital neuromuscular disease, and the marked atrophy of muscle. In the rare, very severe type 0 disease, long bone fractures may be observed.
Studies with real-time ultrasonography of limbs have demonstrated several types of changes (i.e., an increase in the intensity of echoes from muscle and the degree of muscle atrophy). The echogenicity of muscle correlates well with the severity of histopathological changes observed in biopsy specimens.
Neuropathology
Essential Cellular Changes.
The major neuropathological changes are confined to the anterior horn cells of the spinal cord and the motor nuclei of cranial nerves ( Box 32.5 ). The essential cellular changes in infantile cases are (1) depletion of the number of neurons, (2) degenerative changes of neurons, (3) neuronophagia, and (4) infiltration with microglia and astrocytes ( Fig. 32.7A and B ). Enhanced apoptosis of anterior horn cell neurons is the most prominent feature during fetal life. Some workers also described cytological findings indicative of immaturity of neurons and suggested impaired development, as well as degeneration, of neurons. The depletion of neurons may be so marked that few, if any, remain in an anterior horn or cranial nerve nucleus. The degenerative changes consist particularly of central chromatolysis, characterized by rounded and distended neuronal cell bodies with eccentric nuclei and Nissl substance displaced to the periphery. Neuronophagia is not prominent, although it is readily demonstrated. The glial response consists of both microglia and astrocytes and is particularly prominent in the ventral aspect of the anterior horns.

Topography.
In severe Werdnig-Hoffmann disease, anterior horn cells are affected diffusely, with a particularly prominent affection of the ventromedial group. Cranial nerve nuclei involved invariably and markedly are of cranial nerves VII, IX, X, XI, and XII. The motor nucleus of nerve V is affected in approximately 70% of cases, and the abducens nucleus is involved in fewer than 50% of cases.
Other areas of the motor system are not consistently involved. Hypoxic changes are occasionally observed in hippocampal neurons and Purkinje cells of cerebellum. As noted later, associated degenerative changes in pontocerebellar structures are a feature of an SMA variant that is genetically distinct from type 1 SMA.
The motor nerves exhibit the changes expected from a loss of anterior horn cells (i.e., a decrease in number of myelinated fibers particularly and an increase in connective tissue). Striking atrophy with glial proliferation may be apparent in the proximal anterior roots. Indeed, the glial proliferation may be so conspicuous that some workers have attributed to it a pathogenetic role. The view that the glial infiltration is a secondary event is more widely accepted. Perhaps of greater interest is the demonstration of changes typical of axonal neuropathy in some severe cases. Such changes have led to the suggestion that the anterior horn cell degeneration is a secondary phenomenon, although the most prevalent view is that the neuropathic abnormalities are secondary. Kariya et al. also demonstrated structural and functional abnormalities at the level of the neuromuscular junction that precede symptoms in mice, as well as structural abnormalities in the neuromuscular junctions of humans with SMA, and hence proposed that SMA may be a synaptopathy .
Management.
Type 1 SMA that is apparent at birth or in the first month of life is usually accompanied by serious disturbances of sucking and swallowing early in infancy. Frequent aspiration of the oropharynx is needed, and usually cessation of oral feedings and institution of tube feeding are required before long to ensure adequate nutrition. Indeed, when dysphagia becomes severe enough to preclude reasonable nutrition and is complicated by frequent aspirations, many clinicians recommend gastrostomy or gastrojejunostomy feeding. This maneuver is carried out not to prolong life but to improve the patient’s quality of life and particularly to reduce parental anxiety associated with attempts at oral feeding. Surveillance for respiratory infection must be diligent, because this complication is the usual mode of demise for these unfortunate babies. The intensity of therapy (e.g., antibiotics, chest physical therapy, postural drainage, noninvasive positive pressure ventilation, and even tracheostomy and long-term mechanical ventilation) sometimes is difficult to determine decisively. Tracheostomy and mechanical ventilation have been associated with survival into childhood, although longer survival occurs, albeit rarely, without such invasive intervention. The quality of life in such bedridden infants and children is a grave concern. More important, range-of-motion exercises are used to prevent contractures and, I feel (JJV), to help the parents “do something” for their infant. In severe Werdnig-Hoffmann disease, my approach is foremost to provide emotional support to the parents and to ensure as much comfort as possible for the infant. I indicate to the family our current understanding of the dire outcome and recommend careful attention to oral-pharyngeal secretions, measures to ensure adequate nutrition, and diligent therapy of respiratory infections. I discuss the complex issues related to long-term mechanical ventilation.
The clinical trials pipeline for SMA over the past 15 years has been developing several therapeutic agents. With the goal of increasing SMN transcript, histone deacetylase inhibitors (such as valproic acid and sodium phenylbutyrate) were tested in randomized trials, but neither was effective ; nonhistone deacetylase inhibitors (such as hydroxyurea) were ineffective in a randomized trial; albuterol showed promise in two pilot studies; however, the mechanism of action remains unknown. Ionis Pharmaceuticals has conducted phase 3 clinical trials of an intrathecally delivered antisense oligonucleotide (SMNRx, or Nusinersen) targeting SMN2 exon 7 inclusion. Interim analysis of a phase 3 Nusinersen SMA type 1 study (ENDEAR) showed that infants receiving the medication intrathecally experienced a statistically significant improvement in the acquisition of motor milestones, compared with a control cohort, and led to the approval of nusinersen (Spinraza) by the FDA in December 2016. Early phase clinical trials are testing other orally administered small molecules that modulate SMN2 alternative splicing. Roche-PTC-SMA Foundation partnership put on hold clinical studies of RG7800, an orally administered molecule, after an unexpected safety finding at higher long-term doses in an animal study. They have recently announced phase 1 trials for a new molecule, RG7916. Novartis has entered phase 2 of a clinical trial for the orally administered molecule LM1070. The goal of stabilizing the SMN protein has led to preclinical studies of aminoglycosides, proteasome inhibitors, and indoprofen. Among neuroprotectors, olesoxime, a mitochondrial pore modulator that enhances motor neuron survival in culture, may be effective in stabilizing the disease, but riluzole and gabapentin have shown mixed results. Cell therapy through stem cells is under development; gene replacement therapy with a scAAV9/SMN1 gene construct in type 1 infants is in progress, and preliminary results seem promising. The goal of enhancing muscle function is on the horizon, with new potential therapeutic targets such as myostatin, follistatin, and other molecules.
