Summary of Key Points
- •
Lumbar interbody cages are commonly used to supplement fusion procedures and function to stabilize the anterior spine, increase the area for fusion, provide indirect decompression of nerve roots, and restore lumbar lordosis.
- •
Cages may be inserted via anterior, lateral, or posterior approaches.
- •
Cage designs incorporate features to provide immediate stability and resistance to migration, allow ingrowth of fusion, and localize the implant on radiographs.
- •
Supplemental fixation increases biomechanical stability of the cage construct.
- •
Subsidence is related to disc space preparation, cage selection and implantation, host bone quality, and supplemental fixation.
- •
Complications of cage usage can be minimized by understanding the local anatomy, careful and durable insertion technique, and use of intraoperative radiography and neuromonitoring.
Lumbar fusion procedures have become increasingly common in the treatment of degenerative, traumatic, neoplastic, and deforming conditions of the lumbar spine. As techniques have advanced to increase the success of these procedures, the adjunctive use of interbody cages to add structural stability and to improve fusion rates has become more common over time. This chapter describes the types, indications, and both advantages and disadvantages of the various interbody fusion devices applicable to the lumbar spine.
Historical Perspective
The concept of interbody fusion was first introduced in the 1940s by Ralph Cloward, who pioneered the posterior lumbar interbody fusion technique. Toward the end of the 1970s, Dr. George Bagby introduced the stainless steel basket as an adjunct to spinal arthrodesis. He implanted a cervical interbody cage made of stainless steel in a racehorse with successful fusion. He called his technique distraction-compression stabilization . In the 1980s, Kuslich and others adapted Bagby’s basket for human use. Subsequently, threaded posterior lumbar interbody fusion (PLIF) cages were introduced. Threaded PLIF cages were thought to have biomechanical advantages over traditional dorsolateral fusion, including providing anterior column support, placement closer to the vertebral center of rotation, and a reduced bone graft requirement. The Food and Drug Administration (FDA) approved anterior lumbar interbody fusion cages in 1996. Until the end of the 1990s, most cages were made of titanium. It was not until the late 1990s that the first radiolucent polymer implants (e.g., polyether ether ketone [PEEK]) cages, were introduced. Since the early 2000s, the field of interbody cage technology has exploded, and a variety of implants have been introduced into the market.
Interbody Fusion
It has been demonstrated biomechanically that the most effective means of eliminating motion between two vertebrae is through the disc space rather than the facet joints, transverse processes, or spinous processes. Traditionally, posterolateral fusion alone has been associated with a higher pseudarthrosis rate than has a circumferential fusion. Furthermore, it has been shown that posterolateral fusion does not completely achieve immobilization of the motion segment despite the presence of a solid fusion. This result makes intuitive sense, as 80% of spinal loads are transmitted through the anterior column, and a “diving board effect” occurs when the mobile body-disc interfaces are preserved.
Additionally, there are several other potential advantages to the interbody fusion techniques. Because the center of body mass lies anterior to the spine, and the function of the spine en bloc is to act as a tension band in the upright posture, grafts placed within the anterior and middle columns are subject to compression under physiologic loads. Fusion, therefore, would be expected to be promoted according to Wolff’s law. With that said, careful surgical technique is also essential for creating an optimum milieu for fusion. Whereas discectomy and removal of the cartilaginous end plates exposes a large surface area of well-vascularized bone, overzealous decortication may impair the stability of the final bone-graft-bone configuration by diminishing the structural integrity of the vertebral end plates and potential subsidence.
The use of structural interbody devices and grafts can be mechanically advantageous, as well. Proponents of interbody fusion argue that disc height and lumbar lordosis are better restored, thereby improving overall alignment in patients with positive sagittal balance. Although most patients with degenerative conditions of the lumbar spine affecting one or two levels do not have significant sagittal imbalance, failure of restoration of segmental lordosis at each level at the index operation combined with future adjacent segment degenerative changes (which are almost uniformly kyphotic) may ultimately result in a flat back deformity. From a neurologic standpoint, anterior implants facilitate indirect decompression of nerve roots by rostral-caudal distraction of the neuroforamina, much akin to opening a cigar cutter.
