Chapter 20 Lumbar Puncture and Imaging Studies
Lumbar Puncture
Neurologists often obtain cerebrospinal fluid (CSF) by performing a lumbar puncture (LP) – one of the oldest neurologic tests still employed – under a variety of clinical circumstances. When patients have at least two elements of the relatively common triad of headache, fever, and nuchal rigidity, neurologists usually perform an LP to look for meningitis, subarachnoid hemorrhage, or other inflammatory conditions affecting the central nervous system (CNS). They also perform an LP in cases of dementia attributable to infectious illnesses. In Creutzfeldt–Jakob disease (CJD), the CSF almost always contains 14-3-3 protein; in subacute sclerosing panencephalitis, antimeasles antibodies; in acquired immunodeficiency syndrome (AIDS), retrovirus markers; and other infectious illnesses, such as cryptococcal or tuberculous meningitis, herpes simplex encephalitis, and neurosyphilis, specific antigens or DNA detectable by polymerase chain reaction. In another particularly useful test, neurologists send CSF for the JC virus in patients with AIDS who have a cerebral lesion. If present, the JC virus indicates that the diagnosis is progressive multifocal leukoencephalopathy (PML) (see Fig. 15-10). Moreover, a positive test obviates the need for a brain biopsy. Neurologists also test the CSF of patients suspected of having multiple sclerosis (MS) for oligoclonal bands and myelin basic protein (see Chapter 15). In Alzheimer disease, the CSF contains increased levels of tau protein but decreased levels of β-amyloid and Aβ42 peptide.
Diagnosing neurologic illnesses sometimes rests on abnormalities of the CSF profile, which comprises the CSF color, red and white blood cell count, and concentrations of protein and glucose (Table 20-1). For example, most infectious or inflammatory CNS illnesses cause a CSF pleocytosis (increase in the CSF white blood cell count). In these illnesses, a rise in protein concentration parallels CSF pleocytosis, and, in their hallmark, glucose concentration falls to abnormally low levels. Bacterial meningitis accentuates that profile: CSF pleocytosis is markedly elevated, with a predominance of polymorphonuclear instead of lymphocytic cells, and the glucose concentration can fall to undetectable levels. Cultures of virus, fungus, and Mycobacterium may require 1–3 weeks to identify an organism, but sometimes antigen testing can immediately indicate bacterial and nonbacterial organisms. As an exception to the general observation that infectious and inflammatory conditions produce CSF pleocytosis, in Guillain–Barré syndrome, CSF contains a markedly elevated protein concentration but little or no increase in the white cell content (the “albumino-cytologic disassociation,” see Chapter 5).
TABLE 20-1 Cerebrospinal Fluid (CSF) Profiles*
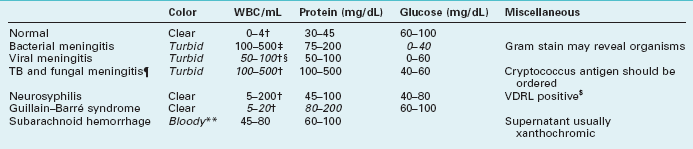
WBC, white blood cells; TB, tuberculosis; VDRL, Venereal Disease Research Laboratory.
*Characteristic abnormalities in italics.
‡Mostly polymorphonuclear cells.
§In encephalitis from herpes simplex virus, the CSF also contains red blood cells.
¶In carcinomatous meningitis, the CSF profile is similar to fungal meningitis but malignant cells may be detected on cytologic examination.
$About 40% of neurosyphilis cases have a false-negative VDRL CSF test (see Chapter 7).
**White and red cells are in same proportion as in blood (1:1000).
An intracranial mass lesion is one of the most common contraindications to an LP. This prohibition is based on the fear that an LP could suddenly reduce pressure in the spinal canal, allowing the unopposed force of a cerebral mass to lead to transtentorial herniation (see Fig. 19-3). Moreover, a CSF examination would not help in diagnosing most mass lesions because their CSF profiles are not distinctive. Although increased intracranial pressure without an associated mass lesion defines idiopathic increased intracranial hypertension (pseudotumor cerebri, see Chapter 9), in this setting neurologists perform LPs with impunity for diagnosis and occasionally for treatment. Overall, unless neurologists suspect acute bacterial meningitis or subarachnoid hemorrhage, in which case rapid diagnosis is crucial, they usually do not perform an LP or they postpone it until after imaging studies have excluded an intracranial lesion.
