27 From the initial three-column model proposed by Denis, the biomechanical model of spinal stability continues to be further elucidated. A growing body of research, especially in the past decade, has highlighted the complex and interconnected role played by the osseous, ligamentous, muscular, and soft tissue components of the spine. Injury to any of these structures has the potential to cause instability and ultimately failure. With the advancement of imaging modalities the interplay between these components in both the degenerative and traumatic setting is becoming more understood with paramount implications for treatment. In particular, the importance of the integrity of the collective posterior ligamentous complex (PLC) and how it serves as more than just a posterior tension band, is assisting in the diagnosis and management of spinal degeneration and trauma. The PLC consists of the supraspinous ligament (SSL), interspinous ligament (ISL), ligamentum flavum (LF), and facet joint capsules. The integrity of this complex protects the spine from excessive flexion, rotation, translation, and distraction. These structures act in a complex and interconnected manner to offer restraints to deformation (Table 27.1). The ISL is a thin membranous ligament that consists of dense parallel collagen and elastic fibers. The ISL connects adjoining spinous processes and has insertions from the root to the apex of each process. The fibers interdigitate with the LF ventrally and the SSL dorsally. In a recent cadaveric study of 34 fresh cadavers, Scapinelli et al demonstrated that the ISL complex is composed of three unique sections with their own respective patterns.1 The ventral one third of the ISL is formed by fibers that originate from the LF and anterior margin of the caudal spinous process and insert into the inferior margin of the cranial spinous process. The ventral fibers coarse in a curved manner with a posterior-inferior concavity and have a greater predominance of elastic fibers where the ligament merges with the LF. The middle portion is biomechanically the most important, with fiber orientation being in an italic S shape. The dorsal fibers are obliquely oriented and converge with the SSL. The ISL is minimally developed in the cervical region and becomes more stout and quadrilateral as it descends into the thoracic and lumbar regions. The authors performed histological staining with S100 and demonstrated rich innervation of small nerve fibers most notable in the dorsal portions of the ISL.1 This finding of increased innervation of the dorsal ISL is in agreement with prior investigations. Yahia and others identified Ruffini and Pacini corpuscles in the ISL that most likely serve as proprioception for mechanical loading, stretch, and protection from excessive motion.2 These findings suggest that the ISL, in addition to serving as a tension-resistant structure, has a vital role in proprioception and coordination of movements between the paravertebral and abdominal muscles.3–5 The SSL is a myofascial fibrous cord that links the apices of adjacent spinous process from the seventh cervical vertebra to the sacrum. The ligament thickens and broadens as it descends. Recent cadaveric studies by Johnson and Zhang support the description that the SSL is formed from spinal attachments of the trapezius, rhomboideus, splenius cervicis, longissimus thoracis and multifidis muscle tendons, and aponeuroses along the thoracic and lumbar spine.6 Immunohistochemical studies have documented that the SSL has a significant amount of nerve bundles, individual axons, and free nerve endings also suggesting a role in proprioception and coordination.7 The LF connects the lamina of adjacent vertebra and consists of a high percentage of elastic fibers (up to 80%) in the setting of thick collagen fibers and elaunin fibers.8 This elastic fiber makeup assists in return to neutral after flexion-extension and prevents buckling of the LF into the canal during extension exercises. In addition to the highly elastic makeup, the LF is unique in that it is void of nerve bundles and free nerve endings as are present in the other components of the PLC.7 It is overly simplistic to describe the components of the PLC as unique entities. Numerous biomechanical studies have demonstrated that the components of the PLC are intimately related, and the stability offered is a synergistic interplay between the ligaments.9 In an in vitro biomechanical study, Dumas et al9 demonstrated that cutting the interconnections between the SSL and the ISL resulted in a 40% reduction in stiffness. In a porcine model, Gillespie and Dickey showed that the supraspinous/interspinous ligament complex contributes 35.9% (SD 11.2%) to resisting peak flexion moment.10 The authors observed that the facet joint capsules resisted 14.2% (SD 8.0%) and that the LF resisted 25% of the peak extension moment. The main restraints to extension in this study were the facet joint capsules (72%) and the inter-vertebral disks in concert with the longitudinal ligaments. The ISL/SSL complex is a tension-restraint structure with a unique histological fiber arrangement that is crucial for the effectiveness of the construction.11 Scapinelli hypothesized that the S-shaped, elastic poor fibers of the middle portion of the ISL become more rectilinear in the first phases of flexion. As flexion increases these fibers become stretched and then taught, resisting further flexion.1 This constraint