Acute spinal cord injury (SCI) is associated with widespread disturbances not only affecting neurologic function but also leading to hemodynamic instability and respiratory failure. Traumatic SCI rarely occurs in isolation, and frequently is accompanied by trauma to other organ systems. Management of individuals with SCI is complex, requiring aggressive monitoring and prompt treatment when complications arise. Typically this level of care is provided in the neurocritical care unit. This article reviews the pathophysiology of the neurologic, cardiovascular, and pulmonary derangements following traumatic SCI and their management in the critical care setting.
Key points
- •
Traumatic spinal cord injury (SCI) is frequently accompanied by multiple injuries and widespread derangements requiring aggressive monitoring and management in the intensive care unit.
- •
Neuroprotective therapies are designed to minimize further neurologic deterioration. Several pharmacologic agents and hypothermia are currently under investigation.
- •
Cardiovascular complications including hypotension and arrhythmias are not uncommon, and represent an important source of morbidity following acute SCI.
- •
SCI may lead to absent or inefficient respiratory mechanics, atelectasis, pneumonia, and pulmonary dysfunction requiring intubation and mechanical ventilation.
- •
Prophylaxis and surveillance of deep venous thrombosis is paramount in individuals with SCI, and should be instituted within 72 hours of injury.
Introduction
Acute spinal cord injury (SCI) is often devastating, and imposes significant emotional and economic costs to the individual and society. The morbidity and sequelae are severe and frequently fatal. Mortality at the time of injury is 48% to 79%, with another 4.4% to 16% of deaths occurring before hospital discharge. Traumatic SCI occurs with an annual incidence of approximately 15 to 40 cases per million in the United States. Estimates of prevalence are 236,000 to 327,000. SCI disproportionately affects young adults. The mean age at injury is 41 years, although two-thirds of individuals are younger than 30. Motor vehicle accidents account for 40% to 50% of SCI followed by falls (20%), violence (14%), and recreational and work-related activities. The epidemiology and mechanism of SCI have important implications on preventive measures, the type of injury sustained, and subsequent management in the critical care unit.
Evaluation and management of acute SCI is complicated by broad pathophysiologic derangements that require timely identification and intervention. Respiratory insufficiency or other pulmonary dysfunction, systemic hypotension, cardiac arrhythmias, and delayed neurologic decline are well-described phenomena following SCI. Additional systemic injuries are commonplace, with 20% to 57% of those with SCI having other significant injuries typically involving the head or chest. Isolated SCIs are reported to occur in only 20% of individuals. The management of these patients in the intensive care unit is frequently directed by treatment of concurrent injuries. The majority of traumatic SCIs (55%) involve the cervical spine. The last 3 decades have witnessed an increase in the proportion of cervical spine injuries. Specifically this has been injury to C1 to C4, nearly tripling the number of individuals requiring intubation and ventilator dependence from 1.4% in 1970 to 4.6% in 2004. During this same period there has been a concomitant decrease in the number of complete SCIs from more than 60% to 45%. This decline is multifactorial and reflects improvements in prehospital resuscitation and retrieval, immobilization, and motor vehicle restraint and safety devices. Advances in monitoring and medical management also hold an important role in limiting secondary injury from hypoxia or hypotension. Several studies have demonstrated improved morbidity and mortality with monitoring and aggressive management of SCI in the critical care setting. The median length of hospital admission following SCI is 67 days, with 11 of those days spent in the acute care setting at a cost of $95,203. This review discusses the potential complications and medical management of acute SCI in the neurocritical care unit.