Computed Tomography
Although lacking fine detail, CT can clearly reveal changes in major structures (Fig. 20-1). It shows generalized cerebral atrophy, such as occurs in advanced age or Alzheimer disease (Figs 20-2 and 20-3), and atrophy of a particular region, such as with porencephaly (Fig. 20-4), Huntington disease (Fig. 20-5), and frontotemporal dementia (Fig. 20-6). Similarly, it shows expansion of the ventricles – hydrocephalus – not only as a consequence of generalized atrophy (hydrocephalus ex vacuo, Fig. 20-3), but also from normal-pressure hydrocephalus (Fig. 20-7) and CSF obstructions (obstructive hydrocephalus) (see later). CT readily detects large lesions, such as primary and metastatic tumors (Fig. 20-8). CT will also reveal subdural hematomas, except perhaps for isodense ones (Fig. 20-9). CT is even superior at finding dense, calcium-laden meningiomas (Fig. 20-10). It can show numerous small lesions, such as in toxoplasmosis (Fig. 20-11) and cysticercosis (Fig. 20-12), with the detail necessary for a firm diagnosis. (Cysticercosis, which is caused by the parasite Taenia solium, is the most common cerebral mass lesion in South and Central America.)
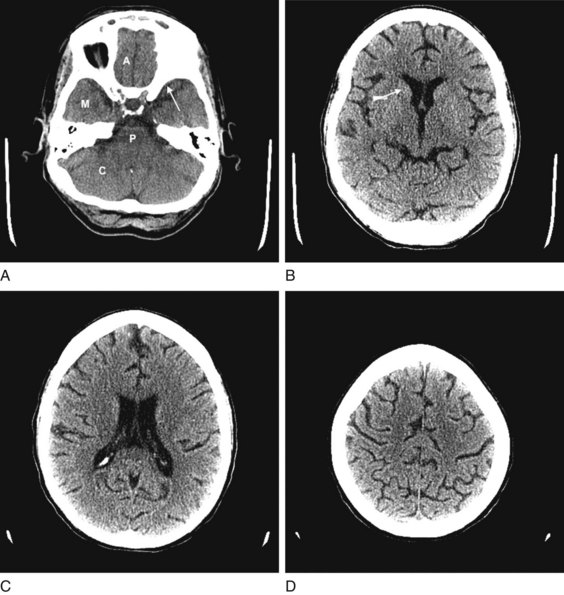
FIGURE 20-1 These computed tomography (CT) scans show progressively higher axial images of a normal brain. A, The anterior cranial fossae (A) contain the anterior frontal lobes and the olfactory nerves. The middle fossae (M) contain the anterior temporal lobes, which are situated behind the sphenoid wing (arrow). The posterior fossa contains the cerebellum (C) and the medulla and pons (P) – main components of the bulb. B, The head of the caudate nucleus (arrow) indents the anterior horn of the lateral ventricle. C, The lateral ventricles spread lengthwise in the hemispheres. D, The cerebral cortex rests against the inner table of the skull. Thin sulci separate the gyri.
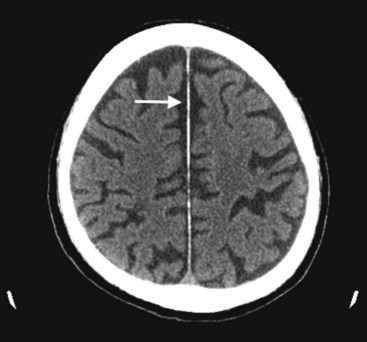
FIGURE 20-2 This computed tomography (CT) scan illustrates generalized cerebral atrophy. (See Fig. 20-18 for magnetic resonance imaging appearance of cerebral atrophy.) Because of atrophy, the gyri shrink, sulci expand, cerebral cortex retracts from the inner table of the skull and from the falx (arrow), and ventricles expand (see later). Cerebral atrophy, as pictured in this case, represents a normal concomitant of old age. Although cerebral atrophy is associated with Alzheimer disease, vascular cognitive impairment, trisomy 21, alcoholism, neurodegenerative illnesses, and treatment-resistant schizophrenia, it is not invariably associated with dementia.
