Surgery of any kind carries risk of complications and, to date, there is no surgical intervention that is completely safe. As ever, the goal of the surgeon in this regard has been twofold – to prepare the patient for the possibility of complications, as a part of preoperative preparation and informed consent and, perhaps more importantly, to develop strategies to minimize or avoid complications to begin with. Since even the best orchestrated process may result in adverse events, the surgeon’s job is to know how to deal with these events once they occur so that negative end-results, measured by morbidity and mortality, are minimized.
When it comes to neuromodulation, an attractive feature of the field, along with reversibility, adjustability and testability of action, is its non-destructive nature. Most devices that are implanted to modulate activity of the nervous system, whether electrical or chemical neuromodulation, require relatively straightforward interventions – and yet the most difficult and, possibly most important part of the process has often been considered to be patient selection, as the proper selection of appropriate candidates greatly affects the short- and long-term outcome of neuromodulation procedures.
So how does one limit morbidity associated with placing neuromodulation device(s) in the human body? Mainly knowing of possible complications and procedural traps beforehand and taking steps to avoid them, goes a long way. This principle applies to all stages of the implantation procedure, from choice of best anesthesia and most appropriate neuromodulation equipment, to postoperative care of neuromodulation patients and troubleshooting during the follow-up period.
Listed below are the main groups of complications that will be discussed along with the recommended steps for their avoidance:
- 1.
Infection
- 2.
Hemorrhage
- 3.
Injury of nervous tissue
- 4.
Cerebrospinal fluid leak
- 5.
Placing the device into the wrong compartment
- 6.
Hardware migration
- 7.
Hardware erosion
- 8.
Hardware malfunction/fracture/disconnection
- 9.
Granuloma formation
- 10.
Other issues (radiographic safety, drug overdose, etc.).
The list of issues presented here is not all-inclusive. Other complications and side effects may occur at every stage of neuromodulation – and readers are encouraged to approach each patient and each intervention individually so special patient- and procedure-related peculiarities might be taken into account.
Infection
Although it would be expected for neuromodulation procedures to have a higher infection rate due to the presence of implanted devices and frequent need in keeping some part of the system externalized (like electrodes or extensions during neurostimulation trials and temporary epidural or intrathecal (IT) catheters used in screening for subsequent pump insertion), the incidence of infection may be equal or slightly higher than that observed in most surgical interventions. This may be, at least in part, explained by the elective nature of neuromodulation procedures. Since insertion of the neuromodulation device is usually done in a planned fashion, there is always a time interval that would allow the implanter to check the patient for ongoing infection or bacterial colonization, and to treat this infection to decrease the chances of the new device becoming colonized.
This would include examining a prospective patient for signs of infection and getting routine laboratory tests (peripheral white blood cell count, urinalysis) for active infection – and postponing surgery, including trial procedures, until any infection is treated adequately. In addition, in patients with known exposure or infection with methicillin-resistant Staphylococcus aureus (MRSA), it is generally recommended to obtain nasal swabs and treat those who are positive with chlorhexidine showers until the colonization and/or infection are eliminated. It is usually recommended to follow one’s institutional protocol for addressing MRSA infections if one exists.
In addition to the above measures, a recent comprehensive review of the literature and post-marketing surveillance data suggested controlling blood glucose levels in the perioperative period and stopping tobacco use for 1 month prior to surgery would further mitigate against infection . In general, the presence of healing surgical incisions or open wounds serves as a reason for postponing the elective neuromodulation surgery – with the possible exception of non-infected pressure sores in patients with severe spasticity who are scheduled for IT baclofen pump insertion, as these sores are unlikely to heal until spasticity is aggressively treated.
In terms of antibiotic prophylaxis, there is no consensus among neuromodulation implanters regarding the duration, route of administration and choice of antibiotic regimen. An almost universally accepted practice of administering preoperative antibiotics, usually second generation cephalosporins, within 2 hours of making a surgical incision represents more of a general standard that applies to most surgeries done in the operating room. We do not change such routines in our neuromodulation procedures and the choice of antibiotics is usually determined by the patient’s allergies and local hospital guidelines.
