© Springer International Publishing Switzerland 2017
Gin S. Malhi, Marc Masson and Frank Bellivier (eds.)The Science and Practice of Lithium Therapy10.1007/978-3-319-45923-3_88. Lithium Response Variability (Pharmacogenomics Studies)
(1)
Mood Disorders Unit, Division of Psychiatric Specialties, Department of Mental Health and Psychiatry, University Hospitals of Geneva, Rue de Lausanne 20bis, CH-1201 Geneva, Switzerland
(2)
TRE Program, Division of Psychiatric Specialties, Department of Mental Health and Psychiatry, University Hospitals of Geneva, Rue de Lausanne 20bis, CH- 1201 Geneva, Switzerland
(3)
Division of Psychiatric Specialties, Department of Mental Health and Psychiatry, University Hospitals of Geneva, and Faculty of Medicine, University of Geneva, Rue de Lusanne 20bis, Geneva, 1201, Switzerland
Abstract
Lithium is considered to be the prototypical mood stabilizer. Naturalistic studies indicate that about one third of bipolar patients show a good or very good clinical response to lithium therapy. However, based on clinical evaluation, it remains difficult to reliably predict which patients are more likely to benefit from lithium therapy. In the era of personalized medicine, pharmacogenetics offers the hope that genetic markers could provide reliable predictors of individual responses to lithium therapy. As reviewed in this chapter, pharmacogenetic studies performed over the past two decades have unfortunately failed to produce predictive markers for lithium response that are meaningful for clinical practice. Lack of progress may be due to a variety of factors, including small sample sizes, clinical heterogeneity and variable definitions of lithium response versus non-response phenotypes. To circumvent these difficulties, large-scale international collaborative consortia are emerging, thus opening the possibility of performing genome-wide association studies on well-defined lithium-response phenotypes.
Keywords
PharmacogeneticsPharmacogenomicsLithiumGenome-wide association studiesPersonalized medicineClinical predictorsKey Points
Reliable genetic predictors of individual responses to lithium therapy would be of great benefit to both patients and clinicians.
Current findings based on small sample sizes and candidate-gene approaches lack robust replication.
Large-scale international collaborative consortia are needed to replicate positive associations and to identify novel genetic targets.
8.1 Introduction
Lithium is considered to be the prototypical mood stabilizer. It has been shown to treat and prevent both manic and depressive phases of bipolar disorder (Fountoulakis et al. 2012; Fountoulakis and Vieta 2008; Suppes et al. 2005; Yatham et al. 2013a, b). Although lithium is a first-line treatment in bipolar disorder (Grandjean and Aubry 2009; Nivoli et al. 2011, 2012; Malhi et al. 2015), only a subset of patients shows a positive therapeutic response to it (Manchia et al. 2013). Naturalistic studies indicate that about one third of bipolar patients may achieve complete remission with lithium therapy (Garnham et al. 2007; Maj et al. 1998; Prien et al. 1974; Rybakowski et al. 2001; Solomon et al. 1995). However, the fraction of bipolar patients responding to lithium is likely to vary in a given clinical sample depending on whether bipolar II and subthreshold bipolar disorders are included. Furthermore, the definition of what constitutes a meaningful clinical therapeutic response varies in the literature (Geoffroy et al. 2014). At the clinical level, a number of variables have been associated with a poor lithium response (Grof et al. 1993). Generally, it has been shown that bipolar patients displaying an early onset of the disorder, an episodic clinical course, rapid cycling and mixed manic states are poor lithium responders (Grof et al. 1993; Strober et al. 1988). There is also some evidence that a positive therapeutic lithium response could run in families (Alda 2001). Bipolar patients responding to lithium appear to have a greater likelihood of having family relatives with a bipolar disorder responsive to lithium, thus suggesting that lithium responders may be genetically distinct (Aronoff and Epstein 1970; Duffy et al. 2007; Grof et al. 2002).