to flexion can only be overcome by violent mechanisms that result in rupture of the both the SSL and the ISL. The stability of the posterior human spine is dependent not only on the ligamentous structures but also on the para-spinal and abdominal muscles that provide a restorative force during loading. In a biomechanical study of a cadaver spine dissected from its muscular attachments Lucas and Bressler demonstrated that the spine begins to fail when loaded with 2 kg of weight.12 From this and other studies it is clear that coactivation of anterior and posterior spinal musculature provides a key component to the overall stability. Solomonow and others performed an intraoperative human study helping identify a posteroligamentous mechanoreceptor–paraspinal muscle reflex arc.13 They found that stimulation of mechanoreceptors in the SSL activated multifidus muscles up to three levels above and below the site of stimulation. This activation acts to stabilize the motion segment of the spine and thus describes the multifidus as an “active ligament” that alters its tension in reaction to the forces sensed by the posterior ligaments. This augmentation to spinal stability could have clinical significance in numerous settings ranging from disruption in trauma to surgical planning. It may also help explain surprising stability in traumatic spines that are ligamentously disrupted. The use of magnetic resonance imaging (MRI) modalities may offer some insight into the integrity of the paraspinal muscles; however, the clinical use of the information will take further studies to elucidate. In the evaluation of the acutely injured spine, numerous modalities can be informative (Table 27.2). MRI modalities are capable of providing a vast amount of information regarding the integrity of the ligamentous structures of the spine. Due to the uniformly high collagen content, the supraspinous and interspinsous ligaments appear as a low signal intensity (dark) on T1 and T2 MRI sequences.14 The LF appears as an intermediate signal, due to its 80% elastin content. Injury to the ligamentous structures is often visualized as a disruption in the normally dark ligament due to a discrete fluid level at the zone of injury. This appears as a clear disruption of the SSL that appears as a black stripe on T1- or T2-weighted sequences. The evaluation of the soft tissues in the trauma setting can be further enhanced by elimination of the fat signal in T2-weighted images. This can be accomplished by either fat saturation (FAT SAT) techniques or short tau inversion recovery (STIR) imaging. FAT SAT T2 images utilize frequency selective suppression of the fat signal by taking advantage of the different resonance frequencies of fat and water protons. This allows better visualization of acute edema without interference from native fat stores. STIR imaging applies an electromagnetic pulse that inverts the magnetization of a tissue. Fatty tissue has a shorter recovery time, and this can be used to eliminate the fat signal. Eliminating the fat signal allows an improvement in the specificity of detecting ligamentous injury on T2 sequences.15,16 Table 27.2 Radiographic Findings of Injury to the Posterior Ligamentous Complex
Magnetic Resonance Imaging Findings of Posterior Ligamentous Injury
Radiographic Modality | Radiographic Finding |
---|---|
MRI | Disruption/discontinuity of the black stripe on T1 and T2 |
MRI | Increased signal in the ISL on T2 |
MRI | Posterior edema on STIR/FAT SAT |
MRI | Increased fluid in facets T2/STIR/FAT SAT |
XR/CT | Focal kyphosis and facet diastasis |
XR/CT | Greater than 20% widening of interspinous distance |
XR/CT | Avulsion fracture of contiguous spinous processes |
XR/CT | Greater than 50% compression fracture of anterior column |
XR/CT | Vertebral body translation |
Abbreviations: CT, computed tomography; FAT SAT, fat saturation; ISL, interspinous ligament; MRI, magnetic resonance imaging; STIR, short tau inversion recovery; XR, x-ray.
MRI of the acutely injured spine is most effective with a high field strength system with at least 1.5 tesla (T), which allows for increased resolution by increasing the signal-to-noise ratio. Higher-strength magnets also allow for shorter imaging times that can be crucial in an acutely traumatized patient.17 The majority of studies concerning MRI use in the detection of PLC injuries use at least a 1.5 T magnet with a slice thickness of 3 mm.
The role of MRI in evaluating the injured spine has taken on a new role as we have begun to understand the importance of the PLC and its role in spinal stability. This chapter evaluates the (1) MRI findings and correlation with injury to the PLC components and (2) effect of PLC integrity on decision making in the injured spine. To address these questions we have performed a comprehensive review of the literature to determine the best evidence available on each of these topics. The search included Med-line, Embase, and the Cochrane Controlled Trials Registry. The references obtained from this search were reviewed for additional articles. A search for the term “posterior ligamentous complex or PLC” returned 41 articles. A search for the term “magnetic resonance imaging” returned 232,724. When these were combined the search returned 19 articles.
MRI Findings and Correlation with Injury to the Posterior Ligamentous Complex Components in the Thoracolumbar Spine
Level I Data
There are no level 1 data published regarding this topic.
