Fig. 19.1
Cystoscopes of 1920s vs. endoscopes of 2000s. (a) A collection of cystoscopes from 1923 (Image courtesy of Dr J.T. Goodrich, from his private collection) (b) ‘Ventriculoscope’. Neuroendoscope that was commercially available from Aesculap, until the mid-2000s (Aesculap, Tuttlingen, Germany)
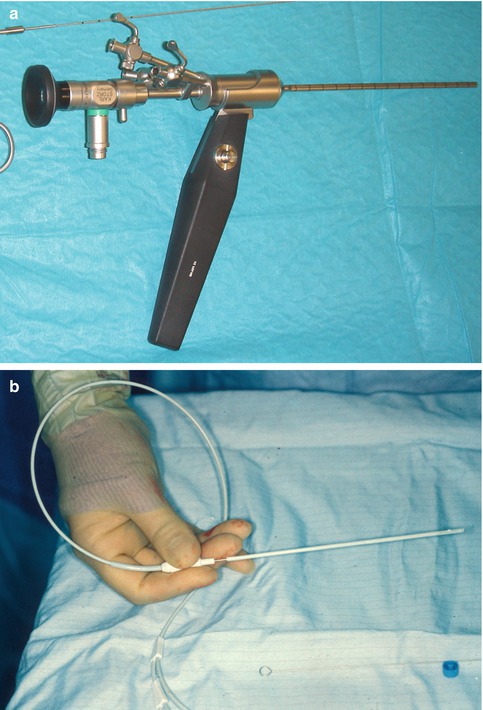
Fig. 19.2
Narrow neuroendoscopes (suitable for paediatric work). (a) Storz Oi endoscope system with external diameter 4 × 2 mm (Storz, Tuttlingen, Germany) (b) Neuropen, 1 mm fibre-optic endoscope in a rigid tube designed to go inside the lumen of a CSF shunt ventricular catheter with ‘fish-mouth’ opening, to aid visualisation of the final position of its tip
One small but important change has been the angle of the viewpiece. Originally in continuation to the long axis of the rod lens (straight vision), in many endoscopes today the viewpiece is at an angle to it with the use of a coupling prism (angled vision) (Fig. 19.3), giving arguably better handling of the whole system.
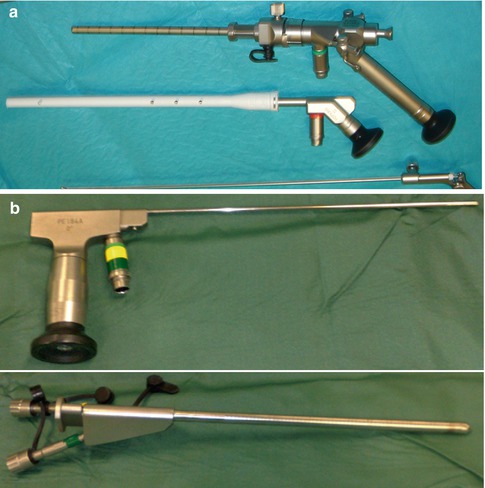
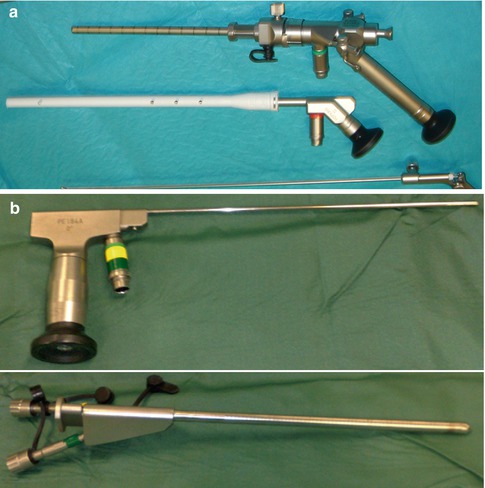
Fig. 19.3
Endoscopes with the viewpiece at an angle to the rod lens. (a) Storz Schroeder LOTTA endoscope system with the viewpiece at 45° to the rod lens. (b) Aesculap MINOP endoscope system with the viewpiece at 90° to the rod lens
The light available at the operative field at the tip of the endoscope has improved significantly over the years with the advent of fibre-optic cables that ‘conduct’ the light from the ‘cold’ light source to the endoscope. The resulting improved illumination has made significant difference to the operating surgeon and is one of the main advantages of the endoscope over the microscope.
The biggest improvement though has been on the camera system. In the 1920s, and even in the reinvention of endoscopy in the 1980s, the surgeon had to look directly through the eyepiece at the end of the rod lens (direct vision). From the early 1990s there is a digital camera coupled to it (vision by proxy), and this has improved dramatically the quality of the image and the handling and ease of use of the whole endoscope system. The technological advance of the digital cameras, which goes hand in hand with the progress of computers and electronics, has made a dramatic change in neuroendoscopy [18]. Further improvement is expected to come from miniaturisation of the camera.