In order to sterilize the surgical field, we clean the surgical area with two sets of preparations containing iodine povacrylex (0.7% available iodine) and 74% isopropyl alcohol (DuraPrep, 3M, St Paul, MN) or, if no contact with nervous tissue is anticipated, 2% chlorhexidine gluconate/70% isopropyl alcohol formulation (ChloraPrep, CareFusion, San Diego, CA). We also routinely cover the entire surgical field with iodophor impregnated adhesive (Ioban, 3M) unless the patient is known to have an allergy to any of the mentioned components.
Postoperative antibiotic administration is probably the most controversial aspect of care as implanters do not agree with each other on the most rational, safe and effective way to approach infection prophylaxis. Specialists from the field of infectious diseases generally recommend keeping postoperative prophylactic antibiotic administration to a minimum, but most implanters give it either over 24 or 72 hours after device insertion, or for the duration of the trial. We are not aware of any scientific evidence that such practice reduces the risk of infection but, in our own practice, we continue prescribing oral antibiotics to all neuromodulation implant patients for the duration of the trial, when the part of implanted device is externalized, and several days after the permanent implantation. This way, patients with IT pumps and vagal nerve stimulators (VNS) receive a total of 5 days of postoperative antibiotics, those with deep brain stimulation (DBS) where trial or inter-stage interval lasts for 3–5 days receive 8–10 days of oral antibiotics, and those with spinal cord stimulators (SCS) and peripheral nerve stimulators (PNS) where trials are usually 7-days long end up getting a total of 12–14 days of oral antibiotics. With clear understanding of the lack of scientific support for this particular approach, we have been reluctant to change it since our incidence of infection after all neuromodulation procedures is well below 1%.
A recently suggested use of local antibiotic administration around the site of implantable pulse generators may be an attractive option for reduction of surgical infection but, so far, this technique has not gained expected popularity.
Handling of implantable hardware deserves separate mention – and here one may extrapolate recommendations for handling ventriculoperitoneal shunts published almost 20 years ago . These, among other things, include suggestions to do implant surgery as a first case of the day or as early during the day as possible, minimizing the number of people in the operating room, obtaining meticulous hemostasis and quality skin closure and, perhaps equally important, opening sterile packaging of the implanted hardware at the last moment .
One of the avenues that has not been explored so far would be to coat implantable components with antibiotic, similar to currently used shunt catheters, ventricular drains and venous access devices – and I am sure the device manufacturers have already considered (or are considering now) this as an additional approach further to reduce infection rates.
Once a device is implanted, infections do happen unpredictably – sometimes due to hematogenous spread of microorganisms from some other identifiable source, sometimes from local skin dehiscence or erosion over the implanted components, and sometimes from an unknown source in the absence of bacteremia. The recommended approach in either case would be to remove the implanted hardware and then re-implant it a few months later. Although multiple reports exist in the literature documenting successful salvage of infected neuromodulation hardware, our preference is to remove the device(s) altogether as systemic antibiotics do not penetrate all crevices and material interfaces of implants, and therefore eradication of infection is typically very challenging.
Hemorrhage
Hemorrhage may occur either during or after an operation – and since bleeding involving areas in or near the central nervous system may produce severe and sometimes irreversible neurological deficits, prevention of hemorrhage is extremely important in all neuromodulation procedures. There are several steps that have to be undertaken in order to limit hemorrhage-related morbidity; they include preoperative patient preparation, surgical planning, and procedural details aimed at obtaining adequate hemostasis.