Level II Data
Lee and coworkers performed one of the better-designed studies correlating MRI findings with intraoperative inspection of the PLC. This level II prospective study compared blinded MRI reads, physical exam, and plain radiographs to intraoperative findings, which served as the gold standard to diagnosing PLC injury.15
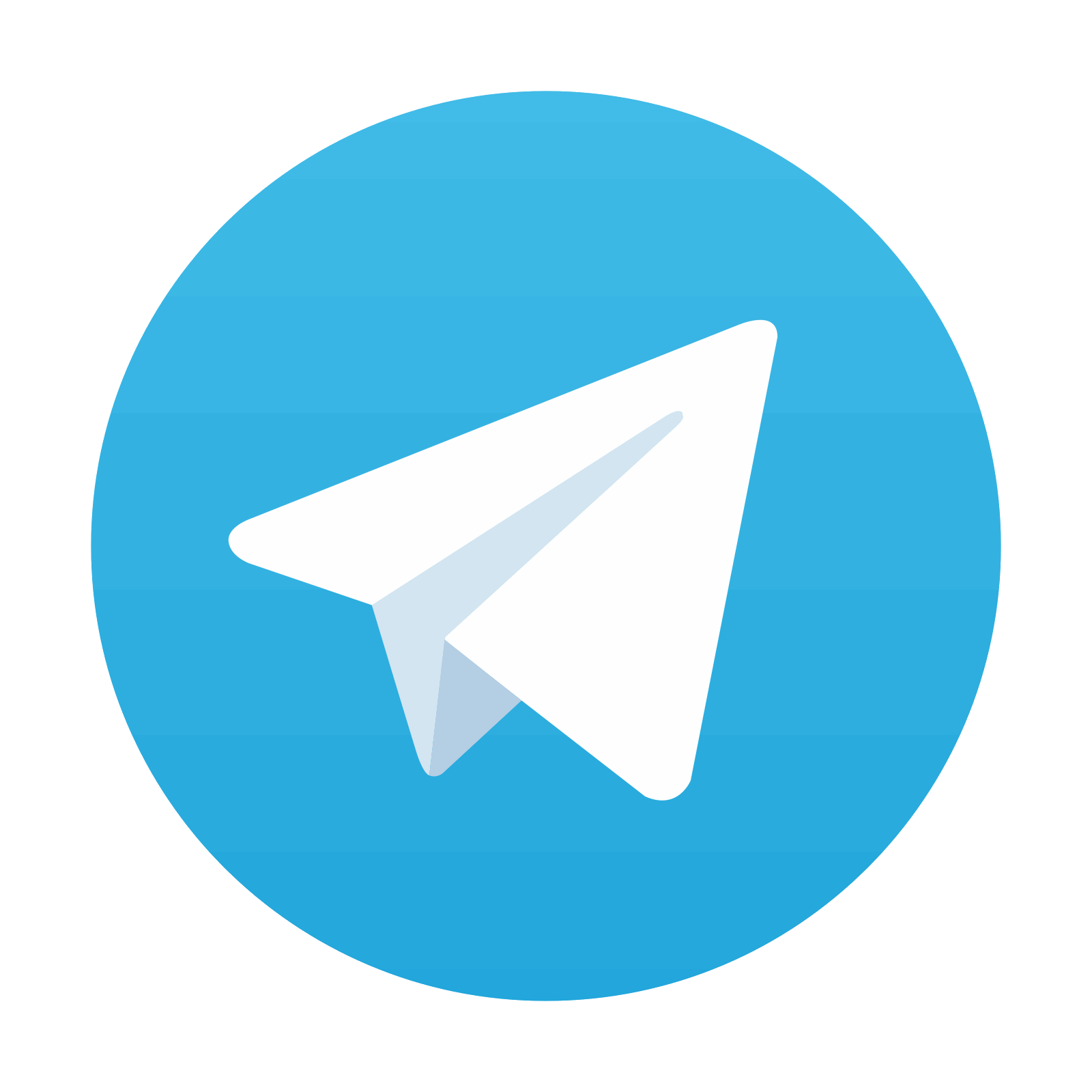
Stay updated, free articles. Join our Telegram channel

Full access? Get Clinical Tree
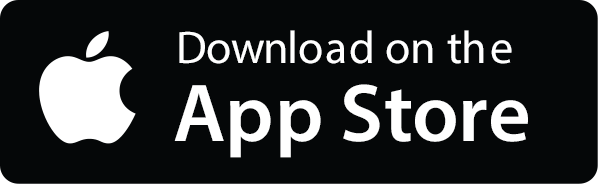
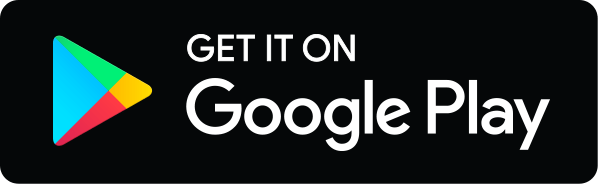