Fig. 4.1
Engel classification of postoperative seizure outcome

Fig. 4.2
ILAE classification system of postoperative seizure outcome. Seizure outcome class determined for each year at annual intervals after the date of surgery. *Differentiates from Class 1 which refers to seizure freedom within the last year of follow-up
For these reasons, the ILAE proposed a classification system in 2001 [2] that addressed the shortcomings of the Engel system. Specifically, the introduction of “seizure days,” referring to the incidence of at least one seizure in a 24-h period is thought to be more clinically meaningful and addresses patients who occasionally have clusters of seizures or episodes of status epilepticus.
To utilize the ILAE system correctly for surgical patients, baseline seizure days for the 12-month period prior to surgery must be known. This makes the ILAE system applicable to individuals and populations both on a year-by-year basis and cumulatively to the last documented outcome. However, ILAE outcome determination for retrospective studies is not possible if baseline data is unavailable. This latter point may explain why the Engel system continues to be popular in epilepsy surgery studies as most published series are retrospective and baseline data for the preceding 12-month period is often unknown. Further, there may be a relatively high frequency of seizures in young children in whom benefit may not be captured utilizing the ILAE scheme.
Only one published study has compared the ILAE and Engel classification systems [3]. Independent interpreters assessed a mixed cohort of 76 patients that included 25 children and both systems were found to have high inter-rater agreement.
There currently is no pediatric-specific outcome scale and reliance on either the Engel or ILAE classification systems is acceptable [4]. However, the Engel system is the most widely employed outcome measure and has adequate inter-rater reliability. The Engel system is, therefore, utilized throughout this chapter to maintain consistency across interpretation of data unless referenced studies specifically applied the ILAE classification system.
The Engel and ILAE classification systems address only seizure outcome and do not assess psychosocial, behavioral, cognitive, or vocational development, all vital to gauging the utility of epilepsy surgery [4, 5]. Discussion of these measures and their relationship to quality of life is beyond the scope of this chapter and are discussed in detail in other chapters. Further, the system does not specify or include duration of follow-up at the time of reporting, meaning direct comparison between studies is not possible.
Predictors of Seizure Outcome
Predicting seizure freedom after epilepsy surgery in childhood is imperative for guiding candidate selection and family counseling. This can be a challenging task as children have unique clinical, developmental, and pathological variables that make comparisons with adults difficult [6]. Furthermore, factors that influence surgical outcome in adults such as temporal lobe location and presence of a gross structural lesion are less important in childhood [6]. An understanding of both positive and negative predictors of seizure freedom greatly assists in candidate selection. Table 4.1 presents various clinical and peri-operative factors associated with seizure outcome.
Table 4.1
Factors that result in favorable and unfavorable outcomes or have no effect on postsurgical seizure freedom in all types of epilepsy in children
Presurgical | Surgical | Postsurgical | |
---|---|---|---|
Favorable outcome | Abnormal MRI Presence of MRI lesion Focal interictal discharges Regionalized ictal discharges MEG clusters within ictal onset zone | Completeness of resection Temporal resections | Focal cortical dysplasia |
No effect on outcome | Sex Age at surgery Duration of epilepsy Seizure frequency Seizure type (partial or generalized) Cognitive impairment Normal/non-focal MRI PET SPECT | Tuberous sclerosis Low grade tumor | |
Unfavorable outcome | No MEG Clusters within ictal onset zone Multiple MEG clusters overlapping the ictal onset zone | Interictal discharges within/near resection cavity Interictal discharges lateralized to side of resection Nonspecific histopathology Postencephalitic histopathology |
Presurgical Predictors of Seizure Freedom
Patient Demographics
Most preoperative clinical features do not predict seizure freedom [6–9]. There is no reported association of sex, age at surgery, duration of epilepsy, seizure frequency, seizure type (partial or secondarily generalized) or presence of cognitive impairment with postsurgical seizure status [6–8]. In contrast, a single preoperative seizure semiology is an important predictor in MR-negative cases [8, 10] and the absence of generalized seizures also correlates with favorable outcome in TLE and ETLE [11, 12]. The effect of age at onset of epilepsy and subsequent postsurgical seizure freedom is often confounded by the underlying substrate. For instance, surgical success is less likely in patients who present in early life with focal cortical dysplasia possibly due to associated atypical widespread epileptogenic networks that are established prenatally or in early postnatal life [13, 14].