The development of the flexible endoscope has been less dramatic. Flexible endoscopes borrowed technology from the gastroscopes using flexible optical fibres, but their application in the small narrow confines of the brain has not been very successful. Their picture quality is inferior to the rod lens rigid endoscopes, their manoeuvrability is limited and not user friendly, and they have only one side port for all uses (Fig. 19.4). They were in vogue in the early 1990s. Although they are still used by many neurosurgeons, overall it would be fair to say that they have fallen out of favour and certainly they have not been developed any further. Flexible endoscopes available in the market today are more than a decade old designs.
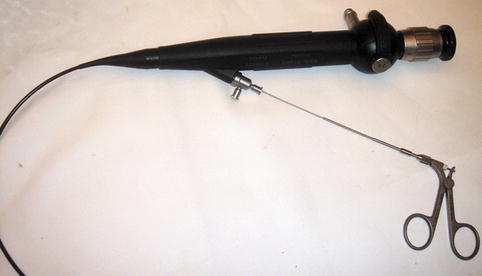
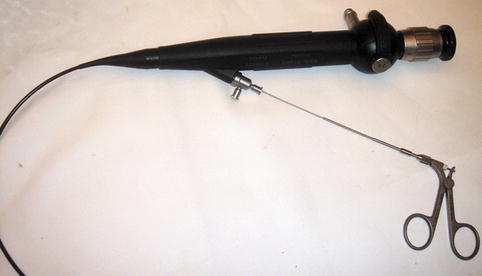
Fig. 19.4
Flexible fibre-optic neuroendoscope from Storz, with one working channel
Another field of progress has been the development of purpose-design surgical instruments for the neuroendoscopes (Fig. 19.5). The availability of suitable monopolar and bipolar diathermies can provide haemostatic control when working in the ventricular system. It has to be said though that this is limited in comparison to the microsurgical technique, and, often, intraoperative haemorrhage during a ventricular procedure leads to the premature ending of the operation. Equally, suction through the endoscope is of limited power. In contrast, haemorrhage during transnasal skull base work is easier to control and has much less significance as it takes place in an open space extracranially. It is rare to have to abandon a transphenoidal endoscopic hypophysectomy because of bleeding, unless the intracavernous part of the internal carotid artery has been injured. The development of other equipment such as scissors, biopsy forceps and probes of various types gives the neuroendoscopist the ability to biopsy tumours or even remove them if they are not haemorrhagic (e.g. colloid cysts), divide adhesions and open cysts and in general perform a much wider spectrum of procedures, in comparison to what was possible 30 years ago [4]. It should be said though that the endoscopic biopsy forceps have small ‘cup’ and can only yield small samples, with a corresponding risk of false histology results.
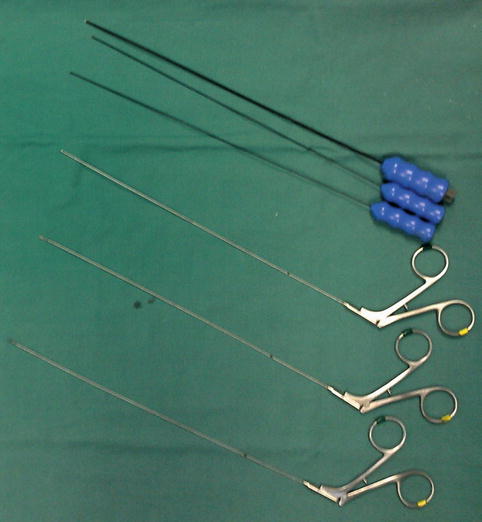
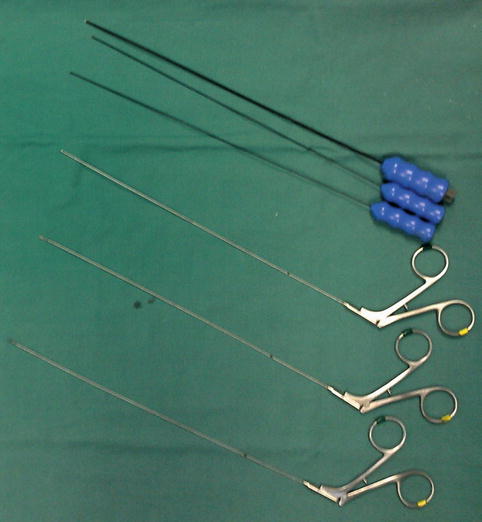
Fig. 19.5
Instruments for neuroendoscopes. (Top three) Bipolar diathermy for the Aesculap MINOP endoscope. (Bottom three) Biopsy forceps and microscissors used for ventricular surgery
A significant innovation, which has not materialised fully as yet, is the integration of the neuroendoscopes with image guidance systems (Fig. 19.6). This gives increased abilities to perform tasks that otherwise would not be attempted, for fear of causing surgical damage. Multiloculated hydrocephalus is easier treated endoscopically with image guidance, which allows confidence of access in the presence of distorted ventricular anatomy. Equally, for extended skull base approaches image guidance is of paramount importance [13]. Further improvement is likely to come in this field as commercial image-guided systems develop further.