Spinal Muscular Atrophy Variants
At least four disorders with primary involvement of anterior horn cells, clinical presentation at birth, and subsequent course of progressive deterioration (or severe static disability) mimic SMA in the neonatal period. However, these disorders are not linked to chromosome 5q and do not involve SMN. Thus the term SMA variants or non-5q SMAs seems most appropriate until the molecular bases are further clarified. At least four disorders should be distinguished ( Table 32.3 ).
VARIANT | MAJOR FEATURES |
---|---|
SMA with respiratory distress type 1 (SMARD1) | Mild hypotonia, weak cry, distal contractures initially; respiratory distress from diaphragmatic paralysis 1–6 months, progressive distal weakness; autosomal recessive, locus 11q13.3, gene: immunoglobulin mu-binding protein 2 (IGHMBP2) |
Pontocerebellar hypoplasia type 1 (PCH1) | Arthrogryposis, hypotonia, weakness, bulbar deficits early; later, microcephaly, extraocular defects, cognitive deficits: pontocerebellar hypoplasia; molecular defect unknown; autosomal recessive, genes VRK1 and EXOCS3 |
X-linked infantile SMA with arthrogryposis ± bone fractures (SMAX2) | Arthrogryposis, hypotonia, weakness, congenital bone fractures, respiratory failure, lethal course as in severe type 1 SMA: most cases X-linked (Xp11.1–3, gene UBA1 ); a few cases likely autosomal recessive |
Congenital SMA with predominant lower limb involvement | Arthrogryposis, hypotonia, weakness, especially distal lower limbs early; nonprogressive but severe disability; autosomal dominant or sporadic; locus 12q24, gene TRPV4 |
SMA with respiratory distress type 1 (SMARD1) is a striking disorder. From the first days of life, intrauterine growth retardation, mild hypotonia, weak cry, and mild distal contractures are noted. Between 1 and 6 months of age, respiratory distress secondary to diaphragmatic paralysis becomes obvious, and progressive primary distal lower limb weakness ensues. Motor, sensory, and autonomic disturbances develop; these infants become ventilator dependent. A report from the Netherlands of 10 patients with SMARD1 noted significant phenotypic variability and no clear phenotype-genotype correlations. CPK is usually normal; there is no cardiac involvement. Life expectancy in children with SMARD1 is limited; however, patients rarely can have only mild sleep hyperventilation. The responsible gene (IGHMBP2) encodes immunoglobulin mu-binding protein 2, the function of which is unclear.
Pontocerebellar hypoplasia type 1 (PCH1) manifests clinically at birth with arthrogryposis, hypotonia, weakness, and bulbar deficits (swallowing difficulty, stridor). Subsequent features include progressive bulbar deficits, nystagmus, microcephaly, and cognitive deficits. Brain imaging shows pontocerebellar hypoplasia and cerebral atrophy. PCH1, linked to mutations in the VRK1 gene, is an unusual phenotype wherein a characteristic brain malformation combines with abnormalities of spinal cord motor neurons. EXOCS3 was recently sequenced in a cohort of 27 families with PCH1; mutations were found in 37%. A common c.395A>C, p.D132A mutation was present in about half of these families. Despite some variation in clinical features, phenotype-genotype correlation underscored the continued usefulness of PCH1 as a clinical category.
X-linked infantile SMA with arthrogryposis ± congenital fractures (SMAX2) manifests clinically in the neonatal period with arthrogryposis, hypotonia, areflexia and weakness. Congenital fractures of long bones and ribs are common. Respiratory failure and progressive weakness lead to death in the first days to weeks in most infants. Anterior horn cell loss is marked. Approximately 80% of cases have been in male infants, and linkage to Xp11.3 has been shown. However, some cases with predominance of bone fractures likely are autosomal recessive. The lethal X-linked form of SMA (SMAX2) can be confused with SMA type 1A; mutations in the UBA1 gene have been detected in this group of patients. A clinical and pathological study of a UBA1 gene mutation-positive SMAX2 case showed white matter abnormalities on MRI brain imaging, prominent motor and sensory system disturbance, as well as cerebellar involvement and widespread inflammatory changes on muscle biopsy.
Congenital distal SMA predominantly affecting the lower limbs is the only autosomal dominant disorder of this group. Presentation at birth is with talipes equinovarus and lower limb hypotonia. EMG and muscle biopsy show signs of anterior horn cell disease. The subsequent course is marked by severe weakness of the lower extremities, although it is generally nonprogressive. The disorder is linked to chromosome 12q24.11, and the molecular defect involves mutations in the TRPV4 gene.
Neurogenic Arthrogryposis Multiplex Congenita
Generalized and Localized Types
The term neurogenic has been applied to cases of arthrogryposis multiplex congenita secondary to involvement of either anterior horn cell or peripheral nerve (see Chapter 31 ). Obvious overlap exists with type 0 SMA and with the SMA variants just discussed. I prefer to use the term neurogenic arthrogryposis multiplex congenita for the clearly nonprogressive forms of arthrogryposis related to anterior horn cell or peripheral nerve disease. By far the most common variety is amyoplasia congenita, probably related in most cases to a dysgenetic disturbance of anterior horn cells, described in Chapter 31 . Thus because this and other generalized forms of arthrogryposis multiplex congenita secondary to anterior horn cell have been discussed, I consider in this section only the localized forms.
Cervical Form.
A group of infants with a cervical form of anterior horn cell disease and arthrogryposis was described. The striking findings in the neonatal period were signs of severe symmetrical lower motor neuron deficit in the upper extremities in the absence of a history suggestive of traumatic injury of spinal cord or brachial plexus. Atrophy and flaccid weakness of upper extremities and the proximal and distal muscle groups were marked, and flexor contractures at the elbow and interphalangeal joints were apparent. Bulbar muscles were not affected. Lower extremities were normal. The course was nonprogressive. Onset in utero was suggested by the presence of flexion contractures, and onset in the first trimester was suggested by the presence of poorly formed palmar creases. The nature of the intrauterine insult to cervical anterior horn cells was not clear. One patient with a clinically similar case had intramedullary telangiectasia.