Determining the genetic factors that predict lithium responsiveness is of considerable interest, as it would facilitate the personalization of pharmacological treatment strategies for bipolar patients (Can et al. 2014; Geoffroy et al. 2014). Ideally, this would enable clinicians to maximize the benefits of lithium therapy in genetically prone responders and to minimize lithium side effects in genetically determined nonresponders by instead proposing to these patients alternative classes of mood stabilizers. The field of lithium pharmacogenomics is still in its infancy, and results are largely based on candidate-gene approaches performed on small sample sizes. Candidate genes have mainly been selected based on a priori hypotheses informed by preclinical data describing the molecular pathways modulated by lithium. In this chapter we review the available data on the pharmacogenetics of lithium response in bipolar patients and proceed by evaluating the evidence obtained in candidate genes involved in lithium-regulated signalling pathways.
8.2 The Inositol Pathway
Preclinical work over the past decades has led to the identification of a large number of molecular pathways modulated by therapeutic levels of lithium (0.6–1.2 mM) (Quiroz et al. 2004). Among these pathways, the impact of lithium on the regulation of inositol metabolism has been supported by a great deal of research (Manji et al. 1999; Quiroz et al. 2004; Williams et al. 2002). Activation of the inositol pathway classically occurs through G-protein-coupled receptors (GPCR) coupled to the enzyme phospholipase C (PLC). PLC activation leads to the hydrolysis of phosphatidylinositol 4,5-biphosphate (PIP2) into two important signalling molecules: inositol 1,4,5-triphosphate (IP3) and diacylglycerol (DAG). IP3 has multiple intracellular signalling properties, such as the induction of calcium release from the endoplasmic reticulum, whereas DAG activates downstream targets such as protein kinase C (PKC). IP3 is used to produce myo-inositol, which is recycled into PIP2 by phosphodiesterase enzymes, among which are inositol monophosphatase (IMPase) and inositol polyphosphate 1-phosphatase (IPPase).
Lithium is a positively charged ion that enters the cell and competes with the magnesium ion, which is used as a cofactor for enzymes such as IMPase and IPPase. By competing with magnesium, lithium is thought to inhibit IMPase and IPPase activity, thus decreasing the recycling of myo-inositol into PIP2 and leading to a state of relative inositol depletion. Evidence for this hypothesis is robust in rodent preclinical models following acute and chronic lithium administration (Allison et al. 1980; Allison and Stewart 1971; Lubrich et al. 1997; Sherman et al. 1981). In humans, decreased brain myo-inositol levels have been observed after chronic lithium therapy (Moore et al. 1999), whereas other studies have found no effect or even increased levels of myo-inositol after lithium therapy (Forester et al. 2008; Patel et al. 2006; Silverstone and McGrath 2009). Although there is a large amount of data indicating that lithium regulates the inositol pathway, there is only scant evidence in humans that differential clinical response to lithium therapy is determined by variations in genes belonging to the inositol pathway. In a small pilot sample of bipolar patients, a single nucleotide polymorphism (SNP) in the INPP1 gene coding for the inositol polyphosphate 1-phosphatase enzyme (C973A) was found to be associated with lithium responsiveness (Steen et al. 1998). However, replication for this association failed in a second cohort tested in this initial report (Steen et al. 1998) and was not replicated in an independent study (Michelon et al. 2006). Apart from these initial findings, an interaction between a SNP in INPP1 and post-traumatic stress disorder in lithium responders with bipolar disorder has been reported (Bremer et al. 2007). In contrast to the INPP1 gene, no association has been found between lithium response and genetic variation in other genes regulating the inositol pathway, such as the inositol monophosphatase IMPA2 and IMPA1 genes (Bremer et al. 2007; Michelon et al. 2006; Sjoholt et al. 2004), the phospholipase C-gamma 1 (PLCG1) gene (Ftouhi-Paquin et al. 2001) and the diacylglycerol kinase eta (DGKH) gene (Manchia et al. 2009b).