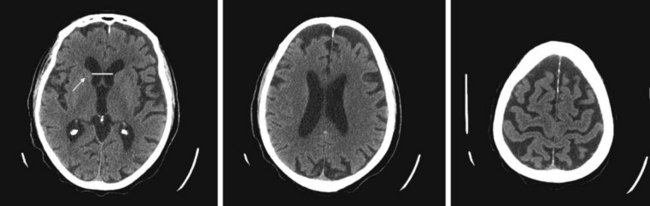
FIGURE 20-3 These three progressively higher computed tomography (CT) images (left to right) show that cerebral atrophy leads to expansion of the lateral ventricles (line in the left-most image) and widening of the third ventricle – hydrocephalus ex vacuo – as well as thinning of cerebral gyri and widening of sulci (right-most image). Nevertheless, as the left-most image shows, the head of the caudate nucleus (arrow) maintains its normal volume and continues to indent the lateral border of the lateral ventricle. In Huntington disease, by way of contrast, the characteristic atrophy of the head of the caudate nuclei allows the ventricles to bow outward (see Fig. 20-5).
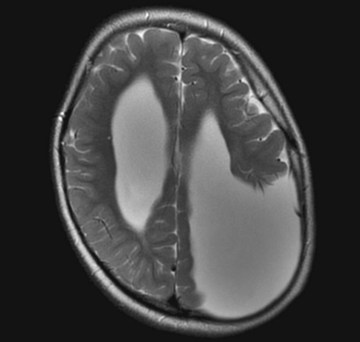
FIGURE 20-4 Computed tomography (CT) and magnetic resonance imaging (MRI), by convention, display the brain with its lateral, but not vertical, sides reversed. For example, the left cerebral hemisphere appears on the right side of the CT image while the frontal lobes still appear on the top on the image. In this MRI, the misshapen region filled with cerebrospinal fluid in the parietal lobe represents a congenital absence of brain tissue, porencephaly, in the patient’s left parietal lobe. The porencephaly displays the opposite effect of a mass lesion. In particular, the absence of mass effect draws the adjacent lateral ventricle and midline structures shift toward it.
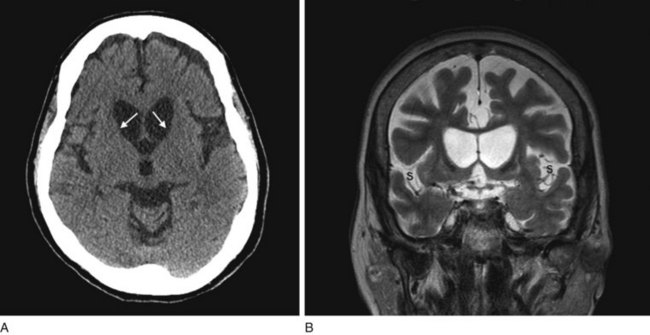
FIGURE 20-5 A, This computed tomography (CT) scan shows the characteristic abnormality of Huntington disease: the anterior horns of the lateral ventricles are convex (bowed outward) because of atrophy of the caudate nuclei (arrows). Contrast that convex shape of the ventricles in Huntington disease to the concave shape seen in normal individuals (see Figs 20-1B and 20-17) and in those with cerebral atrophy and hydrocephalus ex vacuo (see Figs 20-2, 20-3, and 20-18). In addition to the caudate atrophy, Huntington disease, like many other neurodegenerative illnesses, causes cortical atrophy with widened sulci and enlarged ventricles. B, This coronal view of the MRI of the same patient also shows the convex expansion of the lateral ventricles, large sulci, and widened sylvian fissures (S).
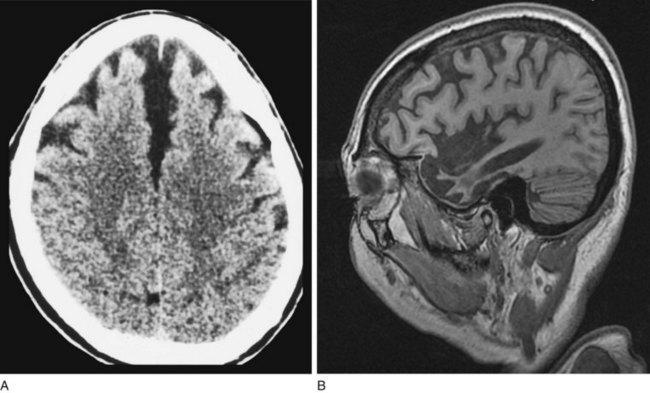
FIGURE 20-6 A, This computed tomography (CT) scan from a patient with frontotemporal dementia shows that the frontal lobes have undergone atrophy and retracted both from each other and from the inner table of the skull. Other views, which are not shown, reveal comparable changes in the temporal lobes; however, characteristically, the parietal and occipital lobes remain unchanged. B, Sagittal magnetic resonance imaging (MRI) scan from another patient with frontotemporal dementia clearly demonstrates the selective frontal and temporal lobe atrophy.