Caudal Form.
A caudal form of anterior horn cell disease with arthrogryposis was described as an apparently sporadic disorder with involvement localized to the lower extremities. Weakness, hypotonia, areflexia, and multiple joint contractures were the major features. A few patients later developed signs in the upper extremities and fasciculations of the tongue after several years. Whether these cases represent a different disorder from the SMA variant with predominant lower limb weakness described earlier (see Table 32.3 ), or are related to intrauterine infections of the anterior horn cells, is unknown.
Laboratory Studies.
EMG and muscle biopsy in both the generalized and local forms of neurogenic arthrogryposis multiplex congenita usually demonstrate signs of denervation (see Chapter 30 ). In the localized forms, the possibility of a correctable structural lesion of the cervical cord or the lumbosacral cord and cauda equina should be ruled out by appropriate studies.
Type II Glycogen Storage Disease (Pompe Disease)
Type II glycogen storage disease, Pompe disease , is an inherited disorder, transmitted in an autosomal recessive manner. The disease is associated with glycogen deposition in anterior horn cells (as well as in skeletal and cardiac muscle, liver, and brain), and with striking weakness and hypotonia in early infancy.
Clinical Features
Onset.
Onset of the Pompe disease may be apparent in the first days of life, although the median age at symptom onset in the largest series ( n = 168) was 2.0 months.
Neurological Features.
Initially, the weakness and hypotonia may be so severe that type 1 SMA is considered the probable diagnosis. The concurrence of fasciculations of the tongue and difficulty sucking, crying, and swallowing further mimics this primary anterior horn cell degeneration. However, several clinical features help distinguish Pompe disease from Werdnig-Hoffmann disease. First, cardiac involvement, resulting from accumulation of glycogen, is prominent in Pompe disease; radiographs demonstrate an enlarged globular heart, and electrocardiograms demonstrate evidence of myocardiopathy with shortened PR interval, giant QRS complexes, and inverted T waves. Second, the tongue, although weak and perhaps even fasciculating, is usually large in Pompe disease because of the glycogen accumulation, unlike the small, atrophic tongue of Werdnig-Hoffmann disease. Third, the skeletal muscles, although weak, are usually prominent in Pompe disease, because of glycogen accumulation (i.e., a true hypertrophy), and they have a characteristic rubbery feel. This finding is unlike the atrophy of Werdnig-Hoffmann disease. Fourth, the liver usually is enlarged and readily palpable in Pompe disease. Tendon reflexes are variable in glycogen storage disease. Most patients have preserved tendon reflexes early in the clinical course and later have total areflexia.
A recent report described a delay in myelination, determined by MRI, in five patients with infantile-onset Pompe disease. The clinical correlates of this disturbance remain to be clarified. Deposition of glycogen is a feature of human oligodendroglial development.
Clinical Course.
The clinical course is malignant. Infants require ventilatory support at a median age of 6 months, and death in one large series ( n = 168) occurred at a median age of approximately 9 months. In another series ( n = 153), the median age at death was 6 months. Survival rates at 12 months of age are approximately 25%, with only 17% ventilator free; at 18 months, the respective values are 12% and 7%. Death is related usually to a combination of cardiac involvement and respiratory complications caused by thoracic muscle weakness and bulbar paralysis.
Laboratory Studies.
The serum CK level is elevated because of cardiac and skeletal muscle involvement, and the serum transaminases also are usually elevated because of the combination of muscle and hepatic disease, with the former predominating. Muscle biopsy reveals large amounts of periodic acid–Schiff–positive material. Large vacuoles, often confluent, are apparent when standard fixation procedures are used. The confluent vacuoles, often resulting in a lacework appearance, are lysosomes filled with PAS-positive diastase-digestible material; they stain positively for acid-phosphatase. Also, free glycogen can be seen in the cytoplasm with electron microscopy ( Figs. 32.8A and B and 32.9 ). The EMG reveals fibrillations but often also signs of myopathic disorder because of prominent muscle involvement (see Chapter 33 ). An additional characteristic feature is irritability of muscle with the occurrence of pseudomyotonic bursts of discharges. The large R waves on electrocardiography are particularly helpful signs in diagnosis.


Neuropathology.
The neuropathology of Pompe disease is characterized by a striking increase in glycogen throughout the central and peripheral nervous systems (including the myenteric plexus of rectal mucosa). The accumulation of glycogen in the anterior horn cells is the most striking change.
Pathogenesis and Etiology.
The biochemical defect involves acid alpha-glucosidase (GAA; acid maltase) activity ( Fig. 32.10 ). This enzyme catalyzes the lysosomal pathway of glycogen degradation, presumably used during normal turnover of cellular constituents. (The major pathway of glycogen degradation, used for glucose-6-phosphate production, occurs in the cytosol and is catalyzed by phosphorylase.) Thus, in Pompe disease, the largest accumulation of glycogen is apparent in lysosomal structures. The enzymatic defect is demonstrable in muscle or cultured fibroblasts. Moreover, the enzyme is also present in fibroblasts grown from amniotic fluid cells, and this procedure can be used for prenatal diagnosis.

There is good correlation between GAA gene mutations and severity of the phenotype. Infants tend to have deletion or nonsense mutations in the GAA gene, leading to significant enzyme deficiency, whereas older children and adults have milder, leaky mutations.
Management.
Attempts to replenish alpha-glucosidase by bone marrow transplantation have been unsuccessful. Very promising results are available for the use of enzyme replacement therapy (ERT), involving recombinant human alpha-glucosidase, at least for survival and cardiac and skeletal muscle function. In addition, a study of five infants treated from a median age of 6 months showed improvement in myelination by MRI in four of the patients. However, the response of infants with Pompe disease has been variable, with some infants surviving for more than 11 years but being paralyzed and ventilator-dependent; early treatment seems to improve the outcome. In a series of 20 infants treated in the UK between 2000 and 2009, the overall ventilator-free survival was 35%; 30% were alive and ventilator-dependent, and 35% died at a median age of 10 months. Further, questions regarding cognitive outcome have been raised in surviving infants because the recombinant GAA does not cross the blood-brain barrier.