Taken together, available data indicate that genetic variation in genes involved in the inositol pathway does not appear to determine major differences in therapeutic responses to lithium. However, only a fraction of genetic variability in inositol-related genes has been tested, and large-scale studies are clearly needed to further explore additional targets in the inositol pathway and to potentially validate initial positive findings in the INPP1 gene.
8.3 Glycogen Synthase Kinase 3 and Circadian-Cycle-Related Genes
Glycogen synthase kinase 3 (GSK-3) is a constitutively active serine/threonine protein kinase that was initially found to deactivate glycogen synthase (Gould 2006). Lithium directly inhibits GSK-3 through competition with magnesium ions (Ryves and Harwood 2001) and indirectly through phosphorylation of N-terminal serines (Chalecka-Franaszek and Chuang 1999; Gould 2006; Gould and Manji 2005; Gurvich and Klein 2002). Given that GSK-3 regulates a variety of molecular pathways, including the Wnt and AKT signalling pathways (Chalecka-Franaszek and Chuang 1999; De Sarno et al. 2002), there has been a great deal of interest in understanding the molecular consequences of GSK-3 lithium-induced inhibition on neuronal function, signalling pathways and behavioural rodent phenotypes such as manic-like hyperlocomotion and depression-like behaviours (Beaulieu et al. 2004; Gould 2006; Gould et al. 2004; Polter et al. 2010; Prickaerts et al. 2006). Taken collectively, rodent studies support the role of GSK-3 in mediating lithium-induced behavioural responses (Gould et al. 2004), thus suggesting that genetic variants in GSK–3 could be associated with differential lithium responsiveness in bipolar patients. An initial study reported that carriers of a rare functional variation in the promoter region of the beta isoform of GSK–3 displayed a better respond to lithium therapy compared to carriers of a more common SNP allele (Benedetti et al. 2005). Interestingly, this finding could be subsequently replicated in an independent study (Adli et al. 2007). In addition to these two positive studies, an association between a SNP in GSK–3beta gene and lithium response in patients with bipolar disorder and post-traumatic stress disorder has been reported (Bremer et al. 2007). In contrast, no clear associations between polymorphisms in GSK–3beta were reported in other studies (McCarthy et al. 2011; Szczepankiewicz et al. 2006), thus casting doubts on the robustness of previous studies. Bipolar disorder is associated with dysregulation of circadian rhythms, and the NR1D1 gene, encoding for the orphan nuclear receptor REV-ERBalpha, is a negative component of the circadian clock. Interestingly, REV-ERBalpha is a phosphorylation target of GSK-3beta, and its proteasomal degradation is regulated by lithium in cell cultures (Yin et al. 2006). Associations between genetic variation in NR1D1 and lithium response have led to positive (Campos-de-Sousa et al. 2010; McCarthy et al. 2011) and negative results (Manchia et al. 2009b). Interestingly, genetic variations in GSK–3beta and NR1D1 were found to act additively to predict response to lithium (McCarthy et al. 2011), suggesting that this pathway could be relevant for future studies. Taken collectively, published data are suggestive of a role for genetic variation in GSK-3beta in determining lithium response, but the robustness of these findings needs to be further confirmed in larger samples.
8.4 The Brain-Derived Neurotropic Factor (BDNF) Pathway
In both neuronal cultures in vitro and rodent models in vivo, lithium has been shown to be neuroprotective and to prevent the damaging effects of excitatory neurotoxicity and ischaemic insults (Chiu et al. 2013). Several molecular pathways could mediate these effects, including activation of pro-survival pathways such as the neurotrophin BDNF signalling pathway. For example, it has been shown that chronic lithium administration increases BDNF in brain regions such as the frontal cortex and the hippocampus (Angelucci et al. 2003; Fukumoto et al. 2001; Omata et al. 2008). Given the role of lithium in promoting BDNF signalling, genetic studies have focused on genetic variation in the BDNF gene and lithium responsiveness. The commonly studied BDNF val66met functional polymorphism has been investigated in several studies with mixed results. Initial studies reported an association between the BDNF val66met polymorphism and lithium responsiveness (Dmitrzak-Weglarz et al. 2008; Rybakowski et al. 2005b, 2007); however other studies failed to replicate these positive findings (Masui et al. 2006; Michelon et al. 2006). Some studies have focused on genetic variants in the NTRK2 gene, which encodes for the TrkB protein, a key receptor binding to BDNF. Two independent studies have reported a significant association between NTRK2 variants and lithium responsiveness (Bremer et al. 2007); however this result was not replicated in another sample (Dmitrzak-Weglarz et al. 2008; Wang et al. 2013). Taken collectively, there is some suggestive evidence in the field that genetic variation in BDNF signalling could account for differential clinical responsiveness to lithium, but these findings clearly need further validation in larger sample sizes.