Introduction
Acute spinal cord injury (SCI) is often devastating, and imposes significant emotional and economic costs to the individual and society. The morbidity and sequelae are severe and frequently fatal. Mortality at the time of injury is 48% to 79%, with another 4.4% to 16% of deaths occurring before hospital discharge. Traumatic SCI occurs with an annual incidence of approximately 15 to 40 cases per million in the United States. Estimates of prevalence are 236,000 to 327,000. SCI disproportionately affects young adults. The mean age at injury is 41 years, although two-thirds of individuals are younger than 30. Motor vehicle accidents account for 40% to 50% of SCI followed by falls (20%), violence (14%), and recreational and work-related activities. The epidemiology and mechanism of SCI have important implications on preventive measures, the type of injury sustained, and subsequent management in the critical care unit.
Evaluation and management of acute SCI is complicated by broad pathophysiologic derangements that require timely identification and intervention. Respiratory insufficiency or other pulmonary dysfunction, systemic hypotension, cardiac arrhythmias, and delayed neurologic decline are well-described phenomena following SCI. Additional systemic injuries are commonplace, with 20% to 57% of those with SCI having other significant injuries typically involving the head or chest. Isolated SCIs are reported to occur in only 20% of individuals. The management of these patients in the intensive care unit is frequently directed by treatment of concurrent injuries. The majority of traumatic SCIs (55%) involve the cervical spine. The last 3 decades have witnessed an increase in the proportion of cervical spine injuries. Specifically this has been injury to C1 to C4, nearly tripling the number of individuals requiring intubation and ventilator dependence from 1.4% in 1970 to 4.6% in 2004. During this same period there has been a concomitant decrease in the number of complete SCIs from more than 60% to 45%. This decline is multifactorial and reflects improvements in prehospital resuscitation and retrieval, immobilization, and motor vehicle restraint and safety devices. Advances in monitoring and medical management also hold an important role in limiting secondary injury from hypoxia or hypotension. Several studies have demonstrated improved morbidity and mortality with monitoring and aggressive management of SCI in the critical care setting. The median length of hospital admission following SCI is 67 days, with 11 of those days spent in the acute care setting at a cost of $95,203. This review discusses the potential complications and medical management of acute SCI in the neurocritical care unit.
Management of neurologic injury
Treatment of acute SCI in the intensive care unit focuses on prevention of secondary injury and neuroprotection. Following an initial insult or trauma producing spinal cord compression, contusion, laceration, or shear, a cascade of pathologic events develops. These abnormalities include loss of normal blood flow in the spine, ischemia, vasospasm, thrombosis, hemorrhage, impaired autoregulation of the microcirculation, and systemic hypotension from neurogenic shock; electrolyte disturbances; neurotransmitter accumulation and excitotoxicity; lipid peroxidation; arachidonic acid production and inflammation; free radical production; edema; apoptosis; and necrosis. Delayed neurologic deterioration is well described, with a reported incidence of 1.8% to 10%, and may be seen from hours to days following the onset of injury. Intensive monitoring and rapid correction of hypotension or hypoxia is essential (see later discussion). Pharmacologic strategies to blunt secondary injury have also been used, perhaps the most well described being methylprednisolone.
Glucocorticoids offer neuroprotection by increasing cell membrane integrity, augmenting spinal cord perfusion, inhibiting endogenous endorphin release, and decreasing inflammation and free radical production, as well as by limiting vasogenic edema through stabilization of the blood–spinal cord barrier. The largest clinical trials investigating the efficacy of methylprednisolone are the 3 multicenter randomized NASCIS (National Acute Spinal Cord Injury Study) trials. The first of these studies (NASCIS I) compared motor and sensory scores in individuals with SCI following administration of either a 100-mg or 1000-mg bolus of methylprednisolone followed by a daily dose for 10 days. There was no placebo, and comparison of the outcomes at 6 weeks and 6 months showed no difference. With the NASCIS II study, the dose of methylprednisolone was increased and individuals with SCI were randomized to 3 different treatments: (1) methylprednisolone (30 mg/kg bolus followed by 5.4 mg/kg/h for 23 hours); (2) the opioid antagonist naloxone (5.4 mg/kg bolus followed by 4.0 mg/kg/h for 23 hours); and (3) placebo. Through post hoc analysis the investigators reported improved motor and sensory scores at 6 months if methylprednisolone was administered within 8 hours of injury. This benefit was observed for both complete and incomplete injuries, but was not present in patients receiving steroids more than 8 hours following injury, naloxone, or placebo. A third trial (NASCIS III) randomized patients to receive methylprednisolone for 24 or 48 hours or the antioxidant tirilizad mesylate for 48 hours after a bolus of methylprednisolone. Tirilizad mesylate impairs lipid peroxidation but does not contain the steroid moiety to interact with and activate glucocorticoid receptors, and is thus thought to avoid the adverse effects of methylprednisolone. Neurologic outcomes were similar for all treatment groups if initiated within 3 hours of injury. When comparing individuals treated between 3 and 8 hours following injury, motor scores and functional measures were improved at 6 weeks and 6 months in participants treated with methylprednisolone for 48 hours. The neurologic outcomes were equivocal for subjects who received 24 hours of methylprednisolone and 48 hours of tirilizad mesylate. No difference in functional outcome scores was seen in the 3 groups at 1 year.