Neonatal Poliomyelitis
Neonatal poliomyelitis in this section refers to infection with poliovirus acquired either in utero or in the first 4 weeks of life. Only one case has been reported in the United States in the approximately 40 years since the widespread use of live oral poliovirus vaccine (Sabin vaccine). An additional infantile case with onset at 3 months of age occurred 1 month after administration of live oral polio vaccine. However, poliomyelitis at later ages is still observed, not only in other parts of the world but in the United States among certain religious groups that prohibit vaccination of children. In addition, approximately nine cases per year of poliomyelitis caused by vaccine-related poliovirus still occur in the United States. Indeed, the single neonatal case reported in the past 3 decades in this country acquired the infection by contact with a recently vaccinated infant with diarrhea. Moreover, other enteroviruses rarely cause a paralytic syndrome in older patients and, theoretically at least, could cause a neonatal disorder comparable to poliomyelitis. For these reasons, brief attention to neonatal poliomyelitis is warranted here.
Clinical Features
Onset.
The disorder may be apparent at birth or may develop in the first weeks of life. Because the incubation period of the disease is approximately 10 days, infants affected at birth or in the first week presumably were affected in utero.
Neurological Features.
Most patients present with diffuse flaccid paralysis that is usually asymmetrical. Apnea may be the initial clinical feature. Not uncommonly, encephalitic involvement results in cerebral signs, including seizures. Respiratory failure may ensue promptly. Moreover, contractures can evolve rapidly, and care must be taken to avoid the postnatal development of arthrogryposis multiplex. Asymmetrical paresis is a common sequel.
Laboratory Studies.
The diagnosis is established by viral isolation from the stool. CSF pleocytosis with elevated protein content occurs.
Neuropathology.
The neuropathological features in the newborn are often more severe than in the adult. The qualitative features are similar. Neuronal necrosis, neuronophagia, and perivascular cuffing with inflammatory cells involve neurons of the anterior horns, motor nuclei of the brain stem, roof nuclei of the cerebellum, diencephalon, and motor cortex of the cerebrum.
Pathogenesis and Etiology.
Neonatal poliomyelitis is caused by infection of neurons by poliovirus, a classic neurotropic virus. The acquisition of virus may occur prenatally or early postnatally. The demonstration of maternal viremia and placental infection and the recovery of virus from meconium of stillborn infants of diseased mothers support the concept of intrauterine infection by transplacental mechanisms. Because of the incubation period for poliomyelitis, infants affected after approximately 10 days of age presumably were infected during delivery by contamination with infected stool or by postnatal exposure.
Management.
Management is entirely supportive.
Level of the Peripheral Nerve
Disorders affecting the peripheral nerve are the least defined of those leading to hypotonia and weakness in the neonatal period. However, the more frequent consideration of disorders of peripheral nerve as the cause of motor deficits in neonatal patients, the more frequent use of refined techniques for studying nerve histology, such as electron microscopy, and the explosion in application of molecular genetics lead to the conclusion that disease at this level is probably considerably more common than was previously suspected. The major disorders to be considered in the neonatal period are shown in Box 32.6 . The categorization is somewhat arbitrary because available reports suggest some overlap, as well as the need for more definitive means of study.
Chronic Motor-Sensory Polyneuropathy
Myelin
Hypomyelination a
a Each accounts for approximately 25% of all chronic infantile motor-sensory polyneuropathies.
Autosomal recessive
Sporadic
Hypomyelination and demyelination-remyelination (onion bulbs)
Autosomal recessive a
Autosomal dominant a
Sporadic
Other (associated with axonal disease or focal hypermyelination)
Chronic inflammatory demyelinating polyneuropathy
Neuronal-Axonal (Rare)
Autosomal recessive
Autosomal dominant
Sporadic
Subcellular
Mitochondrial: disorders of electron transport chain complex subunits, pyruvate dehydrogenase
Cytoskeletal structures: intermediate filaments (i.e., giant axonal neuropathy), neurofilaments (i.e., infantile neuroaxonal dystrophy)
Lysosomal: Krabbe disease
Hereditary Congenital Sensory and Autonomic Neuropathies
Familial dysautonomia, congenital insensitivity to pain with or without anhidrosis and cognitive impairment
Acute Polyneuropathy
Guillain-Barré syndrome
Chronic Motor-Sensory Polyneuropathy: Myelin Disease
In chronic motor-sensory polyneuropathies (see Box 32.6 ), we include several groups of cases, somewhat heterogeneous in basic type but readily identified as affected with a disorder of peripheral nerve myelin. The disorders are categorized best as those that appear to involve a failure of the Schwann cell’s role of synthesis and maintenance of myelin (i.e., characterized by hypomyelination ). In many cases, an additional feature, related to proliferation of Schwann cells and fibroblasts, is “onion bulb” formation , with evidence of demyelination-remyelination. In a rare third group, the disturbance of myelin is inflammatory . I discuss the two most commonly recognized of these three categories, the hypomyelinative and the hypomyelinative-demyelinating-remyelinating polyneuropathies, together, because their clinical features overlap, and in many respects the disorders are interrelated.
Congenital Polyneuropathies With Hypomyelination, With or Without Demyelination and Remyelination
In this group I include those neuropathies associated with hypomyelination alone and those with hypomyelination and evidence of demyelination-remyelination (i.e., onion bulb formation). Those disorders with onion bulb formation were previously termed Dejerine-Sottas disease (DSD) or hereditary motor sensory neuropathy (HMSN) type III , and those with hypomyelination alone were termed congenital hypomyelinating neuropathy (CHN). The designation Charcot-Marie-Tooth disease type 4 (CMT4) has been used inconsistently in the past to denote autosomal recessive demyelinating neuropathies, some of them with early onset. However, this series of terms was coined before the availability of genetic testing and now may cause confusion. The principal overall features of the entire group are summarized in Table 32.4 .