8.5 The Monoamine Pathways
A large class of antidepressants exerts their action by selectively blocking the serotonin transporter, a key molecule regulating a variety of cellular and physiological processes in the brain and periphery (Murphy and Lesch 2008). Furthermore, manipulation of the serotonin system through tryptophan depletion induces depressive-related symptoms, thus suggesting a possible causal link between serotonin-related dysregulation and mood disorders (Smith et al. 1997; Cowen 2008). Finally, there is evidence of altered brain serotonin function in unmedicated depressed patients (Sharp and Cowen 2011; Cowen 2008). Given these arguments, mood disorders have traditionally been associated with disturbances in serotonin-related pathways (Manji et al. 2001). In the context of resistant depression, lithium has been used as an efficient augmentation therapy in association with antidepressants (Bauer et al. 2010; de Montigny et al. 1983). This has led to the hypothesis that lithium could exert these antidepressive properties by modulating the serotonin system. Studies in rodents indicate that lithium can increase serotonin levels in several brain regions (Baptista et al. 1990; Mork 1998). Furthermore, preclinical studies indicate that coadministration of lithium and antidepressants produces a synergetic effect on serotonergic transmission (Muraki et al. 2001; Okamoto et al. 1996; Wegener et al. 2003). Taken together, these preclinical data suggest that lithium could possibly exert some of its clinical efficacy through the modulation of the serotonin system, and this opens the possibility that genetic variation in serotonin-related genes could be involved in differential lithium responsiveness in bipolar patients.
In the field of mood disorders, the role of a functional genetic variant in the promoter region of the serotonin transporter (5–HTTLPR) has received great attention. A large body of research indicates that the short allele of 5–HTTLPR (s-allele) interacts with early-life adversity to increase the risk of depression (Caspi et al. 2003; Karg et al. 2011; Uher et al. 2011). In addition, s-allele carriers display evidence of altered cortisol response to stress (Chen et al. 2014; Mueller et al. 2011; Way and Taylor 2010) or to tryptophan depletion (Cerit et al. 2013) as well as increased amygdala activation to negative emotional stimuli (Munafo et al. 2008). In addition, s-allele carriers from Caucasian, but not from Asian, origin appear to be less responsive to the antidepressant effects of selective serotonin reuptake inhibitors (Pollock et al. 2000; Porcelli et al. 2012; Smeraldi et al. 1998; Yoshida et al. 2002). In a similar manner, earlier studies have suggested that s-allele carriers could show decreased therapeutic responsiveness to lithium. Indeed homozygote carriers of the s-allele presenting either unipolar depression or bipolar disorder showed a decreased prophylactic response to lithium when prospectively assessed during more than 50 months (Serretti et al. 2001). An association between s-allele genotype and lithium non-response was replicated in an independent study (Rybakowski et al. 2005a). In contrast, opposite results were obtained in another study where s-allele carriers showed a better therapeutic response to lithium therapy compared to l-allele carriers (Serretti et al. 2004). These contradictory results have been further obscured by the fact that several studies revealed no association between lithium responsiveness and the 5–HTTLPR genotype (Manchia et al. 2009a; Michelon et al. 2006). Thus, as described above with other genes investigated, the literature on 5–HTTLPR genotype and lithium responsiveness is mixed and still inconclusive.