In terms of patient preparation, it is extremely important to check each patient for the presence of coagulopathy or thrombocytopenia. This is usually accomplished by obtaining blood coagulation tests (prothrombin and partial thromboplastin times) and a complete blood count that includes platelets. Anticoagulants, such as heparin, enoxaparin and warfarin, should be stopped ahead of time – and the coagulation tests should be repeated and confirmed to be normal so there are no intraoperative surprises. We prefer stopping heparin 6 hours prior to elective surgery, low-molecular weight heparin (such as enoxaparin) 24 hours prior to surgery, and warfarin 5 days prior to surgery, sometimes bridging the patient with intravenous heparin for 2 days before surgery. In terms of antiplatelet agents, the existent guidelines for neuraxial procedures by the American Society of Regional Anesthesia and Pain Medicine postulate that these medications, including aspirin and other non-steroidal anti-inflammatory drugs (NSAIDs), thienopyridine derivatives (ticlopidine and clopidogrel) and platelet glycoprotein IIb/IIIa antagonists (abciximab, eptifibatide, tirofiban) exert diverse effects on platelet function and that pharmacologic differences make it impossible to extrapolate between the groups of drugs regarding the practice of neuraxial techniques . Based on analyzed current literature, these guidelines recommend stopping ticlopidine 2 weeks and clopidogrel 1 week before intervention. Platelet aggregation normalizes 48 hours after abciximab and 8 hours after eptifibatide and tirofiban discontinuation. They also state that aspirin and other NSAIDs do not create a level of risk that will interfere with neuraxial procedures . These guidelines, however, differ from usual surgical practice and we routinely ask our patients to stop aspirin 10 days prior to surgery, and ticlopidine and clopidogrel 2 weeks prior to surgery, including insertion of an IT catheter or pump, DBS, SCS, VNS or PNS electrodes or generators.
In order to reduce the risk of hemorrhage during the procedure, commonsense dictates a need to minimize manipulation with sharp instruments and needles during device insertion. However, it is very important to maintain adequate visualization of the surgical field and, sometimes, keeping incisions too small may be counterproductive as they would limit adequate access for hemostatic instruments. Hemostasis at open incisions is best accomplished with electrical coagulation and, in the beginning of surgery, before any metal implants are in place, monopolar coagulation with a Bovie device is perfectly appropriate (with the known need to avoid coagulation of skin and excessive tissue charring). Once the electrode, pumps or generators are inserted into the body, the use of monopolar coagulation is not recommended any more because of potential distant thermal and electrical effects. At this stage, bipolar coagulation should be used to stop bleeding.
In intraspinal procedures, it is not recommended to try to overcome resistance if it is encountered during electrode or catheter insertion forcefully. It is also not recommended to insert percutaneous electrodes at or through the levels where epidural space is filled with scar tissue as it makes surgery both difficult and dangerous. In these situations, where there was a previous laminectomy or laminotomy, there is a risk of not only hemorrhage but also possible dural penetration, particularly if the dura was violated during the original intervention.
For patients with previous surgery at the site of planned electrode location, it would be more prudent to consider placing paddle electrodes rather than trying to insert percutaneous ones. A procedure that seems to be particularly prone to cause epidural hemorrhage however, is a revision or removal of paddle-type SCS electrodes. For these patients, a somewhat longer postoperative observation may be needed as epidural hematomas may develop and/or become symptomatic as late as several days after the surgery.
For intracranial DBS electrode insertion, it appears that one of the ways to reduce the incidence of intracranial hemorrhage is to place electrodes through a gyrus – sulcal insertion has increased the incidence of cortical and subcortical hemorrhages . The incidence of ventricular hemorrhages also increases with transventricular trajectories and, if possible, the trajectory for electrode insertion should pass next to the ventricle rather than through it. Although male gender, age and diagnosis of Parkinson’s disease were found to be significant risk factors for intracranial hemorrhage, hypertension seems to be the most consistent risk factor . Additionally, the use of microelectrode recording (MER) in DBS targeting resulted in a higher incidence of intracranial bleeding and the highest incidence of hemorrhage was observed in those who had hypertension and MER. We use MER routinely for DBS surgery and, in order to reduce the incidence of intracranial hemorrhage, we try to minimize the number of electrode passes and pay special attention to blood pressure control during surgery with a dedicated anesthesiologist or nurse anesthetist monitoring patients undergoing DBS surgery and treating elevated blood pressure while avoiding sedation or agents that would adversely affect MER findings.
Needless to say, a decision to remove a neuromodulation device and/or evacuate a hematoma depends primarily on the degree of symptomatic impairment. Asymptomatic hemorrhages do not require surgical intervention but have to be monitored clinically and radiographically. Those who present with symptoms of cord compression have to be decompressed emergently. Management of intracranial hemorrhages depends on the size and location of the bleeding as well as on the risks and benefits of possible surgical intervention.