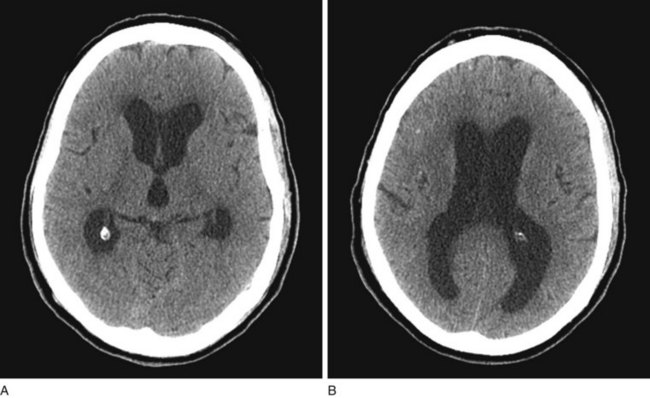
FIGURE 20-7 A, B Computed tomography (CT) scan, from a patient with normal-pressure hydrocephalus (NPH), shows widening of the third and lateral ventricles with little or no cerebral atrophy. (See Fig. 20-19 for magnetic resonance imaging scan of NPH.)
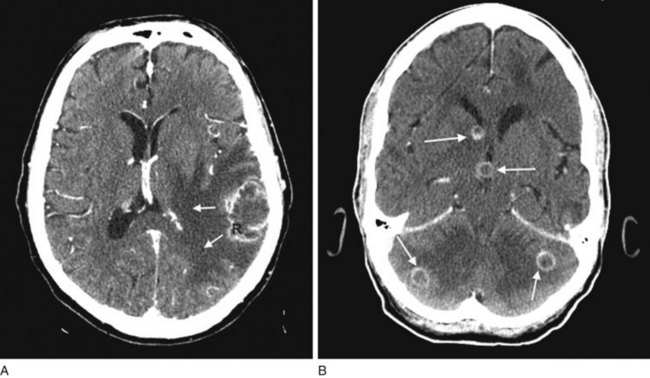
FIGURE 20-8 A, Computed tomography (CT) scan shows a glioblastoma with its characteristic white, contrast-enhanced ring (right) and black border of edema (arrows). (See Fig. 20-20 for magnetic resonance imaging showing a glioblastoma.) B, With contrast enhancement, CT shows several metastatic cerebral tumors (arrows).
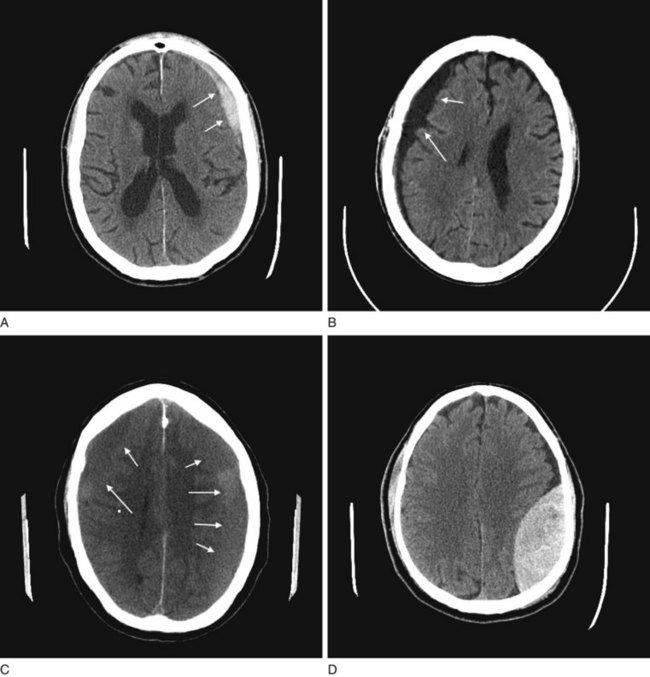
FIGURE 20-9 A, The acute subdural hematoma (arrows), overlying the patient’s left frontal lobe, contains fresh blood, which is radiodense compared to normal brain tissue. B, The chronic subdural hematoma (arrows), overlying this patient’s right cerebral hemisphere, contains aged, liquefied blood that is less radiodense than brain. Chronic subdural hematomas are typically black on a computed tomography (CT) scan and often bordered by a radiodense, contrast-enhancing membrane, not well seen here. C, As acute subdural hematomas evolve into chronic ones, their density decreases to that of the underlying brain’s density. When they become isodense, subdurals are indistinguishable. This CT shows a barely visible isodense subdural overlying both of this patient’s cerebral hemispheres. However, the membrane, compression of the gyri-sulci pattern, and shift of midline structures reveal its presence. D, This CT shows an epidural hematoma, which almost always represents acute intracranial bleed and therefore appears white. In the majority of cases, the CT shows a skull fracture, which is not seen in this view, as well as blood under the scalp. Note the hematoma’s classic elliptical shape and, because of compression, shift of the underlying brain.