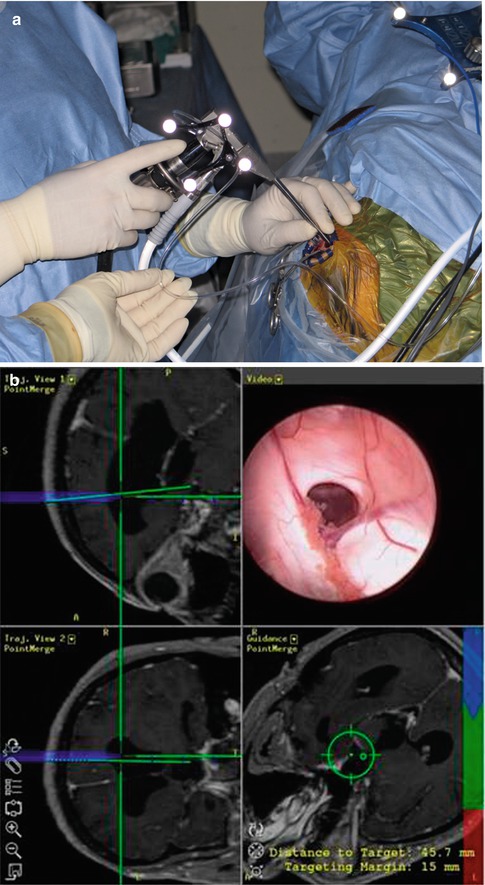
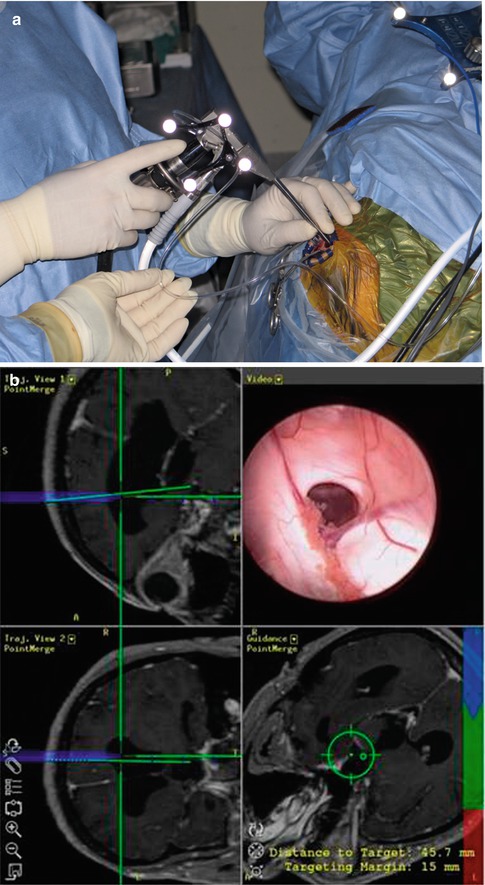
Fig. 19.6
Aesculap MINOP endoscope used in conjunction with the Brainlab neuronavigation system. (a) Reflective spheres attached to the endoscope make it “visible” by the cameras of the neuronavigation system. (b) Computer screen showing the simultaneous projection of the endoscope image and the reconstructed MR images annotated with the superimposed trajectory of the neuroendoscope, calculated by the neuronavigation software (Photograph courtesy of Dr A. Grotenhuis) (Brainlab, Munich, Germany)
New developments such as robotic and 3D endoscopes are not in wide use yet; hence, one cannot comment on their contribution to neuroendoscopy. They are considered as the next frontiers of progress [18].
19.7 Ergonomic Shortcomings of Neuroendoscopic Equipment
In its basic form the endoscope is ergonomically a fulcrum with disadvantageous biomechanical design from the operator’s point of view (Fig. 19.7a). If one examines the use of the endoscope in a ventricular operation (e.g. ETV), the area of interest is approximately 1 cm from the tip of the rod lens, where only a few millimetres of movement is taking place throughout the surgical procedure. The corner of the fulcrum is at the point where the endoscope enters the skull through the burr hole. The part inside the head typically is a few centimetres (6–7 cm). On the other end of the fulcrum, 15–20 cm away from the corner of the fulcrum, a heavy camera lies ‘hanging’, reinforced by the traction that the camera and light cables exert. The operator is obliged to perform very fine manoeuvres of this heavy and ergonomically disadvantageous tail end in order to manipulate and move the front end for a few millimetres, and the region of the third ventricle is not forgiving at all. The avoidance of technical disaster is only a testament of how adaptable humans are, as they can learn very delicate and awkward manoeuvres and perform them without thinking. Certainly, if an engineer was requested to design a new endoscope today from a ‘clean sheet’ (‘tabula rasa’) perspective, ignoring all the technological inheritance of the past, it is unlikely that he would design such a difficult-to-manoeuvre ergonomically disadvantageous system.