Injury of nervous tissue
The principle of neuromodulation involves non-destructive reversible modification of neural function through electrical, chemical or other means. The main advantages of surgical neuromodulation as opposed to non-surgical approaches, such as systemic medications or transcutaneous stimulation, are the high selectivity of action and significantly lower amount of electrical energy or chemical needed to achieve a similar response. This comes from direct proximity of surgical neuromodulation devices to their respective targets within the nervous system – the brain, spinal cord, peripheral and cranial nerves, and cerebrospinal fluid (CSF). Unfortunately, such proximity comes with a price – namely, the risk of inadvertent damage of the nervous system during implantation of neuromodulation components.
Of all instances of nervous tissue injury related to neuromodulation, most occur during the insertion procedure, and each particular location carries its own set of precautions and concerns. Only some of these concerns are listed here. In general, they include direct injury of the nerves, nerve roots, spinal cord, and brain, compression of the neural structures by the device or the scar around it, and long-term neural injury from the presence of the implanted device.
During insertion of SCS electrodes, care must be taken to avoid injury of the spinal cord and the nerve roots. Keeping the patient awake during these procedures solves only part of this concern since the mechanical irritation of nerve roots is usually perceived by the patient as sharp pain or acute discomfort – but spinal cord compression or even penetration are usually painless. Therefore, use of intraoperative fluoroscopy is recommended to keep the electrode in the middle third of the spinal canal away from the nerve roots. It is also recommended that one avoid using excessive force during electrode advancement as this may cause significant focal injury to the underlying spinal cord. Entering the epidural space with percutaneous electrodes is usually safer over the midline under the cephalad lamina or the base of the spinous process as the epidural space is usually largest in that location.
Dural penetration during implantation of percutaneous SCS electrodes is associated with multiple problems. CSF leak, spinal headaches and electrode migrations are discussed in the next sections, but additional risk involves potential direct injury of the spinal cord which may be unrecognized until the patient’s neurological function is tested or until the stimulator is turned on.
Compression of the spinal cord by a SCS electrode is less likely to occur with percutaneous electrodes and more common with paddle leads. Here, the important issue is to assess the size of the spinal canal and presence of any kind of stenosis, as well as clinically symptomatic or asymptomatic compression prior to the electrode implant. Interestingly enough, in those cases where a patient suffers neurological deficit after having a paddle electrode placed over the spinal cord in the presence of a compressing lesion, it is possible, in retrospect, to conceive that some or all of these symptoms that led to electrode insertion may have been indeed caused by that compressive phenomenon. Therefore, it should be mandatory to perform adequate preoperative imaging with MRI or CT myelography prior to placing paddle-type electrodes in order to avoid these situations.
Finding symptomatic or asymptomatic compression, or even subclinical stenosis, at the level of paddle insertion should not prevent SCS placement, but it may be prudent to combine electrode insertion with decompression through a laminectomy if the concern is established.
Another possible mechanism of spinal cord injury during paddle electrode insertion is related to inadequacy of dissecting tools used in the insertion process. Existing dissectors, sometimes called dural separators, carry a certain risk of spinal cord injury themselves as the material they are made of is either too hard (metal or hard plastic covered with thin layer of softer plastic or silicone) producing undue pressure either under the instrument tip deep under the lamina, or under the curvature of the tool directly in a laminectomy/laminotomy opening, or too soft (made of soft plastic attached to the rigid handle) to the point that this plastic tip buckles and folds over itself when resistance is encountered. With this in mind, new insertion tools are now being developed – putting most of the advancing force to the lateral recesses of the spinal canal rather than its middle portion, and shifting possibly injurious manipulation away from the spinal cord itself.
When an IT catheter is implanted, the injury to the nervous tissue may be caused by the needle through which the catheter is introduced or by the catheter itself. In order to reduce the possibility of unrecognized injury, use of fluoroscopy is strongly recommended as the midline of the lower lumbar spinal thecal sac has the most room between nerve roots. Also, the needle insertion site should be chosen in a way that the needle tip enters the spinal canal below the level of L1 (below the spinal cord conus). In addition, some implanters prefer keeping the patient awake during needle insertion so the patients’ complaints of discomfort, pain and their neurological function may guide the operator in the direction and depth of needle placement. We routinely perform catheter and pump implantation under general anesthesia however, as we feel the concern of cord or root injury is outweighed by a chance of a patient’s voluntary or involuntary movement during needle insertion causing the needle tip to injure the underlying neural structures.