In addition to studies focused on the 5–HTTLPR, lithium responsiveness in relationship to genetic variation in serotonin receptors has been investigated. Polymorphisms in several serotonin receptors, such as the 5–HT 2A , 5–HT 2B , and 5–HT 2C , were investigated in bipolar patients, and no variants were associated with lithium outcome in several independent studies (Dmitrzak-Weglarz et al. 2005; Manchia et al. 2009a; Serretti et al. 2000). Finally, it should be mentioned that lithium response in relation to genetic variation in other monoamine systems, such as the dopamine system, has been investigated. Similarly to the serotonin system, no clear association between genetic polymorphisms in different dopamine-related genes and lithium responsiveness has emerged. An initial report suggesting an association between a polymorphism in the DRD1 gene coding for the dopamine receptor 1 and decreased responsiveness to lithium prophylaxis (Rybakowski et al. 2009) was not replicated in a subsequent study (Manchia et al. 2009a). No clear evidence for an association between therapeutic lithium response and genetic variation has been found in other monoamine-related genes, such as the tryptophan hydroxylase gene, the serotonin rate-limiting enzyme in serotonin synthesis (Serretti et al. 1999b); the DRD2, DRD3 and DRD4 genes coding for dopamine receptors (Manchia et al. 2009a; Serretti et al. 1998, 1999a); the dopamine transporter (DAT) gene (Manchia et al. 2009a); the catechol-O-methyltransferase (COMT) gene (Serretti et al. 2002); and the monoamine oxidase A (MAO-A) gene (Serretti et al. 2002; Turecki et al. 1999). Again, it should be mentioned that monoamine systems are highly complex and comprise many receptor subtypes with multiple splice variants. Much work thus remains to be done to map individual genetic variability in monoamine systems, thus leaving open the possibility that differential responses to the beneficial properties of lithium could still be partially determined by monoamine-related genetic differences.
8.6 Glutamate and GABA Pathways
A balance between inhibitory GABAergic and excitatory glutamatergic neurotransmissions is key for the proper function of neuronal circuits. The topic of antidepressant properties of drugs acting on the glutamatergic system has gained considerable interest recently with the observation that molecules targeting NMDA receptors, such as ketamine, can exert rapid antidepressant effects in unipolar and bipolar depression (Zarate et al. 2006, 2010, 2013). A rationale for a link between lithium and the glutamatergic system is provided by the observation that lithium may modulate NMDA/AMPA-mediated neurotransmission by modifying membrane expression of AMPA receptors in the hippocampus (Du et al. 2008; Gould et al. 2008). An association between increased risk for bipolar disorder and specific genetic polymorphisms in subunits of the NMDA receptors, such as the GRIN2B gene, has been reported, thus suggesting that this gene could be involved in lithium responsiveness (Martucci et al. 2006). However, no correlations between genetic variation in GRIN2B and lithium therapeutic responses were observed in a cohort of bipolar patients treated with lithium over 5 years (Szczepankiewicz et al. 2009b). Similarly, no evidence was found for an association between lithium response and polymorphisms in protein kinase FYN, which is involved in phosphorylating NMDA receptor subunits (Szczepankiewicz et al. 2009a). Suggestive evidence for an association between lithium response and genetic variation has been reported in GRIA2, a gene coding for AMPA receptor subunit (Perlis et al. 2009), and CACNG2, a gene coding for a voltage-dependent calcium channel involved in AMPA receptor trafficking (Silberberg et al. 2008). These studies clearly warrant further confirmation in independent samples. Regarding GABAergic pathways, candidate-gene approaches failed to demonstrate an association between lithium response and polymorphisms in GABA-A receptor subunits including GABRA3, GABRA5 and GABRB3 genes (Duffy et al. 2000). More recently, a study performed on bipolar patients of Han Chinese descent revealed a highly significant association (p = 7.07 × 10−50) between lithium response and two SNPs located in the glutamate decarboxylase-like protein 1 (GADL1) (Chen et al. 2014). The function of this protein is currently unknown, but it shares sequence homologies with glutamate decarboxylase isoforms, which normally catalyse the conversion of GABA to glutamate. However, a recent attempt to validate this finding in an Asian subsample of patients from the Consortium of Lithium Genetics (ConLiGen) failed (Hou et al. 2014). Future studies should thus be performed to further investigate the role of GADL1 in lithium responsiveness and to provide a functional role for this protein in neuronal circuit function. Overall, convincing evidence is lacking for an association between lithium response and genes involved in glutamate or GABAergic signalling. However, given the complexity of these pathways, much work remains to be performed in these signalling systems.