Several limitations to the NASCIS trials exist. The studies excluded pediatric SCI, penetrating injury, or cauda equina injuries, and do not specifically address these entities. Administration of methylprednisolone was associated with increased incidence of pulmonary embolism and pneumonia, wound infection, sepsis, and gastrointestinal complications in NASCIS II and III. Other reports have also noted increased length of admission and altered immune function. Several methodological flaws have also been identified, including a post hoc versus prospectively defined time course (ie, 8 hours), inclusion of patients with minimal deficits and lesions below T12, use of right-sided motor scores only, lack of functional measures, and no standardization with respect to surgical or medical treatment. For these reasons, the use of methylprednisolone in SCI is controversial. The Cochrane database offers the NASCIS experience as a recommendation for the use of methylprednisolone within 8 hours of injury. Guidelines published by the American Association of Neurological Surgeons (AANS) and the Congress of Neurological Surgeons (CNS) recently modified the recommendations against the use of methylprednisolone in the management of spinal cord injury.
Other pharmacologic agents have also been studied in the setting of acute SCI. In laboratory studies, GM-1 ganglioside has been associated with increased neural plasticity following injury, diminished excitotoxicity, and reduced apoptosis. A prospective study randomizing 37 individuals with SCI to placebo or GM-1 ganglioside demonstrated improved Frankel and ASIA (American Spinal Injury Association) scores at 1-year follow-up in participants who received 100 mg of GM-1 for 30 days. This study served as the impetus for a large multicenter randomized trial examining the effects of low-dose GM-1, high-dose GM-1, and placebo. All subjects received methylprednisolone as described in the NASCIS II study. In this large study of 797 patients, there was no significant difference in neurologic outcome between the treatment groups, limiting the use of GM-1. Opioid antagonists such as naloxone, glutamate antagonists, nimodipine, other anti-inflammatories, and erythropoietin have all garnered interest following optimistic findings in laboratory models.
In addition to pharmacologic methods of neuroprotection, there is increasing interest in therapeutic hypothermia. In laboratory models, moderate hypothermia has led to alterations in apoptosis as well as reduced mitochondrial dysfunction, metabolic demand, cell membrane injury, and inflammation. In rodents, prompt cooling following induced SCI offered improved motor outcomes, and the use of hypothermia is reported to decrease SCI following aortic cross-clamping. Fourteen patients with complete SCI underwent moderate hypothermia (33°C) for 48 hours in a study by Levi and colleagues in 2009. At median follow-up of 12 months, 6 individuals (43%) had neurologic improvement. The most frequent complications were pulmonary (pneumonia or atelectasis), but occurred at a rate similar to that of controls. Another study had similar results following endovascular cooling in 35 individuals with complete cervical SCI. The duration of hypothermic therapy was 48 hours, and 15 (43%) had improvement based on the International Standards for Neurological Classification of Spinal Cord Injury scale. Following completion of phase I trials, large multicenter studies are currently being conducted (see clinicaltrials.gov ).