In terms of catheter direction, we prefer advancing it in a cephalad direction as the pattern of CSF circulation inevitably creates a drug concentration gradient thereby supporting the old postulate that the catheter should end at the level where medication is needed. The argument of those who aim the catheter in a caudal direction is that the risk of cord injury with that approach is immensely lower and that the catheter tip granuloma (see below), should it form, will not be compressing the spinal cord. This approach has rationale, particularly when one considers the possibility of intramedullary catheter insertion . With this in mind, we suggest checking free CSF flow from the catheter – without active aspiration – at every stage of catheter insertion, including its advancement through the needle, anchoring, tunneling, etc. This approach ensures catheter patency and the subarachnoid tip, position of the catheter tip and eliminates the possibility of an intramedullary or ‘extrathecal’ catheter location. It must be mentioned, however, that a patient whose catheter was placed into the spinal cord by a less skilled implanter did not develop any symptoms from it immediately. Leg weakness came several days later, apparently as a result of intramedullary morphine infusion, and the symptoms resolved once the catheter was removed .
For DBS cases, brain injury other than from hemorrhage described earlier is quite rare, although non-hemorrhagic infarctions have been described in the past. Cortical and subcortical non-hemorrhagic lesions usually develop from superficial coagulation of the pial surface. In addition to this risk, coagulated pia also becomes harder and requires more force to advance cannulas and electrodes into the brain, therefore, we recommend using focal application of Gelfoam (Pfizer, New York, NY) with thrombin rather than electrocoagulaiton if minor cortical bleeding is encountered.
Postoperative imaging studies in DBS patients frequently reveal changes in T2 images and this may or may not be an asymptomatic finding. In our experience, development of changes on T2 and FLAIR MRI images often correlates with the number of passes during DBS implantation and, therefore, one should strive to minimize MER and DBS electrode passes as the brain may not be as ‘forgiving’ as was previously thought. Moreover, penetration of the brain with microelectrodes and DBS electrodes produces some transient neurological effects – sometimes temporarily improving their condition due to a ‘microlesioning’ effect , but making initial programming more difficult and possibly necessitating additional follow-up visits for reprogramming once this improvement wears off.
An injury to the peripheral nerve during PNS procedures may be more likely if the nerve is tethered to surrounding tissues due to scar from previous interventions. This was noted many years ago, when PNS devices were implanted through the open exposure procedure , but the concern remains even now when most PNS electrodes are inserted via the percutaneous approach. It is possible, at least theoretically, to reduce the chance of nerve injury if the nerve course is identified with ultrasound guidance. Live ultrasound may help track the insertion needle during PNS electrode placement in extremities , inguinal area , and occiput and this approach seems to be gaining popularity among implanters according to some recent unpublished surveys.
In addition to surgical injury, neural tissue damage may occur during long-term follow up of neuromodulation patients. Sometimes this is related to ongoing progression of their underlying degenerative or neoplastic disease, sometimes it is caused by a cord compression due to catheter-tip granuloma but, in rare cases, the cause of neurological dysfunction may be the scar tissue that formed around the electrode over the many years. A recently published description of two such cases illustrates such a possibility and underscores the relative youth of the entire neuromodulation field and our lack of knowledge regarding its long-term effects due to the fact that SCS and PNS have been around for less than 45 years and IT pumps, DBS and VNS were introduced even more recently.
Delayed neurological deficit was one of the main reasons for the old open approach for PNS to be abandoned or modified as the nerves targeted by PNS would exhibit perineural fibrosis or nerve ischemia , particularly if the electrode were wrapped around the nerve to avoid migration and assure better contact with tissue. In many cases, however, an open approach is taken because a neurolysis is first performed.