Presence of MRI Lesion
Data on investigative predictors of outcome are limited. The presence of a discrete lesion on MR imaging has been shown consistently to correlate with a favorable seizure outcome [11, 12, 15]. MR abnormalities provide anatomical landmarks for intracranial electrode placement, assist in determining resection margins and confirm localizing findings derived from other presurgical investigations. These factors all contribute to completeness of resection and surgical success [6]. Seizures associated with highly demarcated MRI lesions such as hamartomas, vascular malformations or developmental tumors are often alleviated by lesionectomy alone. In contrast, non-lesional or subtle pathological substrates such as infection, trauma, or degenerative disorders are typically associated with a more diffuse insult with potential secondary areas of epileptogenesis that may activate after initial resection [12].
Interictal Discharges
Focal interictal discharges on scalp and video EEG positively predict seizure freedom while ictal patterns more often only lateralize or regionalize the epileptogenic zone [16, 17]. In a mixed cohort of adult and pediatric patients (mean age 30.8 years; range, 8–57 years) with HS or non-lesional MTLE, seizure freedom correlated with regionalized ictal onset, the absence of contralateral propagation and lateralization of interictal discharges to the operated temporal lobe. When these features are present the likelihood of postoperative seizure freedom is increased [16].
Conversely, postoperative persistence of interictal discharges is linked to poor outcome with a 71 % negative-predictive value for seizure freedom [18]. Rathore and Radhakrishnan (2010) reviewed 1,345 adults and children from 20 studies and noted that postoperative interictal discharges were associated with unfavorable seizure outcome for all resections (odds ratio 3.3, 95 % CI: 2.5–4.5). Further classification by anatomical location showed that the odds of poor seizure outcome was more likely for extratemporal resections (odds ratio 5.6; 95 % CI: 3.9–9.3) compared to temporal resections (odds ratio 2.5; 95 % CI: 1.6–4.0) if postoperative interictal discharges were present [18].
Neurophysiological Findings
The predictive power of scalp interictal and ictal activity is in part related to their assistance with intracranial electrode placement to precisely delineate the epileptogenic zone [6, 17]. This is particularly relevant in MRI negative cases. Jayakar et al. (2008) examined 102 pediatric patients with normal or non-focal MRI scans and identified two variables predicting seizure freedom- convergent focal interictal spikes on scalp EEG and completeness of resection. The definition of completeness of resection included electrophysiologic delineation of the ictal onset zone by intracranial EEG findings consisting of focal transformations into rhythmic activity, bursts of high frequency discharges, repetitive spiking or electrodecremental patterns [17]. Abnormal intracranial EEG findings are more predictive of seizure freedom when they are concordant with anatomical abnormalities. In the pediatric cohort described by Paolicchi et al. (2000), completeness of resection of the abnormal anatomic and electrographic region was the only predictor of seizure freedom [6].
The overall utility of further neurophysiological investigations remains controversial and unclear. There are no outcome studies evaluating the role of EEG source localization in children. A meta-analysis of adult and pediatric studies of magnetoencephalography found insufficient evidence to support a positive relationship between MEG and seizure freedom [19], whereas subsequent reclassification produced the opposite finding [20].
Surgical Predictors of Seizure Freedom
Completeness of Resection
Completeness of resection is the primary determinant of postoperative seizure freedom [6–8, 21]. Most studies define completeness of resection as the complete removal of the epileptogenic zone defined by intraoperative or extraoperative subdural EEG and, in lesional patients, removal of the lesion based on the postoperative MRI.