Cardiovascular complications
Severe SCI is frequently associated with hemodynamic instability. Disruption of sympathetic fibers through injury to the cervical or high-thoracic (>T6) spinal cord produces hypotension and cardiac arrhythmias. Fifty percent to 90% of individuals with acute cervical SCI require aggressive fluid resuscitation or initiation of vasopressors to maintain a mean arterial pressure greater than 80 mm Hg. Typically the degree of cardiac instability is related to the severity of the SCI. At autopsy, individuals with cervical SCI developing significant hypotension, arrhythmia, or autonomic dysreflexia had increased degeneration compared with those without cardiac instability. In addition, these individuals had fewer preserved axons within the lateral funiculus. Descending sympathetic fibers originating in the hypothalamus or brainstem are located within the intermediolateral and dorsolateral aspects of the lateral funiculus. Injury leads to loss of sympathetic outflow and unopposed parasympathetic tone with decreased cardiac contractility, heart rate, and vasoconstrictor tone.
The hypotension and neurogenic shock following acute SCI is a distributive process resulting from loss of vasoconstrictor tone in peripheral arterioles and pooling of blood within the peripheral vasculature. Initial attempts at correction should include volume resuscitation with intravenous fluids. Administration of fluids transiently increases venous return. However, with disruption of sympathetic innervation to the heart and predominance of parasympathetic tone, cardiac output remains low because of impaired cardiac contractility and decreased heart rate. If after 1 to 2 L of intravenous fluids the blood pressure has not improved, vasopressor therapy may be indicated. Vasoactive agents with α-adrenergic and β-adrenergic effects, such as dopamine and norepinephrine, increase vasoconstrictor tone and chronotropic support to the heart. Vasopressors with exclusive α-adrenergic function have no chronotropic effect and are of limited use in neurogenic shock. Furthermore, phenylephrine frequently produces a reflex bradycardia. Throughout these interventions frequent monitoring of blood pressure, heart rate, urine output, and resolution of acidosis are vital. Echocardiography offers the ability to efficiently assess cardiac output and filling. In select situations, invasive monitoring with a pulmonary artery catheter assists in treatment by measuring cardiac output and peripheral vascular resistance, although this has largely been replaced by the echocardiogram.
In a study by Levi and colleagues, 82% of individuals with incomplete cervical SCI required vasopressor therapy for an average of 5.7 days following injury to maintain a mean arterial pressure (MAP) of greater than 90 mm Hg. Another study correlated level and severity of spinal injury with the need for vasopressors: complete cervical SCI 90%, incomplete cervical SCI 52%, complete thoracic SCI 33%, and incomplete thoracic 25%. Management of hypotension following SCI is commonplace in the neurocritical care unit. Throughout the resuscitation efforts it is important to continually reassess and ensure that other events such as hemorrhage, tension pneumothorax, or cardiac tamponade are not etiologic.
Although avoidance of systemic hypotension and adequate tissue perfusion are well accepted, blood pressure targets are not as clearly defined. In addition, it is difficult to accurately assess spinal cord perfusion. There are several uncontrolled studies describing improved neurologic outcomes with a MAP greater than 85 to 90 mm Hg. Vale and colleagues used a MAP of 85 mm Hg to direct aggressive fluid resuscitation and vasopressors for 7 days following injury. The small series reported improved outcomes, but the MAP in this study was selected arbitrarily. The AANS/CNS guidelines recommend maintenance of a MAP of between 85 and 90 mm Hg for the first 7 days as an option for treatment.
