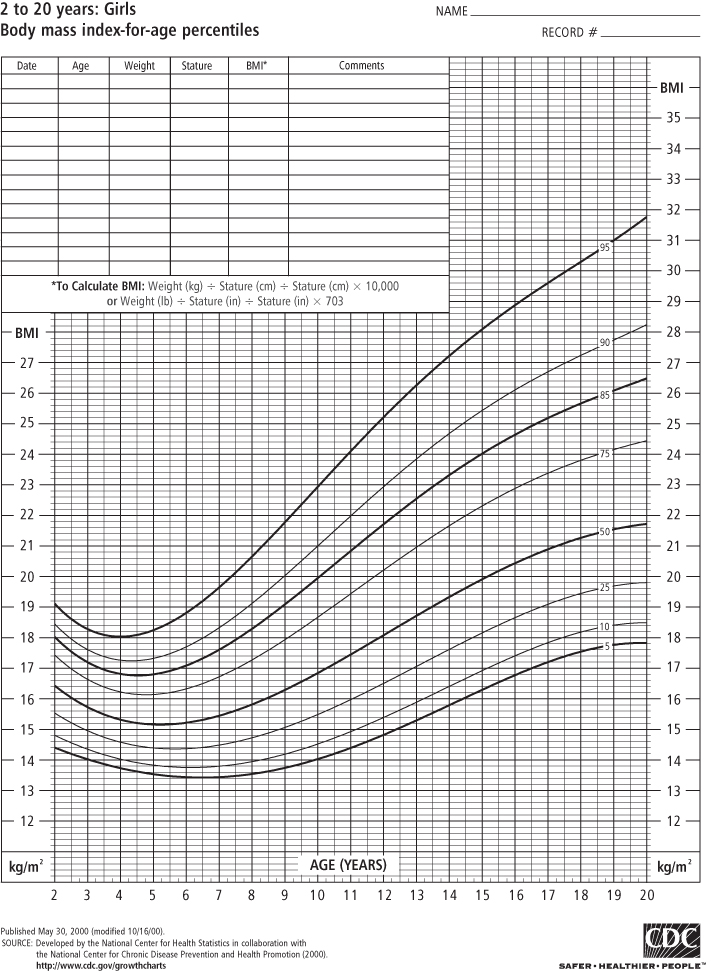
Circumference Measurements
This anthropometric method is based on the principle that differences in the circumference of a body segment can reflect differences in fat mass (FM) and FFM. Circumference measurements are obtained using a flexible tape to measure various parts of the body. These measurements are useful for comparing changes in body size as a result of body weight changes and also to provide information on fat distribution. Circumferences at the waist, hip, and thigh can be used to estimate body fat distribution. Abdominal adiposity, as indicated by waist circumference, is associated with cardiovascular risk factors and with the metabolic syndrome (6). Circumferences may differ depending on the exact location of the measurement. Commonly measured waist sites include: 1) immediately below the lowermost rib, 2) at the narrowest visible abdominal circumference, 3) the midpoint between the lowest rib and iliac crest, and 4) immediately above the iliac crest (7). Waist sites that are identified using an anatomical bony landmark are recommended for greater precision and reproducibility.
The waist/hip ratio is another commonly used index of fat distribution, as it relates to health risk. A ratio of less than 0.90 for men and less than 0.80 for women is considered desirable (8). A high waist/hip ratio indicates that fat is stored in the central part of the body and is suggestive of greater health risks associated with excess fat (e.g., diabetes, heart disease, and high blood pressure). A low waist/hip ratio indicates that fat has been preferentially stored in the femoral-gluteal area (hips), a pattern associated with a lower probability of developing these health problems (9). A limitation of circumference measures in general is that the technician must be well trained to identify bony landmarks and properly align the tape where the circumference measurement is made. Also, circumferences do not distinguish between lean and fat tissue, so the percent fat of muscular subjects could be overestimated by some circumference measurements.
Skinfolds
Skinfold measurements made using special calipers (Figure 13-2) can be used to estimate percent body fat. Skinfolds, in addition to the two layers of skin they include, indicate the thickness of the subcutaneous fat that underlies the skin at a specific site. Skinfolds are obtained at 3–9 standard anatomical sites usually on the right side of the body (subscapular, triceps, biceps, suprailiac, abdomen, etc.). As the subject stands relaxed, the tester pinches a broad fold of the skin at the appropriate site to raise a double layer of skin along with subcutaneous tissue that underlies the skin. The skinfold is picked up firmly between thumb and forefinger of the left hand and pulled away from the underlying muscle. With a few exceptions, the skinfold is pinched in a vertical line. The calipers are then applied 1 cm below the thumb and forefinger, at a right angle to the pinch, and a reading is taken (after 2–3 seconds of caliper pressure) to the nearest 0.5 mm. The mean of several measurements is usually taken.