Patients with incomplete resections can still do well [21]. Thus, children should not be excluded from surgical consideration based on the likelihood that surgery would result in an incomplete resection of either the ictal onset zone or the lesion. In most cases, proximity to eloquent cortex limits the resection boundaries and is responsible for residual tissue. For patients with incomplete resection, factors including unilobar procedures, temporal resection, and the finding of a contiguous lesion on MRI are associated with seizure freedom [21].
Site of Resection: Temporal Versus Extratemporal
Higher rates of seizure freedom are observed in children undergoing temporal compared to extratemporal resections, a finding consistent across all age groups [11, 12, 14, 15, 22–26]. Completeness of resection with diffuse lesions in extratemporal lobe epilepsy, compared to common focal lesions of the temporal lobe such as hippocampal sclerosis, can be more difficult to achieve due to more complicated localization and surgical access issues. In addition, the anterior temporal lobe is more amenable to aggressive resections which increases the likelihood of completeness and contributes to the superior prognosis [10, 14]. Positive and negative predictors of seizure-free outcome in temporal lobe epilepsy are shown in Table 4.2
Positive Predictors | Negative Predictors |
---|---|
Lesion on MR Lateralized interictal or ictal EEG abnormality (not necessarily localized to temporal lobe) At least one semiology sign of temporal lobe origin at onset of the habitual seizure | Sensory motor deficit Intellectual disability MRI abnormalities extending outside of temporal lobe History of generalized seizures Status epilepticus Unremarkable histology Acute postop seizures Ipsilateral epileptiform activity on postoperative EEG |
Postsurgical Predictors of Seizure Freedom
Histopathology
Histopathologic substrate is variably reported as a predictive outcome factor in large mixed cohorts of postoperative patients which makes it difficult to definitively define its role in seizure outcome in children. Two studies reported improved outcomes in pediatric patients with glial-neuronal tumors and focal cortical dysplasia while inflammation, polymicrogyria, and “normal tissue” correlated with diminished rates of seizure freedom [10, 14]. For temporal lobe epilepsy, children with HS and tumors fare better than all other reported substrates [12].
Other studies have generated conflicting findings. D’argenzio et al. (2012) created Cox proportional hazard model survival plots for seizure recurrence in relation to etiology in a cohort of 80 pediatric ETLE patients (Fig. 4.3) [27]. Nonspecific pathology (scar tissue, gliosis, atrophy, perinatal injury, and normal brain) was the only variable significantly associated with a higher risk of seizure recurrence compared to focal cortical dysplasia; whereas, the presence of a low-grade tumor or tuberous sclerosis complex revealed no comparative difference. Similar poor outcomes have been demonstrated in lesional TLE when the histopathology is unremarkable [28].


Fig. 4.3
Cox proportional hazards model survival plots depicting seizure freedom with respect to histopathology. (a) Survival plot up to 36 months for the whole study population (n = 80). (b) Survival plot from 24 to 72 months for patients with a follow-up of 2 years or more (n = 50). The table shows the number of patients at risk of seizure relapse by year of observation and etiology (With Permission: John Wiley and Sons, D’Argenzio et al. [27], Fig. 2.1)
Postencephalitic epilepsy in children tends to be quite intractable and is associated with particularly poor outcomes despite localization of seizure foci with extensive presurgical evaluation and invasive monitoring [29–31]
The wide variation in seizure outcome in relation to histopathology suggests an indeterminate correlation and need for more data.
Type of Surgery
Hemispherectomy, Hemispherotomy, and Multilobar Surgery
Hemidisconnection procedures involve the surgical removal of a portion of one cerebral hemisphere or the disconnection of the lobes within that hemisphere. The classic anatomic hemispherectomy procedure has been modified in an effort to improve outcomes and reduce complications [32]. Typically, hemidisconnection is performed in patients with drug-resistant epilepsy arising from one cerebral hemisphere with evidence of concurrent hemiparesis and hemianopic visual field defect. For all patients under consideration for a hemidisconnection, the possibility of new neurologic deficits arising from the surgery is weighed against the potential neurodevelopmental benefits of seizure control [33].