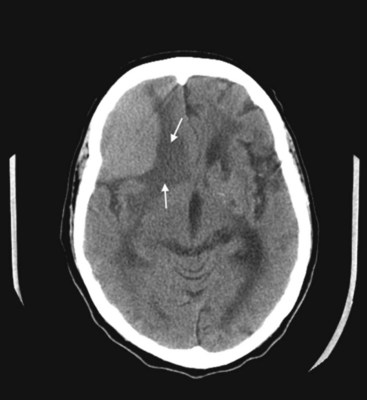
FIGURE 20-10 This coronal computed tomography (CT) scan, of a previous healthy 59-year-old-man, shows a large, rounded radiodense right frontal lesion, with a dural margin, that compresses the adjacent anterior horn of the lateral ventricle and causes some local edema (arrows). The lesion is a typical, chronic, and slowly growing meningioma. As this CT exemplifies, frontal meningiomas often reach a large size before producing seizures, neuropsychologic changes, such as apathy or disinhibition, or other symptoms.
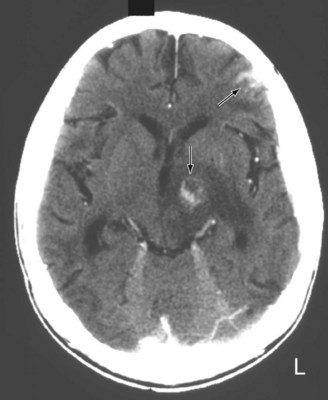
FIGURE 20-11 A computed tomography (CT) scan shows two toxoplasmosis lesions (arrows) enhanced by contrast infusion, in the left cerebrum in a patient with acquired immunodeficiency syndrome (AIDS). As in this study, toxoplasmosis has a predilection for developing in the basal ganglia in AIDS patients with CD4 counts below 200 cells/mL. The infection’s propensity to develop in the basal ganglia explains why AIDS patients who develop involuntary movement disorders, such as hemiballismus or chorea, are likely to be harboring toxoplasmosis.
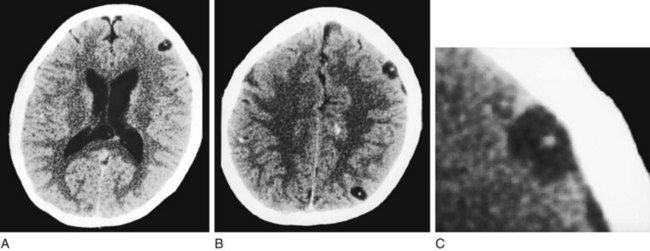
FIGURE 20-12 This computed tomography (CT) scan shows multiple cerebral cysticercosis lesions in views through the cerebrum (A) and cerebral cortex (B) and an enlargement of the frontal cortex lesion (C). These lesions, in contrast to toxoplasmosis (see Fig. 20-11), are usually situated in the cerebral cortex, contain calcification, and lack surrounding edema. Even though each cyst is small, together they exert a substantial cumulative mass effect and irritate the surrounding cerebral cortex. Their tendency to irritate the cerebral cortex explains why patients with cysticercosis often first come to medical attention because of seizures.
CT can also show strokes that are large established infarctions and those that are hemorrhagic (Fig. 20-13); however, MRI can better locate ones that are small or acute. CT is invaluable in the special situation of cerebellar hemorrhage, where rapid diagnosis is essential to prevent brainstem compression and obstructive hydrocephalus (Fig. 20-14).