Figure 13-2 a. Skinfold thickness calipers. b. Measurement of triceps skinfold showing fingers of technician holding the triceps skinfold and caliper jaws placed on skinfold above fingers
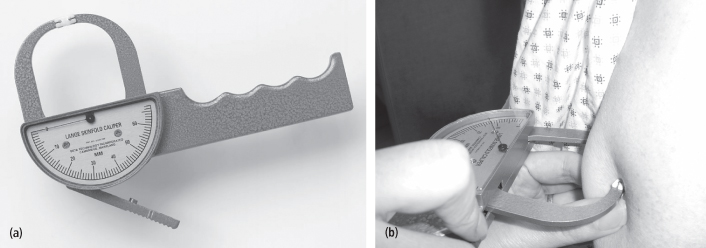
There are numerous empirical equations that relate skinfold thicknesses to body density (10–11). Each equation is specific to the population in which it was developed. Typically, several skinfold measurements are summed and body density is estimated using a regression equation developed with those skinfolds. The value for body density (BD) is transformed to an estimate of percent body fat (%BF) via one of several body composition equations, such as the Siri equation (12):
This equation is based on a set of assumptions about the proportions and densities of water, protein, and mineral in the lean tissue and its resulting average density (see below, “Two-compartment measurement approaches”). The accuracy of the fat values obtained from skinfold measurements depends on how similar the study subjects are to the population used to develop the regression equation that determines body density and also on how closely their lean tissue composition matches that assumed in the Siri equation. However, the thickness of the non-fat layers of the skin may differ by age, race, etc., and can be altered in conditions where body hydration is changed (e.g., pregnancy), affecting the accuracy of the resulting fat estimates. Skinfold thicknesses measured at different sites have been shown to correlate differently with percent body fat and total fat. For example, triceps skinfolds were found to have a better correlation with percent body fat, whereas subscapular skinfolds correlated better with total body fat (13).
The skinfold method is safe. It requires little time to perform and minimal subject participation. Also, it is relatively inexpensive and suitable for large-scale studies. Its primary disadvantages are that the technician must be well trained, good quality calipers must be used, and that mathematical equations, based on several assumptions that may not apply in the target population, must be used to calculate percent body fat.
Two-Compartment Measurement Approaches
The two-compartment (2C) model partitions the body into FFM and FM, and is the most widely used model underlying methods to estimate body composition in adults. The Siri equation is based on a two-compartment model. Behnke’s two-compartment model assumes known and constant proportions of water, protein, and mineral in the FFM (14). It was from these assumed proportions, and the assumed density of each chemical component, that the density of FFM was estimated as 1.10 g/cm3 (15).
When the assumptions that form the basis for the 2C model are not met, such as in aging, pregnancy, maturation, weight reduction in obese people, over- or under-hydration, and in various disease states, body composition estimates derived from this model are less accurate. The 2C model approach is not ideal for measuring FM and FFM in infants and young children, and it does not give information regarding variations in the nutritionally important total body protein and mineral components.
Bioimpedance Analysis
Bioimpedance analysis (BIA) is based on measures of the resistance and reactance of a weak electrical current that is passed through the body. Tissues rich in water and electrolytes offer considerably less resistance to passage of an electric current than does adipose tissue. BIA is based on the principle that the lean tissue of the body is much more electrically conductive than fat tissue due to its higher water and electrolyte content. Thus, the current is easily conducted through the lean compartment. The measured resistance is determined by temperature, the geometry of the conducting material, including its length and cross-sectional area, and its intrinsic resistance to conducting a current (its resistivity, which varies with chemical composition). Thus, the resistance can be related to the total amount of body water or lean tissue with several assumptions about these factors.