The largest review to date reported seizure freedom or Engel Class Ia outcomes in 66 % (112/170) of patients at mean follow-up of 5.3 years [33]. Major improvement (>90 % reduction in seizures or greater) was obtained in 80 % of this cohort at 4.8 years median follow-up. This result is consistent with reported seizure-free outcomes ranging from 41 to 83 %.
Seizure freedom tends to be enduring in hemidisconnection patients. Moosa et al. (2013) used a Kaplan-Meier survival analysis to estimate seizure-free rates at 1, 2, and 5 years postsurgery at 76, 71, and 63 %. The majority of patients who fail hemidisconnection do so in 4–6 months, whereas, seizure freedom persists in patients who are seizure-free at 6 months postoperatively. Patients who are seizure-free at 6 months have a 98, 92, and 81 % chance of seizure freedom at 1, 2, and 5–10 years, respectively [33].
The relationship between substrate and seizure outcome in hemidisconnection patients is less clear as studies reveal contradictory results [34, 35]. Many studies separate substrates into developmental (e.g., hemimegelencephaly), acquired (e.g., encephalomalacia as a sequelae of stroke) and progressive (e.g., Rasmussen encephalitis).
Studies that have established a relationship between seizure freedom and substrate report poorer seizure outcomes for developmental lesions, especially hemimegalencephaly [36, 37], whereas, acquired and progressive substrates are associated with better seizure outcomes [35–37]. In the latter study, older age at surgery predicted seizure freedom but the authors recognized that older children were more likely to undergo hemidisconnection for acquired rather than developmental substrates. Seizures due to developmental substrates present earlier in life and are operated on at an earlier age, especially hemimegalencephaly, falsely indicating that older children fair better in terms of seizure outcomes [35]. Notable negative predictors of seizure outcome for hemidisconnection include bilateral PET abnormalities [33] and early postoperative seizures [33, 35]. Bilateral interictal and ictal EEG and bilateral MRI abnormalities do not correlate with seizure outcomes [33–35, 38].
Multilobar surgery is performed more often in childhood [6, 14, 39]. In three different large pediatric epilepsy surgery cohorts, reported seizure-free rates after multilobar resection were 15 % (64/425), 22 % (25/113), and 69 % (43/62 posterior cortex surgeries) [14, 40, 41] compared to 11.5 % (16/139) and as low as 3 % in other series reporting on mixed cohorts of adults and children [42, 43]. Long-term seizure freedom after childhood multilobar resections ranges from 55 to 68 % [14, 40] with resections within the posterior cortex resulting in better outcomes than in anterior locations [41]. Importantly, in a cohort of 63 pediatric and adult patients, Sarkis et al. (2012) showed that seizure freedom for multilobar resections typically decreased over time [43]. The likelihood of seizure freedom was 71 % at 6 months followed by 64, 52 and 41 % at 1, 5 and 10 years (Fig. 4.4).


Fig. 4.4
Kaplan-Meier curve showing postoperative survival rates for multi-lobar resection at 6 months, 1 year, 5 years, and 10 years (With permission: John Wiley and Sons, Sarkis et al. [43], Fig. 3.2)
Epileptologists recommending hemidisconnection often consider more restricted multilobar resections to spare patient morbidity such as hemiparesis or visual field deficit. No studies have directly compared hemidisconnection to multilobar resection in the pediatric age group but Cossu et al. (2008) found that a tailored “wide multilobar” approach aiming to spare eloquent cortex resulted in relatively poor outcomes compared to hemispherectomy [14].
Focal Resections: Extratemporal and Temporal Lobes
A global summary of seizure-free outcomes by anatomical lobe of origin is given in Table 4.3 and a summary of outcomes of the major studies referenced in this chapter can be found in the Appendix.