Pathogenesis and Etiology
The pathogenesis of congenital hypomyelinative neuropathies is diverse but in general involves a disturbance of myelin formation or maintenance. The major genes involved, myelin protein zero (MPZ), peripheral myelin protein 22 (PMP22), early growth response element 2 (EGR2), myotubularin-related protein 2 (MTMR2), ganglioside-induced differentiation-associated protein 1 (GADP1), periaxin (PRX), and neurofilament protein (NEFL), are either structural myelin or Schwann cell proteins or transcription factors. Nevertheless, mutations in many other genes have been linked to the pathogenesis of these disorders ( Table 32.5 ), including SH3TC2, a frequent cause of autosomal recessive CMT4. Mutations in SH3TC2 account for the etiology in 12% of congenital neuropathies, which is the highest frequency of all genes involved.
CLINICAL FEATURE | CLINICAL PHENOTYPE | GENE |
---|---|---|
Pupillary abnormalities | CHN, DSD, CMT1B CMT4C | MPZ SH3TC2 |
Multiple cranial neuropathies | CHN, DSD, CMT4E | EGR2 |
Prominent foot and hand deformities | CMT4A, CMT2H/K CMT4C | GDAP1 SH3TC2 |
Severe spinal deformities | CMT4C | SH3TC2 |
Asymmetric weakness | DSD, CMT4J GBS/CIDP | FIG4 — |
Posttraumatic rapid progression of weakness | DSD, CMT4J | FIG4 |
Vocal cord paresis | CMT4A, CMT2H/K CMT4B1 | GDAP1 MTMR2 |
Diaphragmatic involvement | CMT4A, CMT2H/K | GDAP1 |
Glaucoma | CMT4B2 | SBF2 |
Prominent sensory involvement | DSD, CMT4F | PRX |
Congenital cataracts | HCC CCFDN | DRCTNNBIA CTDP1 |
Raised creatine kinase | Merosin-deficient CMD | LAMA2 |
Congenital hypomyelinative neuropathies overall have occurred sporadically and by autosomal recessive, autosomal dominant, or X-linked recessive inheritance. The last of these is related to a connexin-32 defect and nearly always presents clinically in childhood. The purely hypomyelinative cases are caused by either de novo dominant or autosomal recessive mutations. Among the hypomyelinative group with onion bulb formation (DSD or HMSN III), autosomal recessive inheritance is most common, but autosomal dominant inheritance has also been defined (often categorized as a subtype of HMSN I or Charcot-Marie-Tooth disease, CMT1).
Autosomal dominant inheritance may be more common than suspected. Thus when nerve conduction velocity determinations have been performed in apparently unaffected parents of some affected infants, clear evidence of disease has been detected. Indeed, in the series of 20 patients with congenital polyneuropathy described by Hagberg and Lyon, five had clear evidence of autosomal dominant inheritance and appeared to represent the HMSN I or CMT1. The most common genetic defect for the latter phenotype is a duplication of the chromosomal 17p region that contains PMP22. Although neonatal electrophysiological abnormalities have been identified in 17p duplication cases, clinical neonatal onset is unusual, but it does occur. Autosomal dominant point mutations of both PMP22 and P0 have been shown to lead to CHN and could have accounted for the earlier observations of Hagberg and Lyon.
Less commonly, hypomyelinative-demyelinating-remyelinating forms are associated with axonal disease or with focal areas of hypermyelination, in addition to onion bulbs. These focal myelin thickenings or tomacula presumably reflect a separate defect in the Schwann cell that differs from those seen in the more typical cases described in Table 32.4 .
Clinical Features
In most cases, the disorder has its onset in utero and manifests at birth. The dominant neurological features in the neonatal period are hypotonia and weakness. Involvement usually is generalized, and the distal more than proximal involvement characteristic of peripheral nerve disease has been difficult to demonstrate in the newborn period. This pattern of weakness, however, usually becomes obvious after several months. Muscle atrophy is usually marked. Tendon reflexes are very hypoactive or, more often, absent. Arthrogryposis multiplex congenita has been a feature in a number of cases and may be very prominent (see Table 32.4 ).
The involvement of musculature innervated by cranial nerves has been variable. Impairment of feeding is common, but the nature of the disturbance is not usually characterized. Clear involvement of facial movement is not common, and extraocular abnormalities have not been reported.
Involvement of the sensory system is a valuable feature to elicit for identification of neuropathy. Elicitation of sensory deficits in the neonatal patient requires very careful examination (see Chapter 9 ). Definitive evaluation of the sensory system usually also requires electrophysiological data (see later discussion). Most patients (70%) never walk, and only 11% eventually do so unassisted (see Table 32.4 ). Approximately 40% of infants die, and a clear relation to severity of disease, as reflected in the presence or absence of evidence for demyelination-remyelination (i.e., onion bulbs on nerve biopsy), is apparent. Thus approximately 85% of infants with absence of onion bulbs have died, whereas 81% of those with onion bulbs survive (see Table 32.4 ).
Three reports of infants with the clinical electrophysiological and pathological features of congenital hypomyelinating polyneuropathy who improved spontaneously after months and were normal or markedly improved at 18 or 19 months and 9 years, respectively, raised the possibility of a transient, reversible form of this disorder. These observations suggest the need for some caution in rendering a dismal prognosis with the neonatal diagnosis of this form of neuropathy.
Some clinical clues to the diagnosis of congenital hypomeylinating polyneuropathies, and associated genes and phenotypes, are shown in Table 32.5 .
Laboratory Studies
Serum Enzymes.
The CK level is normal.
Electromyography.
The EMG indicates changes of denervation, as outlined in Table 30.4 .
Nerve Conduction Velocity.
Determination of nerve conduction velocity is critical and is too frequently overlooked in the evaluation of the hypotonic and weak newborn. In most severely affected infants, a drastic reduction in motor nerve conduction velocity has been demonstrated, and values of less than 10 to 12 m/second are recorded in nearly all cases (see Table 32.4 ). In many infants (including several of my patients), impulse transmission could not be detected. The absence of sensory nerve action potentials is also common.
Muscle Biopsy.
Muscle biopsy usually shows evidence of denervation (see Box 30.4 ), but the muscle histology occasionally may appear remarkably preserved in the presence of severe disease of nerve. The correct diagnosis may be suggested by examination of intramuscular nerves within the biopsy specimen of muscle. Changes seen in full form on nerve biopsy (see later discussion) may be suggested from such examination.