BIA is measured with machines that use these assumptions to calculate total body water (TBW) and estimate FFM from the body water. The estimation of FFM from the calculated total body water assumes a constant hydration level (% water) of FFM on the order of 73% (16). Total body fat is then estimated as the difference between total body weight and FFM. The accuracy of body fat estimates based on this measure are considered to be within 3.5–5.0% of the true value when conditions that can affect the total body water estimate, such as ambient temperature, participant hydration status, position of participant, electrode placement, use of appropriate equations, and time since eating and drinking, are regulated (17).
Conventional single-frequency BIA systems transmit and receive electrical signals through electrodes placed on the body. The body is considered to be a single cylinder-shaped form. Single-frequency BIA is most commonly used for assessing TBW and FFM, but is limited in its ability to distinguish the distribution of TBW in its intra- and extracellular compartments. The arm-to-leg electrical pathway approach is widely used. This approach requires that four gel electrodes be placed on the right side of the body, two at upper sites and two at corresponding lower-extremity sites. A single frequency is passed between surface electrodes placed on the right hand and right foot.
The leg-to-leg pressure contact BIA approach has the potential of simplifying impedance measurements by eliminating the need for gel electrodes and measuring weight concurrently with impedance. The electrical current passes between surface electrodes that both feet stand on (foot-to-foot). (18). However, the electric path followed includes only the legs and a part of the abdomen, so differences in fat distribution in the rest of the body, which can be important, are not included in the results.
Multi-frequency BIA allows for the differentiation of TBW into intracellular water (ICW) and extracellular water (ECW) compartments by adding a measurement of resistance at low frequency. This approach is useful not only to describe fluid shifts and fluid balance, but also to explore variations in levels of hydration (19).
Recently, multi-segmental BIA has become available in both single- and multi-frequency systems. The multi-segmental approach assumes that the body is made up of a group of cylinders. The impedance of the left and right arms, the left and right legs, and the total body are measured separately. The summed value for the four limbs is subtracted from the value for the total body to derive the impedance of the trunk. These values are then used to estimate body fat of the various segments as well as total body fat.
The advantages of BIA include its portability, ease of use, relatively low initial cost and cost per use, minimal subject participation requirement, and its safety, thus making it attractive for large-scale studies. BIA is not ideal for circumstances where typical hydration of lean mass may not be as assumed, such as during growth, pregnancy and lactation, weight loss, and marked obesity; it is also not recommended for participants with a pacemaker.
Hydrostatic Weighing
Underwater weighing (UWW), or hydrodensitometry, has traditionally been considered the gold standard for the determination of body composition and is used as a criterion method in validation studies of new body composition assessment methods. The hydrostatic weighing equipment consists of a large tank of water, with the water temperature held constant at approximately 37° C (Figure 13-3). The system has a seat or platform that is attached to a scale. The subject sits on the platform and expels as much air from the lungs as possible during complete submersion under water.
UWW measures body volume by the application of Archimedes’ principle, which states that the volume of an object is equal to the object’s loss of weight in water. Thus, body volume can be determined by measuring the difference between body weight in air and when submerged in water (see Figure 13-9b). Residual lung volume makes a sizable contribution to total body volume (1–2 L); it is therefore essential to correct for residual lung volume, determined prior to or during the underwater weighing procedure, to obtain an accurate body volume estimate.
With known body volume and weight (in air), body density can be calculated as the ratio of the body’s weight (mass) to its volume. Using the Siri equation (12) shown above, percent body fat is calculated from the density. In this approach, the densities of fat (0.9007 g/cm3) and FFM (1.100 g/cm3) are assumed to be the same across all adults, irrespective of age, race/ethnicity, and disease. Body fat estimated by hydrostatic weighing is reproducible to within 2% in consecutive tests (20). Disadvantages of this technique include its requirement for large, complex equipment and for the subject’s total submersion under water, which can be especially difficult for non-swimmers.
Air Plethysmography
Air displacement plethysmography is an alternative to hydrostatic weighing to measure body volume. A commercially available system is the BOD POD (Life Measurement Instruments Inc., Concord, CA), which can be used in persons weighing between 35 kg and 200 kg (Figure 13-4).
This system consists of a front test chamber and a rear reference chamber. The back of the seat in the BOD POD separates the front and rear chambers, providing a common wall between them. Body volume is derived from the ratio of the pressures in the reference and test chambers based on Poisson’s law, which states that volume varies inversely with pressure at a constant temperature (21). With a subject seated in the BOD POD, a vibrating diaphragm in the wall of the chamber causes small changes in the volume of the test chamber. The pressure in each of the two chambers responds immediately to this volume change, while the magnitude of the pressure change indicates the relative size of the chambers. The difference in the air pressure changes when the chamber is occupied can be used to estimate the volume of air the person displaces.