Table 4.3
Reported percent seizure-free outcomes by anatomical site
Lobe | Seizure-free outcomes (%) |
---|---|
Frontal | 33.7–66 |
Cingulate | 100 |
Insular | ~80 |
Occipital | 30–69.2 |
Parietal | 40–82 |
Temporal | 63.2–85 |
Frontal Lobe
Reported rates of postoperative seizure freedom in frontal lobe epilepsy (FLE) in mixed adult-pediatric series are highly variable, ranging from 20 to 60 %. There is little information regarding FLE surgical outcomes in childhood populations and all existing data are derived from retrospective case series or uncontrolled meta-analyses. Rates of seizure freedom in the pediatric population range from 34 to 66 % at a mean age of 9–11 years; variable follow-up from a minimum of 1–2 years, and/or mean follow-up from 34 to 40 months [9–11, 44–46].
Predictors of FLE seizure freedom include the presence of a gross structural lesion, abnormal preoperative MRI, and localized ictal findings with total resection of the lesion being the most significant single predictor of seizure freedom [44, 45]. However, the presence of a lesion does not guarantee a better outcome [9] and non-lesional FLE surgery outcomes are poorer than non-lesional posterior cortex surgeries [10]. This difference may be related to the establishment of complex neuronal networks in the frontal lobe and the surgical constraints conferred by language and motor cortex. These factors also likely contribute to the poorer outcomes in lesional and non-lesional FLE in comparison to temporal lobe epilepsy (TLE) [9, 10].
Cingulate Epilepsy
Due to its deep anatomical location and various inter-neuronal connections, localization of ictal onset within the cingulate gyrus is challenging and associated with seizure outcomes in children that are likely underreported [47]. Data is limited to small series and individual case reports with consequently limited follow-up. In a large mixed adult-pediatric cohort [47] that included three anterior and two posterior cases, all cases were lesional and had mixed neoplastic and non-neoplastic pathology. All patients were seizure-free between 1 and 11 years postsurgery.
Insular Lobe
Reported seizure outcomes after insular resection are variable and based on small pediatric cohorts in long-term epilepsy surgery studies. More recent case reports describe good outcomes [48–50]. Von Lehe et al. (2009) reported six children in a mixed pediatric-adult sample with follow-up ranging from 12 to 164 months [51]. Four patients had lesions localized solely to the insula; five were seizure-free, and one achieved a 50 % seizure reduction.
Occipital Lobe
There is a paucity of studies in the adult and mixed adult-pediatric literature reporting occipital lobe epilepsy (OLE) surgery, and only one study explored surgical outcomes for OLE in a pure pediatric cohort [41]. All other reports describe either mixed adult-pediatric cohorts or mixed anatomical surgical locations (i.e., “posterior cortex” surgery) encompassing the parietal and occipital lobes or multilobar resections. The small number of studies is due to the low incidence of focal lesional, “pure” occipital lobe epilepsy, and the diminished reliability of standard investigations to localize occipital lobe ictal onset [52]. The incidence of occipital lobe epilepsy in children is unknown but believed to be rare with only one large case series, albeit an older study, showing an incidence of 8 % [53, 54]. Investigations including video EEG, SPECT, and MRI are unreliable predictors of occipital seizure onset [55].
Surgery for OLE is less successful than temporal lobe epilepsy surgery. Studies reporting outcomes in OLE are variable due to non-standardized inclusion criteria, mixed underlying substrates, and inconsistent use of seizure outcome scales [52]. The current literature suggests a range of seizure freedom from 50 to 69.2 % in mixed cohorts of adult and pediatric patients with OLE with follow-up ranging from 13 to 157 months [41, 52, 55–57].
Pediatric outcomes can be extracted from two studies [41, 56]. The first is a series of pediatric posterior cortex surgeries of which eight patients had surgery for “pure” OLE; all were seizure-free at follow-up [41]. Mean age of seizure onset was 8.5 years but specific duration of follow-up was not reported. The second study (Tandon et al., 2009) reported a mixed adult-pediatric sample with 11/21 patients under age 17 years [56]. This cohort was followed from 34 to 157 months with 55 % seizure-free at the last follow-up.