Other Studies.
The CSF protein concentration is a valuable adjunct to the diagnosis of infantile polyneuropathy. The CSF protein concentration is almost always elevated, even in the first weeks of life. The elevation usually becomes more marked with age, and occasionally a CSF protein concentration that is only marginally elevated in the first weeks of life becomes markedly elevated later in infancy.
Neuropathology
The diagnosis of peripheral nerve disease is established by examination of a nerve biopsy specimen. The sural nerve, which has a limited distribution over the skin of the foot, is usually chosen for biopsy. The major abnormalities are hypomyelination and onion bulb formation . Also, the discovery of mutations in a large number of genes in patients with congenital polyneuropathies has allowed for molecular diagnosis of these disorders (see earlier).
Hypomyelination.
In the congenital hypomyelinative neuropathies, the essential feature has been the virtual absence of myelin sheaths ( Fig. 32.11 ). If any myelin is present, large-diameter nerve fibers, which normally are heavily myelinated, are conspicuous by the paucity of myelination. The normal proportion between diameter size and thickness of myelin sheath thus is lost. No signs of myelin destruction can be discerned; the lack of a demyelinating process is supported by biochemical studies, which showed no accumulation of cholesterol ester, the chemical hallmark of demyelination. Accompanying these changes may be a modest proliferation of Schwann cells and endoneurial fibroblasts.


Onion Bulb Formation.
In many cases, so-called onion bulb formation has been detected. This morphological change is associated with the proliferation of Schwann cells and, to a lesser extent, endoneurial fibroblasts. Individual axons become invested by multiple Schwann cells. The multiple concentric lamellae of Schwann cell processes and, to a lesser extent, collagen fibers render the onion bulb appearance ( Fig. 32.12A–D ). In the youngest and most severely affected patients, the concentric lamellae consist principally of basement membrane of Schwann cells, there remaining little of Schwann cell plasma membrane. In older and usually less severely affected patients, the proliferative changes cause considerable separation of nerve fibers and an increase in the transverse diameter of the nerve; hence the term interstitial hypertrophy . In such patients, nerves may be palpable, particularly the posterior auricular in the neck, the ulnar at the elbow, and the peroneal at the fibular head.




Management
Supportive measures (see the earlier discussion of Werdnig-Hoffmann disease ) are important, particularly because many patients live for years. It is critical to prevent contractures, scoliosis, and recurrent pulmonary infection and to optimize the motor function that is retained.
Chronic Inflammatory Demyelinating Polyneuropathy
Chronic inflammatory demyelinating polyneuropathy (CIDP) is a relatively common acquired neuropathy of older children and adults. The disorder is important to recognize because it is treatable. It may be apparent in the neonatal period. Decrease in fetal movement and the occurrence of contractures indicate that prenatal onset may occur. The newborn exhibits a poor suck and is hypotonic and weak. Weakness is either in a distal more than proximal or distal equal to proximal distribution. Areflexia is common. The diagnosis is suggested by the finding of elevated CSF protein level, delayed motor nerve conduction velocities (<70% of normal and usually considerably less), and electrophysiological evidence of an acquired neuropathy (multifocal disease with conduction block and temporal dispersion). Nerve biopsy shows diminution in myelin and evidence of segmental demyelination and remyelination, but especially subperineurial and endoneurial edema with inflammatory cells. Treatment with corticosteroids has been markedly beneficial. Thus in infants with signs of demyelinating neuropathy and no evidence of a familial disorder, this disorder should be considered seriously, because therapy can lead to major clinical improvement. Notably, one reported patient began to improve without therapy by 2 months and fully recovered by age 6 months. It should be noted, however, that certain genetic forms of demyelinating neuropathies, such as CMT4J (FIG4 gene mutations), may present during infancy with a clinical and electrophysiologic phenotype of Guillain-Barré syndrome or CIDP ( Table 32.5 ). Lack of response to immunotherapy should alert the clinician to the possibility of an underlying genetic defect.
Chronic Motor-Sensory Neuropathy: Neuronal-Axonal Disease
A rare cause of hypotonia and weakness in the newborn period is peripheral nerve disease on the basis of neuronal-axonal involvement. Two groups of patients with neuronal-axonal neuropathy should be distinguished: those with and those without associated Werdnig-Hoffmann disease. Of note, the term DSD also includes axonal forms of CMT with early onset.
Axonal polyneuropathy unassociated with Werdnig-Hoffmann disease has been reported only rarely. a
a References .
In the series of 20 cases of congenital polyneuropathy studied by Hagberg and Lyon, only a single case was of the neuronal-axonal type. Neonatal features have included marked hypotonia and weakness and respiratory distress. Arthrogryposis multiplex congenita may be present. The clinical course is usually static or subject to improvement. Results of the EMG and muscle biopsy indicate denervation. Nerve conduction velocities are normal or only modestly depressed, in contrast to the markedly depressed velocities in hypomyelinative neuropathy (see later discussion). Sural nerve biopsy shows axonal degeneration ( Fig. 32.13 ). Secondary changes in myelin surrounding the degenerating axons may occur (see Fig. 32.13 ). Sporadic cases have been most common, although autosomal dominant inheritance has been recorded. A subset of these cases, with a more severe course, consists of infants with SMARD1 , discussed earlier, because of accompanying anterior horn cell disease (see “ Spinal Muscular Atrophy Variants ”). Most sporadic cases are related to de novo dominant mutations in various genes such as mitofusin 2 (MFN2), TPRV4, DYNC1H1, GDAP1, and NEFL. Mitochondrial genes have also been linked to the pathogenesis of infantile, primarily axonal, neuropathies.