Despite the aforementioned theoretic advantages of anterior column fusion, it is not yet clear whether clinical outcomes are improved in comparison with a posterolateral fusion alone. The disadvantages of including an interbody device include the added cost, increased operative time, risk of neurologic injury due to nerve root or dural sac retraction, and the long-term effects of complete immobilization of a motion segment on the adjacent lumbar levels. One study found that at long-term follow-up (average 14 years), clinical outcomes were not correlated with disc height after ventral fusion using tricortical iliac crest bone.
Lumbar interbody fusion can be performed from anterior (anterior lumbar interbody fusion, ALIF), posterior (transforaminal lumbar interbody fusion, TLIF; posterior lumbar interbody fusion, PLIF), or transpsoas/lateral (direct lateral interbody fusion, DLIF; extreme lateral interbody fusion, XLIF) approaches. Anterior exposure to the disc space allows a more complete discectomy and the insertion of larger interbody implants. Advocates of the anterior approach argue that improved access to the disc, more complete discectomy, and superior end-plate preparation is likely to yield a higher fusion rate and improved lordosis. It is easier to restore segmental lordosis from an anterior approach because circumferential release of the annulus fibrosus allows more effective restoration of disc space height, and a larger cage can be inserted in terms of both height and width.
The implant or graft must be capable of withstanding these loads to allow fusion to occur. Early on, autologous bicortical iliac crest autograft was the gold standard, occasionally supplemented with a screw and washer to keep the graft in place. However, high rates of pseudarthrosis, graft collapse, migration, and loss of correction were observed with the use of autologous iliac crest alone. This led to the development of prefabricated bone and synthetic cages, which obviated the need for a harvest procedure and could be produced in a variety of convenient sizes to facilitate faster implantation.
Structural interbody cages have thus become more popular because of the high rate of pseudarthrosis associated with use of interbody bone graft alone. A cage provides immediate rigid axial mechanical support and stability postoperatively, allowing the graft material inside the cage as well as surrounding the cage to form a solid biologic fusion. There are a number of options to provide anterior column support, including cortical allograft, vascularized autograft (usually rib or fibula), vertical mesh cages (e.g., Harms cage; Fig. 81-1 ), carbon fiber cages (Brantigan cage; Fig. 81-2 ), cylindrical threaded cages (BAK, LT cages; Fig. 81-3 ), and PEEK cages ( Fig. 81-4 ).




Biomechanics
Anterior lumbar interbody fusion may be achieved with a stand-alone cage or supplemented with dorsal or ventral instrumentation. At present, there is no consensus for performing one procedure over the other. Proponents of the stand-alone ALIF contend that an exclusively ventral approach allows for superior preparation of the fusion surfaces while preserving the dorsal elements, which avoids perioperative morbidity related to the dissection of the spinal muscles and complications from the dorsal instrumentation. On the other hand, advocates for dorsal instrumentation assert that supplementary dorsal stabilization increases system constraint, creating an environment that is more conducive to fusion. A number of biomechanical studies have investigated the stabilization provided by stand-alone ALIF cages versus those supplemented with dorsal instrumentation. In general, stand-alone interbody cages significantly reduce flexion and lateral bending compared to intact spines. Constraint of axial rotation, however, has not been as successful, although studies suggest that ALIF procedures generate better stabilization in torsion than does PLIF. Regardless of the surgical approach, interbody cages do not stabilize in extension, although it is unclear whether the lack of stabilization in extension is clinically relevant and should warrant dorsal instrumentation.
It is widely accepted that supplementary dorsal instrumentation further increases range of motion constraint and stiffness in one or more planes compared to stand-alone ALIF cages. However, the constraint parameters that are ideal for facilitating fusion have not been defined. If supplementary dorsal instrumentation is required to achieve a stable environment for fusion, the advantages of stand-alone ALIF over dorsal procedures are lost, although adjunctive percutaneous pedicle screw insertion may be considered for a “less invasive” option for posterior stabilization.
Clinical studies comparing stand-alone interbody cages and cages with dorsal stabilization have been equivocal. Fusion rates have been reported between 82% and 98% for both procedures, and patient satisfaction rates have also been similar between stand-alone and circumferential procedures. In a study of 71 patients who underwent stand-alone ALIF with bilateral low-profile interbody fusion cages, the overall fusion rate was 86% with an average time to fusion of 10 months. Although the single-level fusion rate was 93%, the rates for two- and three-level procedures were lower, at 55% and 67%, respectively. This is consistent with other published data reporting fusion rates for both ALIF and PLIF procedures at around 90% to 94%, with lower fusion rates for multilevel procedures. This may suggest that stand-alone procedures are most beneficial for single-level fusions.