There is a trend in the adult and pediatric literature that particular clinical features can be associated with anatomical features of OLE. OLE is more likely to present with preoperative visual field deficits in comparison to parietal or multilobar occipital epilepsies but the deficit tends to be less clinically significant [52, 56]. Medial or lobar occipital lesions have a higher likelihood of preoperative visual field defects while lateral or basal occipital lesions are more likely to present with visual auras and concordant lateralized scalp EEG onset [57]. Jobst et al. (2010) found better outcomes in patients with inferior occipital lobe seizure onset [55].
Parietal Lobe
Outcomes of PLE surgery in children have been reported only rarely as a distinct cohort. Gleissner et al. (2008) described 15 children who underwent parietal lobe surgery for epilepsy of various pathological diagnoses [58]. At 1-year follow-up, 87 % (13/15) were seizure-free. Long-term follow-up with a mean interval of 4.4 years (range: 2–9 years) was available for 11 patients with seizure freedom of 82 %.
Temporal Lobe
The pediatric epilepsy surgery literature typically separates out temporal lobe epilepsy (TLE) and discusses extratemporal lobe epilepsy (ETLE) as a collective cohort although TLE accounts for only 15–20 % of epilepsy cases in children [12, 59]. Similarities and differences exist in presentation of TLE in young children, older children, and adults. Despite these differences, children have similar outcomes as adults and surgery for TLE in children has been proven to be safe and effective [23].
One consistently identified difference between children and adults is TLE etiology. Whereas, hippocampal sclerosis (HS) is the most common cause of intractable TLE in adults, TLE etiologies in children are more variable [12]. In a recent systematic review of TLE surgery, children with HS and tumors are more likely to experience long-term seizure freedom [12].
There are no published randomized control trials in pediatric TLE surgery and all published studies are either retrospective or prospective observational studies with the vast majority having a cross-sectional or longitudinal design with no control group. Skirrow et al. (2011) analyzed long-term TLE surgery outcomes against a small nonsurgical control group (n = 11) and found improved outcomes in the cohort of 42 patients comprising the surgical group (86 % vs. 36 %, respectively) at mean follow-up of 9 years [60].
Individual published cohorts of TLE surgery in children ranging from 19 to 130 patients with follow-up of 1–23 years reveal rates of seizure freedom between 63.2 and 85 % which are comparable to the published adult findings [23, 28, 59, 61–65]. A recent review by Englot et al. (2013), covering the period between 1993 and 2012, accumulated data on 1,318 pediatric patients from 36 studies and found that 76 % (1,002/1,318) were seizure-free at last postsurgical follow-up with a minimum follow-up of 1 year. Ages of the patients ranged from 0 to 19 years (mean [±SEM] 10.7 ± 0.3 years).
Lopez-Gonzalez et al. (2012) showed that seizure freedom rates typically decrease over time in children. This group used a Kaplan-Meier seizure-freedom curve to show that the rate of seizure freedom in their population was 76, 72, 54, and 41 % at 1, 2, 5, and 12 years postsurgery (Fig. 4.5). Although an explanation for this finding is not forthcoming, it is possible that secondary epileptogenesis or incomplete resection of the ictal onset zone was responsible.


Fig. 4.5
Kaplan-Meier curve expressing seizure-free outcomes at 1, 2,5, and 12 years after temporal lobe resection in children with TLE (n = 130; from 1996 to 2006) (With permission: Wolters Kluwer Health, Lopez-Gonzalez et al. [62], Fig. 5.6.1)
Age of the pediatric patient is an essential factor to consider in TLE surgery evaluation and has been analyzed extensively [12, 28, 63, 65, 66]. It is important to acknowledge that cortical maturation likely plays a role in the presentation of TLE [66] and incorrect interpretation of semiology in young patients may impede surgical evaluation by falsely identifying a concordant semiology as a non-concordant one. Younger children tend to have fewer auras and dyscognitive features and more prominent motor manifestations; some have been reported to present with epileptic spasms [28, 63].