Loss of sympathetic innervation with unopposed vagal tone produces frequent and often severe cardiac arrhythmias such as bradycardia and, less often, supraventricular tachycardia or ventricular tachycardia. Estimates of cardiac arrest following cervical SCI are as high as 15%. The arrhythmias typically develop within 3 to 5 days following injury and become less pronounced at day 14. The severity of cardiac instability is influenced by the degree of spinal injury as well as other factors such as hypoxia, endotracheal suctioning, and physical stimulation. Treatment includes continuous monitoring and, when symptomatic bradycardia develops, oxygen, atropine, inotropes, and aminophylline. These pharmacologic measures are usually effective, but if bradycardia persists patients may require a cardiac pacemaker. The number of individuals with cervical SCI requiring cardiac pacing ranges from 9% to 17%.
Orthostatic hypotension and autonomic dysreflexia are known to occur following SCI. Typically these are seen in the subacute or chronic phase of injury, but may complicate admission to the neurocritical care unit. Orthostatic hypotension is defined as a decrease in systolic blood pressure of greater than 20 mm Hg or diastolic blood pressure greater than 10 mm Hg when transitioning from a supine to upright position. The prevalence of orthostatic hypotension is approximately 80% and 50% for quadriplegics and paraplegics, respectively. Nearly 50% of individuals with cervical SCI will experience symptoms of orthostatic hypotension, and require treatment. This orthostasis results from venous pooling of blood resulting from the loss of vasoconstrictor tone and arterial baroreceptors caudal to the injury level, an ineffective increase in heart rate from unopposed vagal tone, and loss of muscular tone in the lower extremities, impairing venous return to the heart. Treatment measures include volume expansion through fluids, increased salt intake, or fludrocortisone; pressure devices such as abdominal binders; devices supplying electrical stimulation to and contraction of the lower extremity musculature; midodrine, a potent α-agonist; or ephedrine. The most well-described pharmacologic agents are fludrocortisone and midodrine.
Individuals with severe SCI rostral to T6 are subject to autonomic dysreflexia. During these periods noxious or seemingly benign stimulation below the level of injury, such as bowel or bladder distention, leads to rapid and dramatic elevation in blood pressure and heart rate. By definition this is characterized by an elevation in blood pressure greater than 20%, tachycardia, or bradycardia, and is associated with other symptoms of overactive autonomic stimulation such as piloerection, diaphoresis, or flushing. Dysreflexia is seen in up to 90% of individuals with complete tetraplegia but in less than 30% of those with incomplete tetraplegia. Autonomic dysreflexia is typically a chronic complication following SCI. In a study of 58 patients with cervical SCI, however, approximately 3% of subjects exhibited dysreflexic episodes acutely. Prompt recognition and treatment is important in avoiding complications of hypertensive crisis such as intracranial or retinal hemorrhages, seizure, or death.
Treatment of autonomic dysreflexia begins with identification of the offending stimulus. A distended bladder or bowel should be relieved and all other noxious stimuli removed. Pharmacologic interventions designed to shorten the disturbance include nitrates, prostaglandin E 2 , hydralazine, labetalol, or captopril. Prevention of dysreflexic episodes involves avoidance of noxious stimuli. In addition, the use of prazosin or terazosin is described in the literature. Nifedipine had once been used for this purpose but has since been abandoned following reports of deaths associated with this medication.