Neuronal-axonal polyneuropathy associated with Werdnig-Hoffmann disease has been described in numerous reports. The infants studied generally have died in the first year of life with the clinical features of Werdnig-Hoffmann disease and with loss of axons noted at postmortem examination, in addition to the characteristic changes of anterior horn cells. Whether the degree of axonal loss is greater than that expected from the involvement of anterior horn cells of Werdnig-Hoffmann disease is difficult to judge from published data. However, of greater interest, disturbance of sensory nerve conduction velocity has been demonstrated, and axonal loss and glial infiltration have been reported in dorsal as well as ventral roots and in dorsal root ganglia at postmortem examination. Indeed, in one study, impairment of sensory nerve conduction velocities was said to be present in approximately 40% of cases of infantile SMA, but the diagnosis in these cases was not molecularly confirmed. In two severely affected sibships, associated with homozygous deletions of the SMN1 gene region, sensory as well as motor nerves were inexcitable on electrophysiological testing. In one sibship, the deletion was large and probably involved genes neighboring the SMN1 gene. However, in clinical practice SMA type 1 cases with sensory nerve or dorsal root ganglion cell involvement are extremely rare; one of the authors (BTD) has never seen such a case in 20 years of clinical and EMG practice.
Chronic Motor-Sensory Polyneuropathy: Subcellular
Various other neurological disorders, in which a specific subcellular structure or biochemical component thereof is affected, occasionally are recognizable in the first month of life and may have associated disease of peripheral nerve (see Box 32.6 ), including mitochondrial disease, cytoskeletal disorders (giant axonal neuropathy [intermediate filaments], infantile neuroaxonal dystrophy [neurofilaments]), and certain lysosomal disorders (Krabbe disease). Infantile neuroaxonal dystrophy is not a disorder of the neonatal period; the pathology of peripheral nerve has been defined. For practical purposes, Krabbe disease does not deserve serious consideration here because central nervous system phenomena usually dominate the clinical presentation and course (see Chapter 29 ). However, impaired motor nerve conduction velocities have been documented in the second month in Krabbe disease. Thus in this section, we review briefly only the neuropathic aspects of mitochondrial disease, as well as giant axonal neuropathy.
Mitochondrial Disease
Peripheral neuropathy is a common feature of mitochondrial disease . As discussed in Chapter 29 in relation to degenerative diseases of the central nervous system, mitochondrial disease, such as Leigh disease, may lead to hypotonia and weakness on the basis of disease above the lower motor neuron. However, in addition, some infants with mitochondrial disease have had electrophysiological and histological evidence of peripheral nerve involvement. In a well-studied series of 43 cases of congenital lactic acidosis related primarily to deficiency of pyruvate dehydrogenase activity or various elements of the electron transport chain (complexes I to IV, isolated or in combination), all exhibited neonatal hypotonia. Nerve conduction studies carried out later in infancy provided evidence of neuropathic disease in 42 of the 43. Approximately 70% exhibited signs indicative of both axonal and demyelinative disease. The EMG may reveal fibrillation potentials, findings supporting the concept of an ongoing denervating process. Nerve conduction velocities generally are reduced by approximately 15%, more consistent with axonal than myelin disease. No such studies of neonatal patients are available. Histological abnormalities of the nerve system have included evidence for axonal and myelin involvement. Several genes, such as SCO2, DGUOK, TK2, and others, have been found to be mutated in patients with infantile, primarily axonal neuropathies. The co-occurrence of neuropathy and CNS involvement, cardiomyopathy, hepatopathy, or ophthalmoparesis with or without lactic acidosis should raise the possibility of mitochondrial disease.
Giant Axonal Neuropathy
The unusual disorder of giant axonal neuropathy is characterized by onset after the first year of life of chronic, slowly progressive, primarily motor neuropathy. However, onset in the neonatal period with marked hypotonia, weakness, and areflexia has been reported. A nearly constant accompaniment is tightly curled, kinky, poorly pigmented scalp hair that may be apparent in the neonatal period (the hair abnormality is usually more obvious after the first months of life, and its absence is associated with a milder phenotype ). Nerve histology is striking: axons are greatly enlarged (giant axons) and are filled with disarrayed neurofilaments. Accumulation of filaments in Schwann cells may account for the associated demyelination. Involvement of neurons and oligodendroglia of the central nervous system may cause progressive cognitive and motor deficits and striking abnormality of cerebral white matter, especially on MRI scans. The demonstrations of disordered filaments in astrocytes and fibroblasts involving the principal proteins of intermediate filaments (i.e., glial fibrillary acidic protein [astrocytes] and vimentin [fibroblasts]) led to the suggestion that the disease represents a disturbance of organization of intermediate filaments. The genetic defect has been localized to chromosome 16q24, and the giant axonal neuropathy gene (GAN) encodes the protein gigaxonin. Gigaxonin appears to play an important role in the integrity of the cytoskeletal structure through interaction with a microtubule-associated protein.
Hereditary Sensory and Autonomic Neuropathies
Currently, hereditary sensory and autonomic neuropathies (HSAN) with clinical presentation in the neonatal period include at least four basic disorders: congenital insensitivity to pain (two types), a similar congenital neuropathy with anhidrosis, and familial dysautonomia (Riley-Day syndrome; see Box 32.6 ). The four disorders are inherited in an autosomal recessive manner and are classified as HSAN types II and V (congenital insensitivity to pain), III (familial dysautonomia), and IV (congenital insensitivity to pain with anhidrosis) in the classic classification system of Dyck and Ohta. Recently, the identification of genes associated with HSANs has led to a genotype-based classification system ( Table 32.6 ). Hereditary sensory radicular neuropathy type I usually exhibits clinical presentation in late childhood to early adulthood, but congenital onset has also been reported. Because autonomic phenomena are common in all these disorders, the term hereditary sensory autonomic neuropathies is preferred. These sensory disorders are discussed in this chapter on motor abnormalities, because hypotonia and areflexia are very common features.