A recent systematic review of TLE surgery outcomes in children did not stratify outcomes by age, but this data can be found in some of the studies they included [12]. Developmental substrates, malignant tumors, and dual pathology (hippocampal plus extrahippocampal findings) tend to predominate in younger children and are all associated with less favorable seizure-free outcomes [12, 23, 63]. Despite this, it appears that even in young children seizure-free outcome rates still approximate those in older children and adults. Mittal et al. (2005) separated their cohort into children (<12 years old) and adolescents (13–18 years old) and found that, at the last follow-up, 84.8 % of children were seizure-free compared to 79.4 % of adolescents (median follow-up of 11.3 years). The rate of persistent seizures (Engel Class III or IV) was comparable at 13.0 and 14.3 %, respectively. The group from the Hospital for Sick Children in Toronto, Canada reported no differences in outcomes in 106 TLE surgery patients based on age [22]. Maton et al. (2008) analyzed a cohort of pediatric patients less than 5 years of age (mean age, = 26 months) with at least 2 years of follow-up and found that 65 % of patients were seizure-free and 15 % had >90 % seizure reduction.
Corpus Callosotomy
For patients with drug-resistant seizures that are not amenable to focal resection, corpus callosotomy (CC) can be a useful treatment option in appropriately selected patients whose predominant seizure type is drop attacks. Often there may be discussion about the merits of callosal section vs. vagal nerve stimulation (VNS); comparative studies suggest superiority of callosotomy over VNS for atonic seizures. VNS alternatively may result in improvement in a wider range of seizure types [67, 68]
CC is primarily indicated for atonic drop attacks but is also beneficial for complex partial seizures with rapid secondary generalization without a defined epileptogenic focus [69]. Disconnecting the corpus callosum blocks the interhemispheric spread of seizures; thus, the primary aim of callosal sectioning is to reduce seizure intensity and decrease seizure burden rather than eliminate seizures completely [70, 71].
Observational studies support the effectiveness of CC for drop attacks and GTC seizures [69–71]. A prospective mixed adult-pediatric cohort with a mean age at surgery of 13 years (range: 2–41 years), went from 190 drop attacks per month presurgery to 100 per month at 2 year follow-up and 20 per month between 5 and 10 years [69]. Seizure freedom was obtained in 10/18 patients and 3 of the remaining 8 patients achieved a >75 % seizure reduction.
Sunaga et al. (2009) reported that complete callosotomy increases seizure freedom for drop attacks in comparison to partial sectioning. In 78 patients (51 patients less than 18 years of age), 90 % who underwent complete callosotomy were free of drop attacks with a relapse rate of 7 % at 6 years in comparison to 54 % seizure freedom after partial callosotomy and a relapse rate of 31 % at 6 years. Twenty-one percent of patients developed new postural seizures.
GTC seizures improved after callosotomy in a retrospective review of 95 mixed adult-pediatric patients (mean age of 24 years) [70]. Forty-two percent were seizure-free at a mean follow-up of 17 years and 35 % were free from drop attacks over the same time period.