Additionally, Greenough and colleagues concluded that ALIF for a single level was associated with significantly less perioperative morbidity than were posterolateral fusion and instrumentation, although the researchers did not find a significant difference in clinical outcome between the two approaches. Nichols and associates found that the addition of a ventral plate to ALIF provided stability similar to that of supplemental dorsal fixation, with dorsal fixation providing only additional constraint in lateral bending with similar results for extension.
Modern ALIF cages are available in a variety of sizes with varying degrees of lordosis to assist with restoration of normal sagittal alignment ( Fig. 81-5 ) and are designed to be used with or without supplemental plate fixation. Whereas dorsally inserted TLIF/PLIF cages can also be contoured with a lordotic wedge, the grafts are much smaller than ALIF cages and therefore theoretically provide a less substantial immediate mechanical lordotic platform.

The sustained ability of ALIF versus TLIF/PLIF cages to correct lordosis and restore height to provide indirect decompression has been directly studied. Superior results are noted for ALIF cages, which restored foraminal height by 18.5% and lumbar lordosis by 6.2 degrees, compared to TLIF cages, which decreased foraminal height by 0.4% and decreased lumbar lordosis by 2.1 degrees. Nevertheless, the authors did not note any clinically significant differences between groups.
Mention should be made of the more recent development and increasingly common utilization of direct lateral transpsoas technique for discectomy, interbody cage placement, and fusion. The implants carry many of the advantages of an anterior approach (greater discectomy, larger cage size) without necessitating a transabdominal approach. Multiple levels may be performed in the same operative setting with a minimally invasive approach, and disc spaces from L1 through L5 may be safely approached with care taken not to damage the lumbar plexus through the use of real-time intraoperative neuromonitoring. The L5-S1 interspace is not usually accessible with this approach, however, due to the intervening presence of the iliac bones bilaterally. Studies have compared mechanical benefits of supplemental posterior fixation as adjunctive stabilization for transpsoas techniques, and these can be performed in an open or percutaneous fashion at the discretion of the surgeon ( Fig. 81-6 ).

Expandable interbody cages are also gaining popularity owing to their versatility in insertion and interbody fit. Although long-term clinical correlations have yet to be determined, biomechanical evidence presented by Pekmezci and colleagues suggest greater contact areas and increased end-plate forces in hyperlordosis of expandable versus fixed cages in a cadaveric model; end-plate forces in neutral and hypolordotic alignment were similar. Continued study is warranted regarding the devices’ long-term effectiveness.
Cage Material and Design
A variety of cages for lumbar interbody fusion surgery are currently available from a number of manufacturers. Cages come in various sizes and shapes and include circular, tapered, rectangular, and curved (banana-shaped) versions. Some devices have design features such as radiolucency that allow assessment of fusion. Some have end-plate interdigitation with serrations, screw threads, or spikes, and others are modular. Modular cages are designed to customize the fit of the cage to each patient’s intervertebral geometry. The following features are desirable in the design of an interbody cage:
- •
A cage should ideally have a hollow region of sufficient size to allow packing of bone graft or bone graft substitute.
- •
It should be structurally sound so that it can withstand the great forces applied to it in the immediate postoperative period and allow immediate patient mobilization.
- •
It should have a modulus of elasticity that is close to that of vertebral bone to optimize fusion and avoid subsidence.
- •
It should have ridges or teeth to resist migration or retropulsion into the retroperitoneal space or the spinal canal. Serrations on the top and bottom surfaces of the cage may improve fixation strength and diminish motion at the cage-bone interface.
- •
It should be radiolucent to allow visualization of fusion on radiographs and may have radiopaque markers to localize the precise location of the implants on intraoperative and postoperative radiographs.
- •
If inserted from a dorsal approach (TLIF or PLIF), it should be tapered, with a bullet-shaped tip to allow easier initial insertion into the disc space with minimal trauma to the adjacent thecal sac and nerve roots. This is especially beneficial when introducing the graft into narrowed disc spaces for distractive purposes.