8.7 Summary
Attempts in the preceding two decades to associate clinical lithium response with genetic variation in candidate genes involved in key signalling pathways modulated by lithium have failed to provide clinically meaningful results. As generally observed with genetic-association studies performed on small sample sizes, replication has been difficult to achieve, thus casting valid doubts on the robustness of positive published data. There is thus a critical need for larger sample sizes of bipolar patients with well-characterized lithium-response phenotypes in order to validate initial findings. Recently the field has moved forward towards achieving this goal with the emergence of large-scale international collaborative consortia. A genome-wide association study (GWAS) has been performed on more than 24,000 bipolar patients and controls (Muhleisen et al. 2014). Results have revealed a small number of hits reaching genome-wide statistical significance and, more generally, failed to validate candidate risk genes previously investigated before the era of GWAS studies. Interestingly, bipolar risk genes revealed in this recent study, such as ANK3, CACNA1C and ODZ4 genes, were also identified in previous GWAS published in the field (Cross-Disorder Group of the Psychiatric Genomics Consortium and Genetic Risk Outcome of Psychosis (GROUP) Consortium 2013; Moskvina et al. 2009; Psychiatric GWAS Consortium Bipolar Disorder Working Group 2011; Schizophrenia Psychiatric Genome-Wide Association Study (GWAS) Consortium 2011).
With the aim of moving on to large-scale GWAS approaches, an international Consortium of Lithium Genetics (www.ConLiGen.org) emerged in 2009, enabling the collection of a sample size of more than 3,000 bipolar patients under lithium therapy and well characterized for their response to lithium (Manchia et al. 2013; Schulze et al. 2010). Given that variability in definitions of lithium response is an important confounding factor in pharmacogenetic studies, bipolar patients in the ConLiGen consortium are assessed using common quantitative scales, such as the Alda scale (Manchia et al. 2013).
In conclusion, there is hope that GWAS performed on larger sample sizes will help to determine whether some of the positive findings that have emerged using candidate-gene approaches can be replicated and whether novel targets can be identified. Overall, the hope is that a new generation of pharmacogenomic approaches will produce reliable and clinically meaningful genetic markers in order to predict which patients will benefit most from lithium prophylactic therapy.