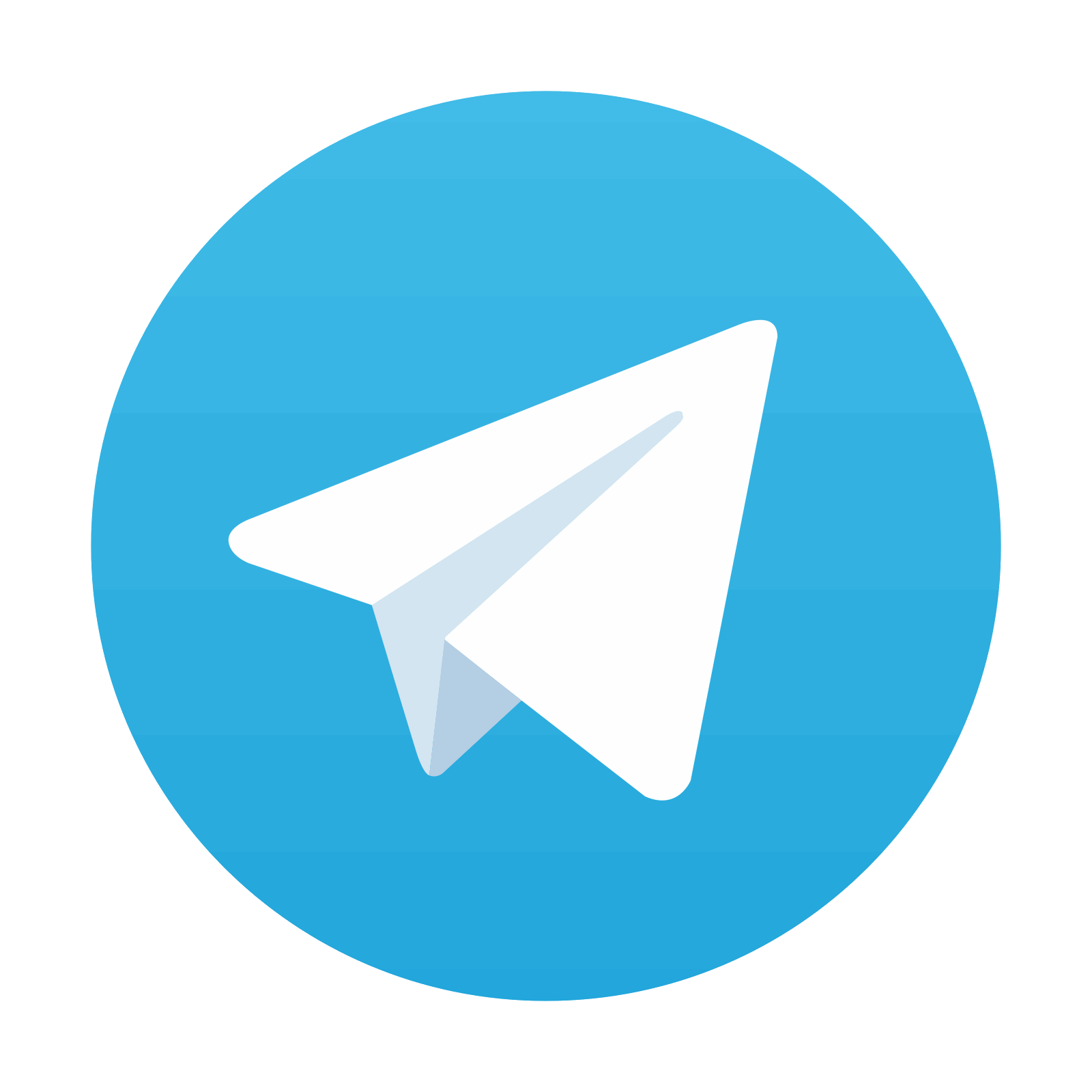
Stay updated, free articles. Join our Telegram channel

Full access? Get Clinical Tree
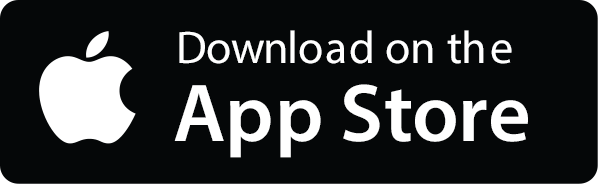
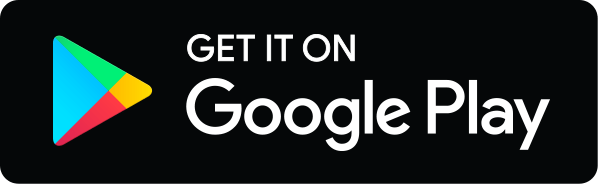