The stiffness of a cage has been found to influence fusion rates. Ideally, a cage would have a modulus of elasticity that is similar to that of vertebral bone, which would optimize the load transfer between the cage and the adjacent vertebral bodies and reduce the effects of stress shielding on the graft material. Carbon fiber cages have a modulus of elasticity closer to that of cortical bone, while metal and titanium cages exceed the stiffness of the vertebral bone. The modulus of elasticity of stainless steel and titanium implants is 200 and 110 GPa, respectively, compared with that of vertebral trabecular and cortical bone, which is 2.1 and 2.4 GPa, respectively. Titanium cages also have the disadvantage of incomplete radiographic assessment of the fusion mass. Furthermore, owing to the mismatch of modulus of elasticity of titanium and vertebral bone, the stiffness of titanium cages may cause subsidence into the vertebral end plates.
To create a more suitable modulus bone-cage-bone transition, PEEK cages have been developed and are routinely used as interbody devices. PEEK is a semicrystalline aromatic polymer that is radiolucent and can be formed into any shape. Radiopaque markers are routinely incorporated into the borders of the cage so that the surgeon can precisely localize the implant on radiographs. PEEK is also magnetic resonance imaging (MRI) and computed tomography (CT) compatible and does not create significant implant artifact on these imaging studies.
Despite a more biologic modulus of elasticity of PEEK as compared to metal, there is some biomechanical evidence showing lower primary fixation and initial stability of PEEK cages compared to titanium cages of equal dimensions. Despite this evidence, PEEK implants have been shown to lead to excellent clinical outcomes and are widely used. Particularly when augmented with dorsal instrumentation, PEEK cages have been shown to provide stability similar to that of titanium cages, reduce stress at the end plates adjacent to the cage, and increase load transfer through the graft. In a study of lordosis correction in circumferential arthrodesis using PEEK cages, Rousseau and colleagues found that the immediate postoperative improvement in lumbar lordosis was lost at subsequent follow-up. Loss of lordosis was statistically related to the initial increase in lordosis, cage size, patient age, and presence of dorsal instrumentation and level of fusion. This tendency of returning toward preoperative sagittal balance occurred despite a 98% fusion rate; even so, the authors noted 86% excellent or good clinical outcomes. Similar loss of lordosis correction, despite solid fusion and good clinical outcomes, has been found in other studies regardless of interbody cage material.
Machined allograft interbody implants are also available to provide the ease of preparation and lack of harvesting advantages of off-the-shelf devices while maintaining the theoretic biologic advantages of a bone-bone interface. A potential disadvantage of allografts is the inherent radio-opacity, which makes visualization of fusion difficult because the space already contains bone. In a study comparing PEEK to allograft cages, Cutler and coworkers found similar rates of fusion, minimal change in postoperative lordotic angle, and Oswestry Disability Indices between the two devices.
Synthetic bioabsorbable cages have also been designed. These cages are absorbed over time, thus leaving only a bony fusion between vertebrae without any foreign material in the spinal segment. There is limited clinical experience with the use of resorbable implants in lumbar fusion surgery, and studies are contradictory. Jiya and colleagues randomized 26 patients to undergo instrumented posterior lumbar interbody fusion with either a nonresorbable PEEK cage or a resorbable poly L-lactide-co-D,L-lactide (PLDLLA) cage. The fusion rate was significantly higher with the PEEK cage compared with the PLDLLA cage. Furthermore, the PLDLLA cage showed a significantly higher rate of subsidence compared with the PEEK cage. Other studies have evaluated the fusion rate and its relationship to cage stiffness, evaluating a poly-L-lactide (PLLA) cage versus a titanium cage. After 6 months, increased interbody fusion was seen with the PLLA cages. An in vitro study showed that PLLA cages were mechanically sufficient directly after implantation. Other studies have supported the use of resorbable cages, fusion rates being comparable to those of nonresorbable implants without adverse effects related to the implant.