References
Adli M, Hollinde DL, Stamm T, Wiethoff K, Tsahuridu M, Kirchheiner J et al (2007) Response to lithium augmentation in depression is associated with the glycogen synthase kinase 3-beta -50T/C single nucleotide polymorphism. Biol Psychiatry 62(11):1295–1302. doi:10.1016/j.biopsych.2007.03.023 PubMed
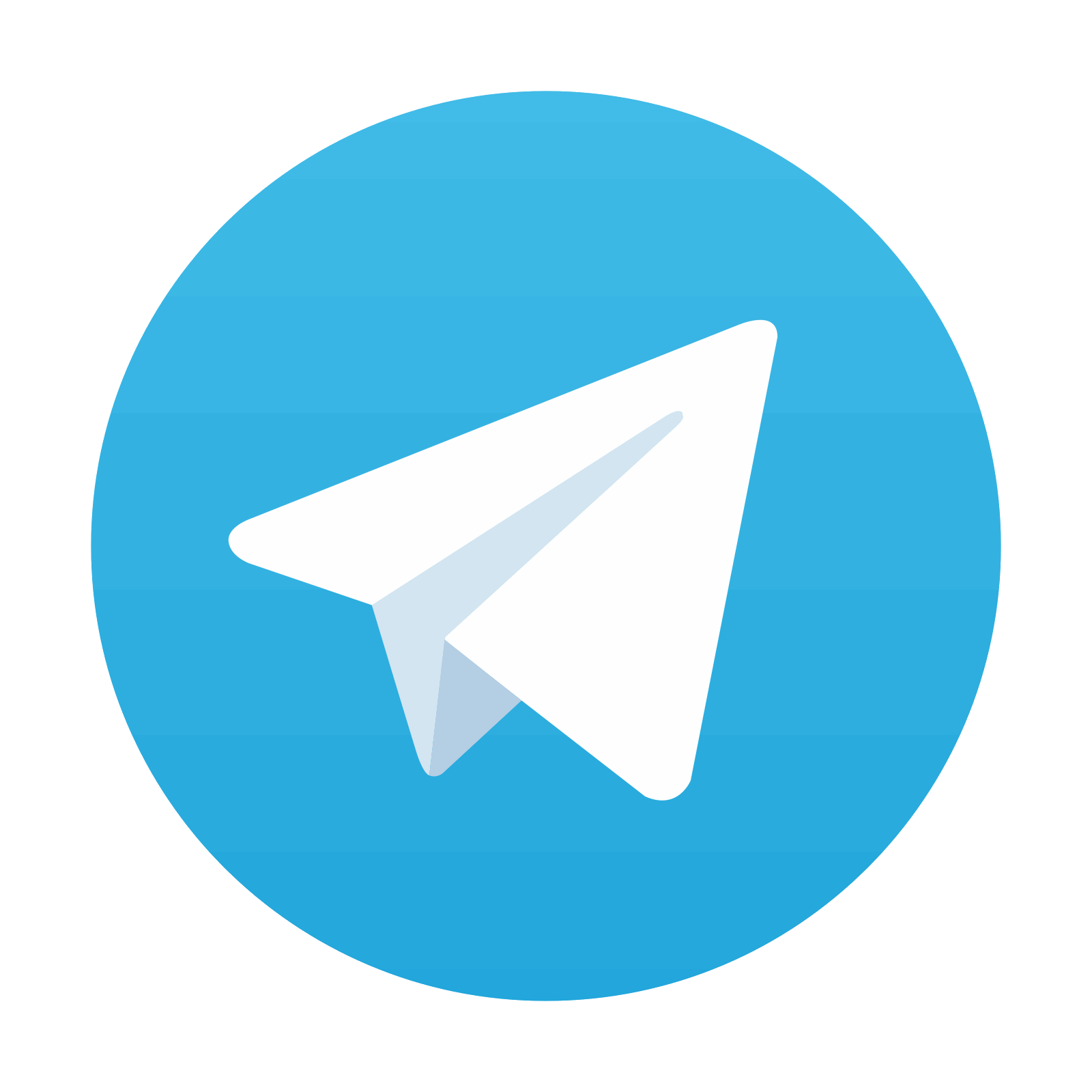
Stay updated, free articles. Join our Telegram channel

Full access? Get Clinical Tree
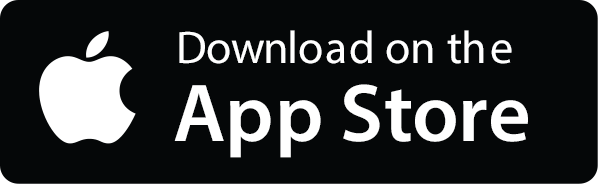
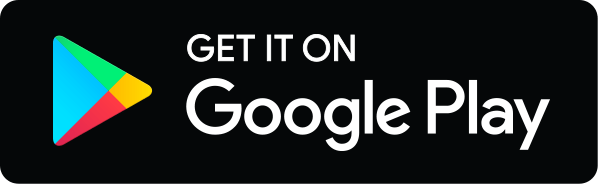