TYPE | OTHER NAMES | INHERITANCE | GENES | OMIM |
---|---|---|---|---|
I | Hereditary sensory radicular neuropathy | Autosomal dominant | SPTLC1, SPLTC2 ATL1 RAB7 DNMT1 | 162400 613640 613708 600882 126375 |
IIA IIB IIC | Congenital insensitivity to pain | Autosomal recessive | WNK1 FAM134B KIF1A | 201300 613115 614213 |
III | FD/Riley-Day | Autosomal recessive | IKBKAP | 223900 |
IV | CIPA | Autosomal recessive | TrK A/NGF R | 256800 |
V | Congenital insensitivity to pain | Autosomal recessive | NGF B | 608654 |
HSAN Types II and V
The designation of congenital insensitivity to pain has been applied to a variety of cases of sensory neuropathy with onset in infancy and either slow or no progression of disease. Of these cases, patients with onset in the neonatal period consistently have exhibited the nonprogressive course. Because evaluation of sensory and autonomic nerve function, the prominent sites of involvement, is difficult in the newborn, the clinical presentation often suggests a motor disturbance because of the presence of hypotonia, areflexia, feeding disturbance , and occasionally, limb weakness. Gag and corneal reflexes are diminished. These deficits are presumably related to the disturbance of afferent input from muscle and the muscle spindle. With careful examination and with maturation of the infant, marked impairment of pain, touch, and temperature develops. Less consistently recorded have been disturbances of hearing, smell, and taste, although more careful testing probably would indicate that such disturbances are common. Although less commonly evaluated, such autonomic disturbances as defective lacrimation, impaired axonal flare after stimulation with intradermal histamine phosphate ( Fig. 32.14 ), and absence of fungiform papillae have been recorded. However, in HSAN type II sweating is normal, and cognitive function varies greatly. Infants with dysmorphic facies and with skeletal dysplasia have been described. In HSAN type V, cognitive impairment is not typical, and the degree of hypohidrosis is variable compared with anhidrosis in HSAN type IV. Mutations in four genes have been detected in patients with HSAN types II and V or congenital insensitivity to pain (see Table 32.6 ).

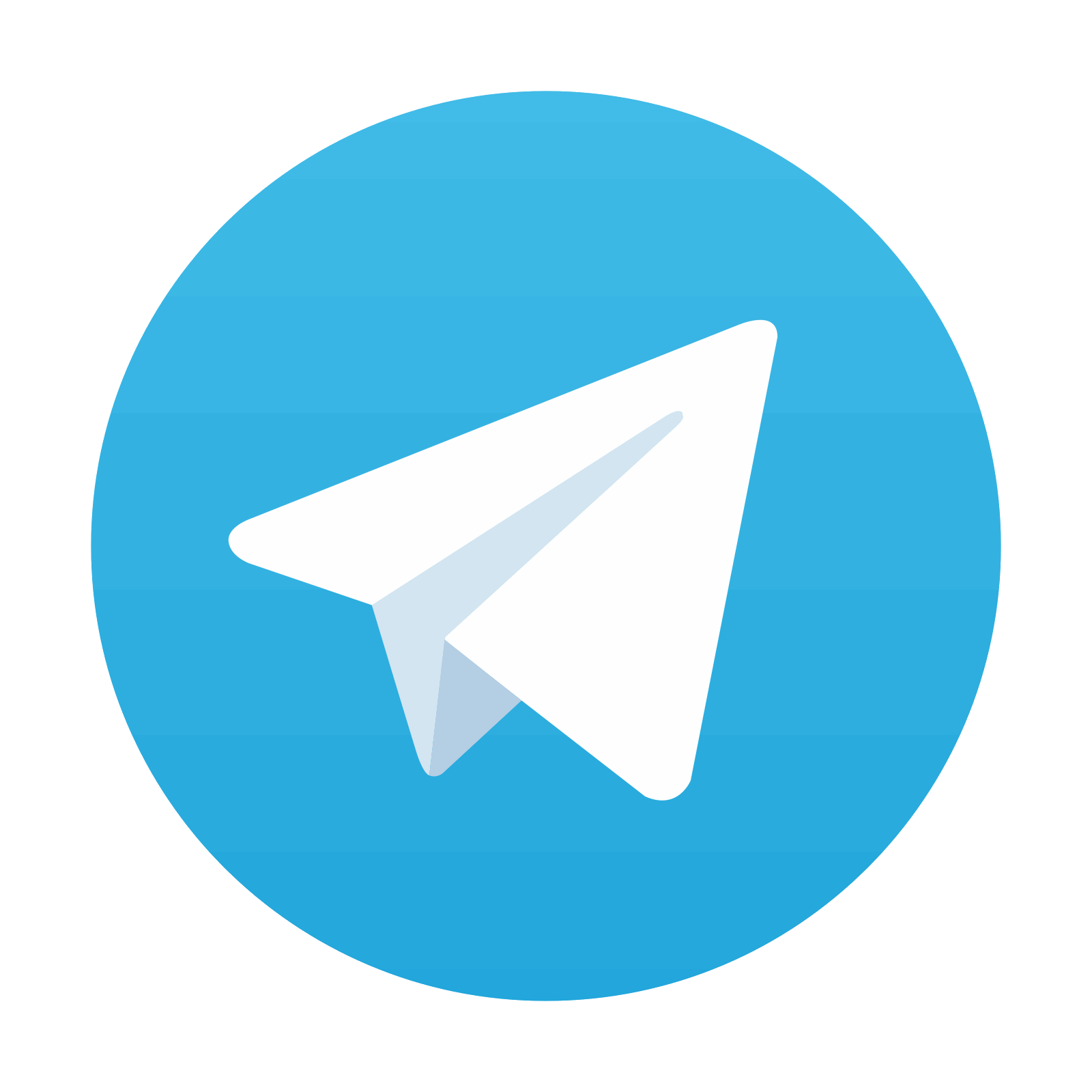
Stay updated, free articles. Join our Telegram channel

Full access? Get Clinical Tree
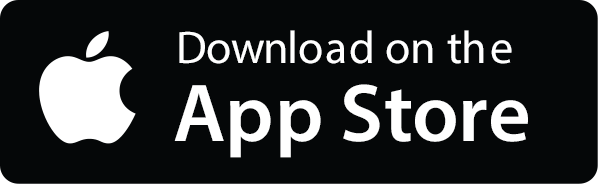
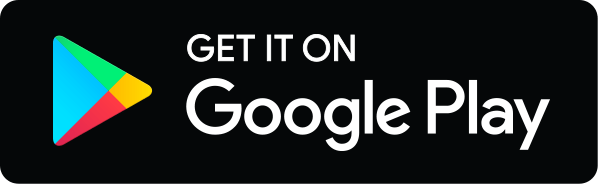