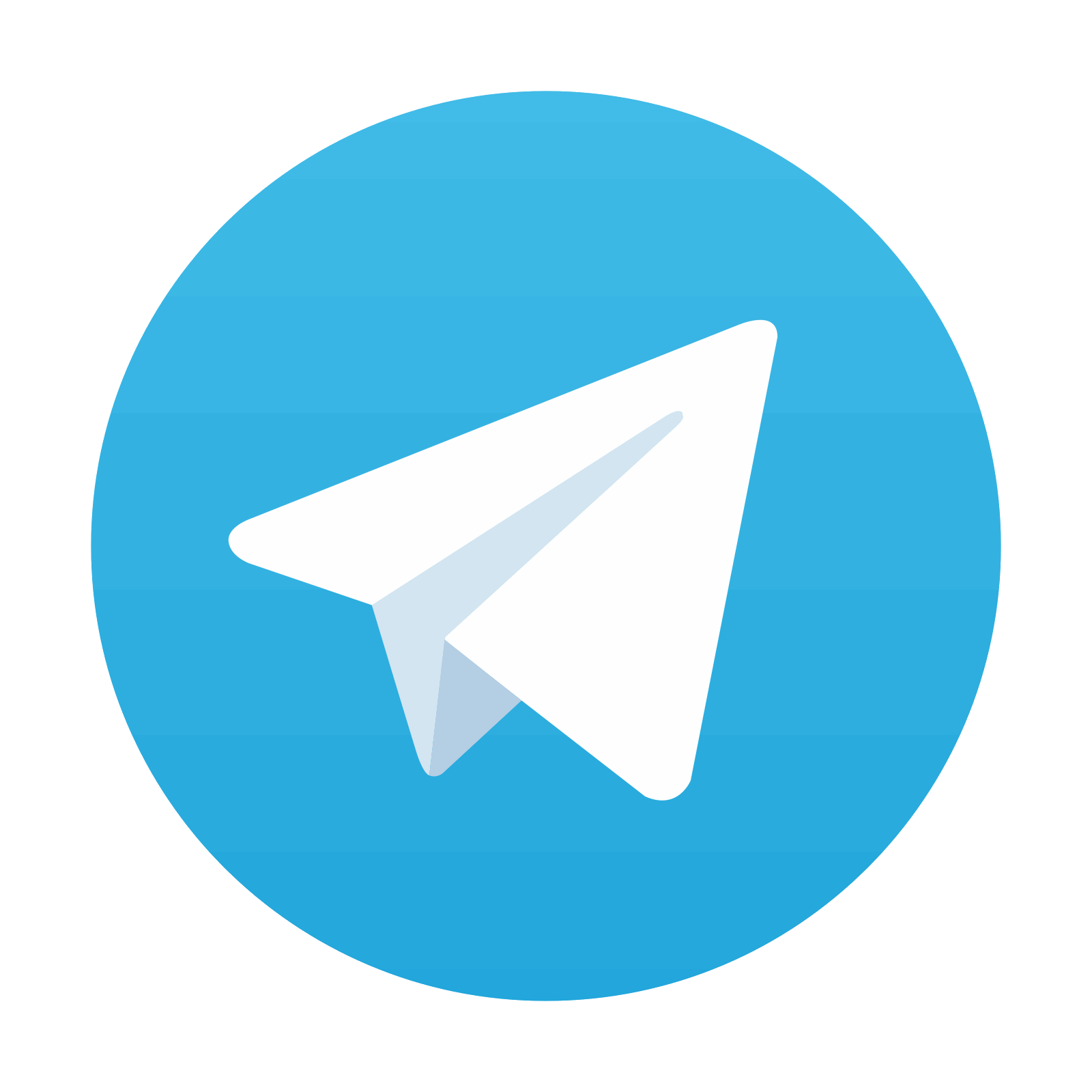
Stay updated, free articles. Join our Telegram channel

Full access? Get Clinical Tree
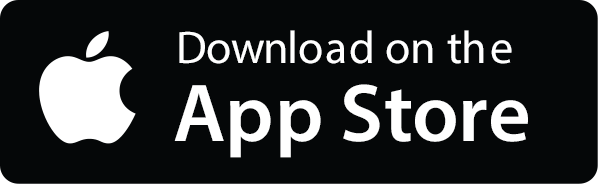
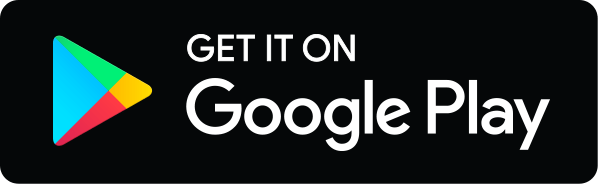