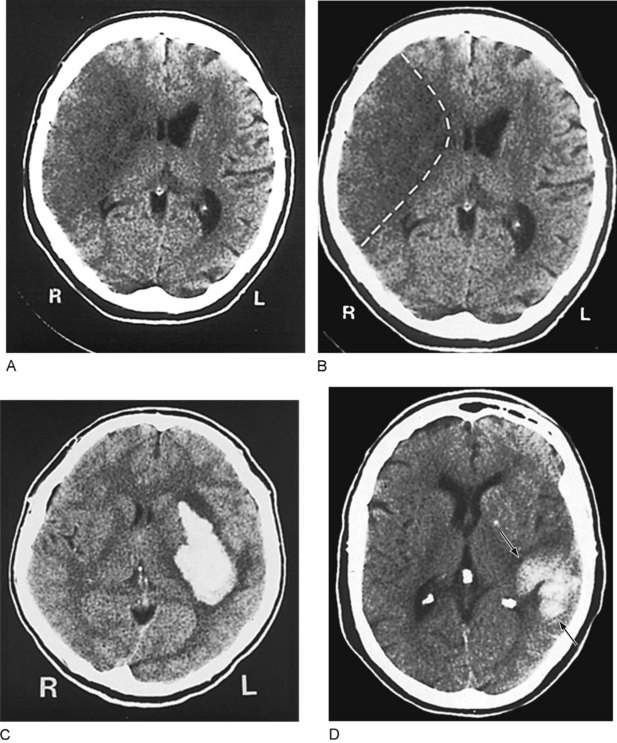
FIGURE 20-13 A, This computed tomography (CT) image shows an acute stroke from occlusion of the right middle cerebral artery. Because the infarcted area of the brain is deprived of blood, which is normally radiodense, it is darker (more hypodense) than the adjacent normal brain. In addition, its mass effect compresses the adjacent lateral ventricle and shifts midline structures. B, An outline of the stroke on the same image shows its pie-shaped area in the lateral portion of the right cerebral hemisphere, containing the origin of the corticospinal tract for the left face and arm. C, This image shows a cerebral hemorrhage that originated in the left basal ganglia. The blood, denser than the brain, forms the white plume. D, In this image, a hemorrhage (arrows) involves the left parietal lobe and compresses the occipital horn of the left lateral ventricle. The three small white objects are, laterally, the normally calcified choroid plexus of the lateral ventricles and, medially, the pineal gland.
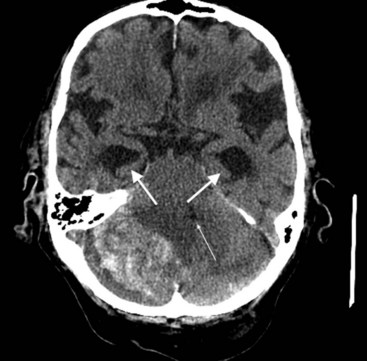
FIGURE 20-14 This computed tomography (CT) scan shows a large white mass lesion in the posterior fossa. The lesion is a cerebellar hemorrhage that has compressed and shifted the fourth ventricle (small arrow), blocking cerebrospinal fluid passage. The resulting obstructive hydrocephalus has caused dilation of the temporal horns of the lateral ventricles (arrows).
The administration of an intravenous contrast solution during CT increases the density of blood-filled structures and whitens their image. This technique, contrast enhancement, highlights vascular structures, such as arteriovenous malformations (AVMs), glioblastomas, and membranes surrounding chronic subdural hematomas and cystic lesions (Fig. 20-15).
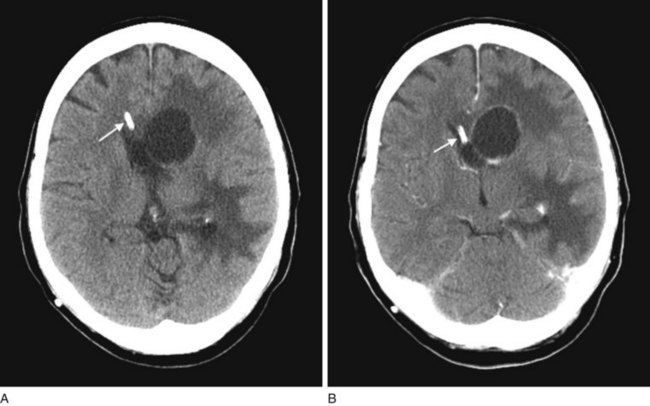
FIGURE 20-15 Computed tomography (CT) scan before (A) and during (B) infusion of contrast material highlights an abscess in a patient’s left frontal region. Disruption of the blood–brain barrier allows the contrast material to concentrate in the lesion’s membranes. In both images, a ventricular catheter can be visualized (arrow), because the catheter is designed to be radio-opaque on CT (with or without contrast).