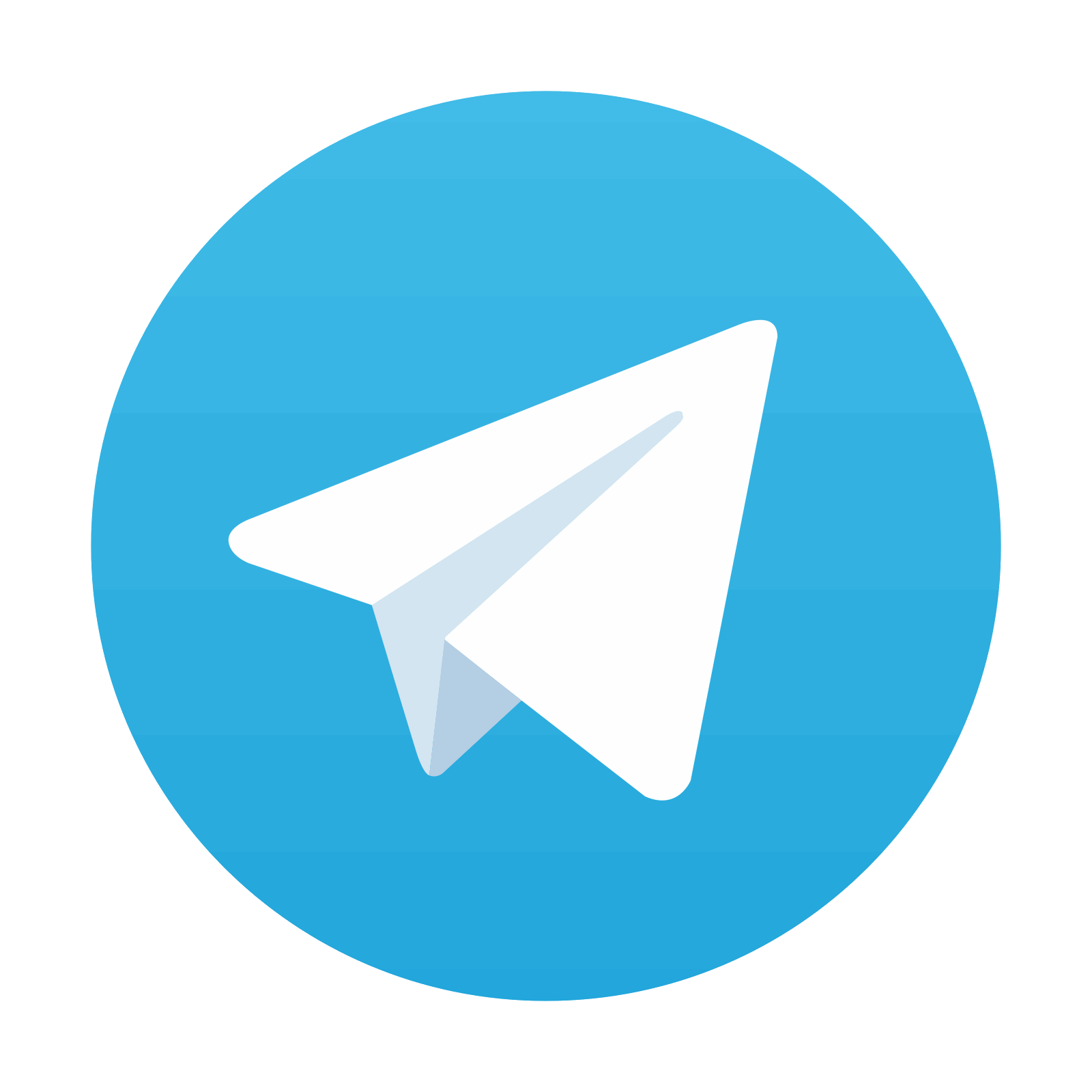
Stay updated, free articles. Join our Telegram channel

Full access? Get Clinical Tree
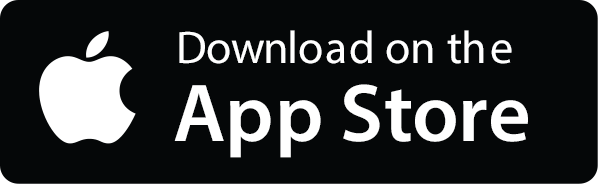
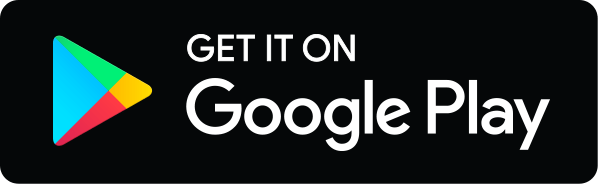