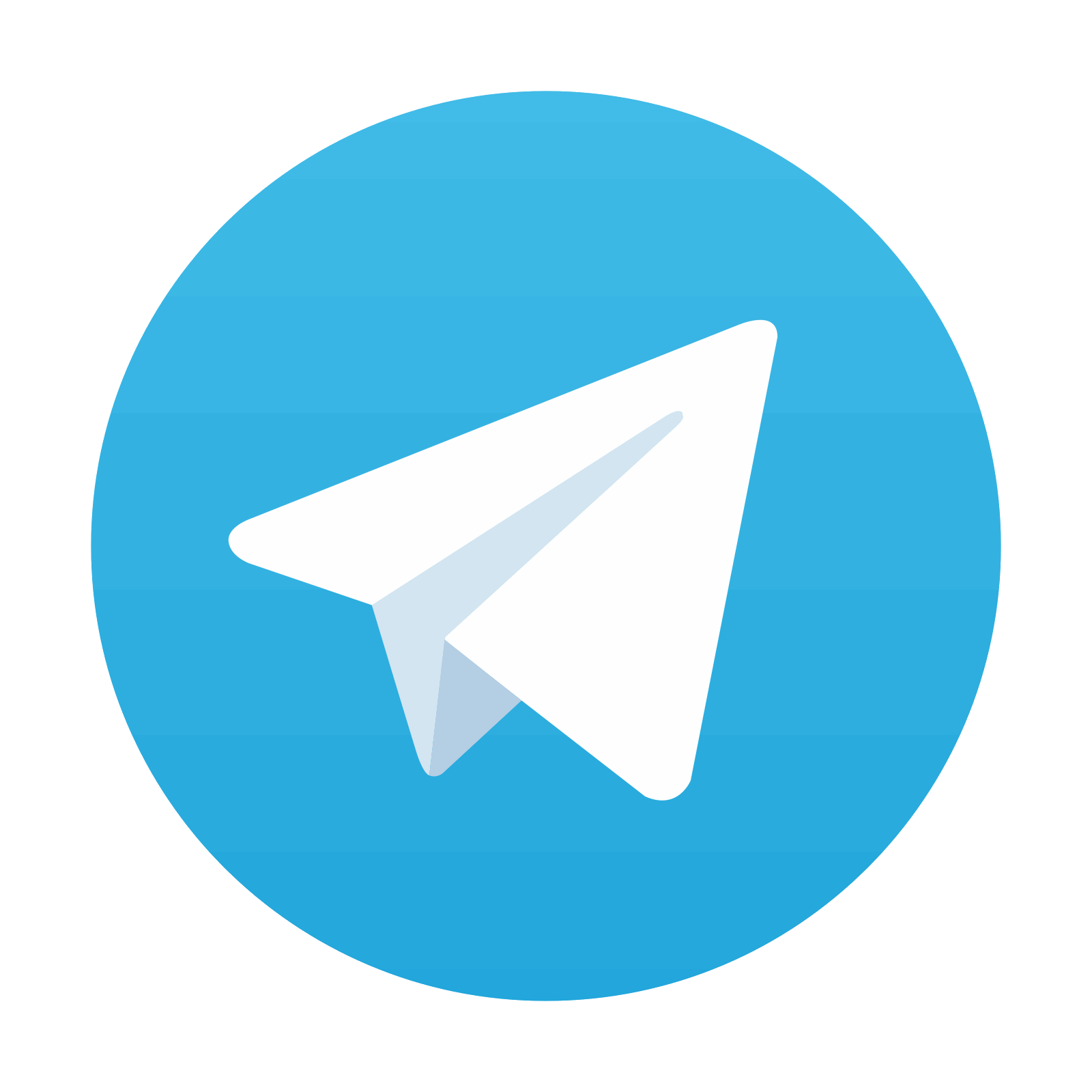
Stay updated, free articles. Join our Telegram channel

Full access? Get Clinical Tree
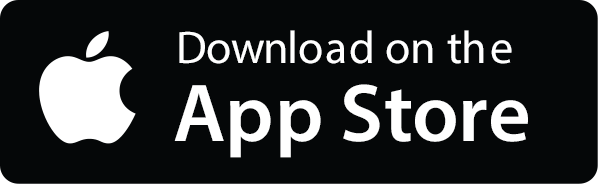
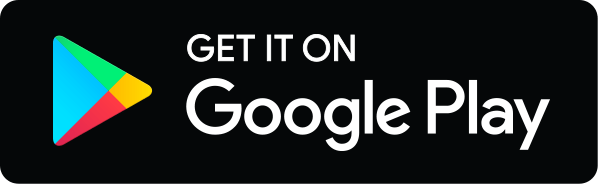