To measure body volume accurately, it is necessary to account for the effects of air trapped in clothing, hair, and lungs, as well as effects related to skin surface area. The measured body volume is corrected for these effects. If trapped air is not adequately controlled, body density can be overestimated, causing an underestimation of percent fat (22). Skin surface area can be estimated if height and weight are known (23).
An infant system (PEA POD Life Measurement Instruments Inc., Concord, CA), with a maximum recommended subject weight of 8 kg, is available for the assessment of body composition in infants. A movable cart houses the reference chamber and calibration volume, and the test chamber is mounted on the cart’s top surface. A volume-perturbing diaphragm is located between the test and reference chambers, and a pneumatic valve (calibration valve) allows the test chamber to be connected to the calibration volume (24) (Figure 13-4).
The advantages of air plethysmography are its speed and relative ease for the subjects compared to hydrostatic weighing. The disadvantages include the need for large and costly equipment and technically trained staff. In addition, the breathing maneuver required for the purpose of predicting lung volume may be difficult for some, claustrophobic persons may be unable to tolerate the chamber, and size limitations exclude from the BODPOD those >200 kg or <35 kg, while the PEAPOD fails to cover those between 8 and 35 kg.
Three-Dimensional Photonic Scanning
The use of a digitized optical method and computer to generate a “3-dimensional photonic scan (3-DPS) image” of the human body surface was developed over four decades ago (25), and has more recently been applied to the measurement of whole-body surface anthropometry, whole-body and regional volumes, and body fat (26). 3-DPS is a noninvasive, optical method that uses high-speed digital cameras and mathematics to detect the exact position of laser-light points (664 nm, eye-safe class-1) projected onto the surface of the body and reflected to the cameras. Software uses these points to generate a 3-D body image as well as to compute values for total and regional body volumes and dimensions, such as body circumferences, lengths, widths, and thicknesses (27).
The measurement accuracy and precision of the scanner are directly related to the number of data points obtained from the surface. The greater the number of data points, the higher the resolution or precision. The software can also calculate volume for any selected region of the body. 3-DPS has potential application in the prevention, classification, and monitoring of disease treatments that relate to body shape, size, or degree of fatness, and in growth and development, aging, weight management, and fitness management. The 3-DPS has important applications in the measurement of extreme obesity and in studies of patients before and after bariatric surgery. Validation studies for total-body volume from the 3-DPS have been conducted in children and adults using underwater weighing and air-displacement plethysmography techniques as standards (Table 13.2, Figure 13-5) (27–29).
Table 13-2 Application of 3-DPS for Volume and Dimension Measurements Compared to Established Methods, with Skill, Cost, and Risk Comparison
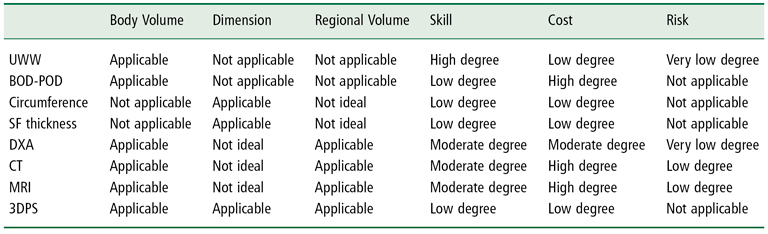
UWW, underwater weighing; SF thickness, skinfold thickness; DXA, dual energy x-ray absorptiometry; CT, computed tomography; MRI, magnetic resonance imaging; 3DPS, three-dimensional photonic scan.
Figure 13-5 A 3-dimensional photonic scan image in A) adult male weighing 182-kg; and B) a child
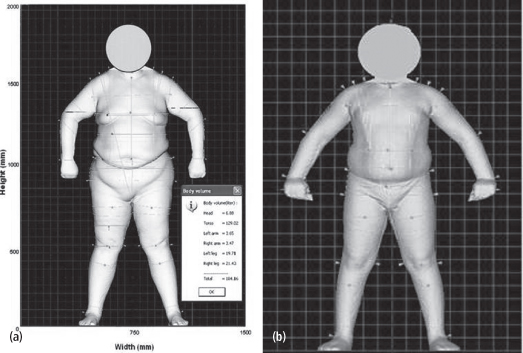
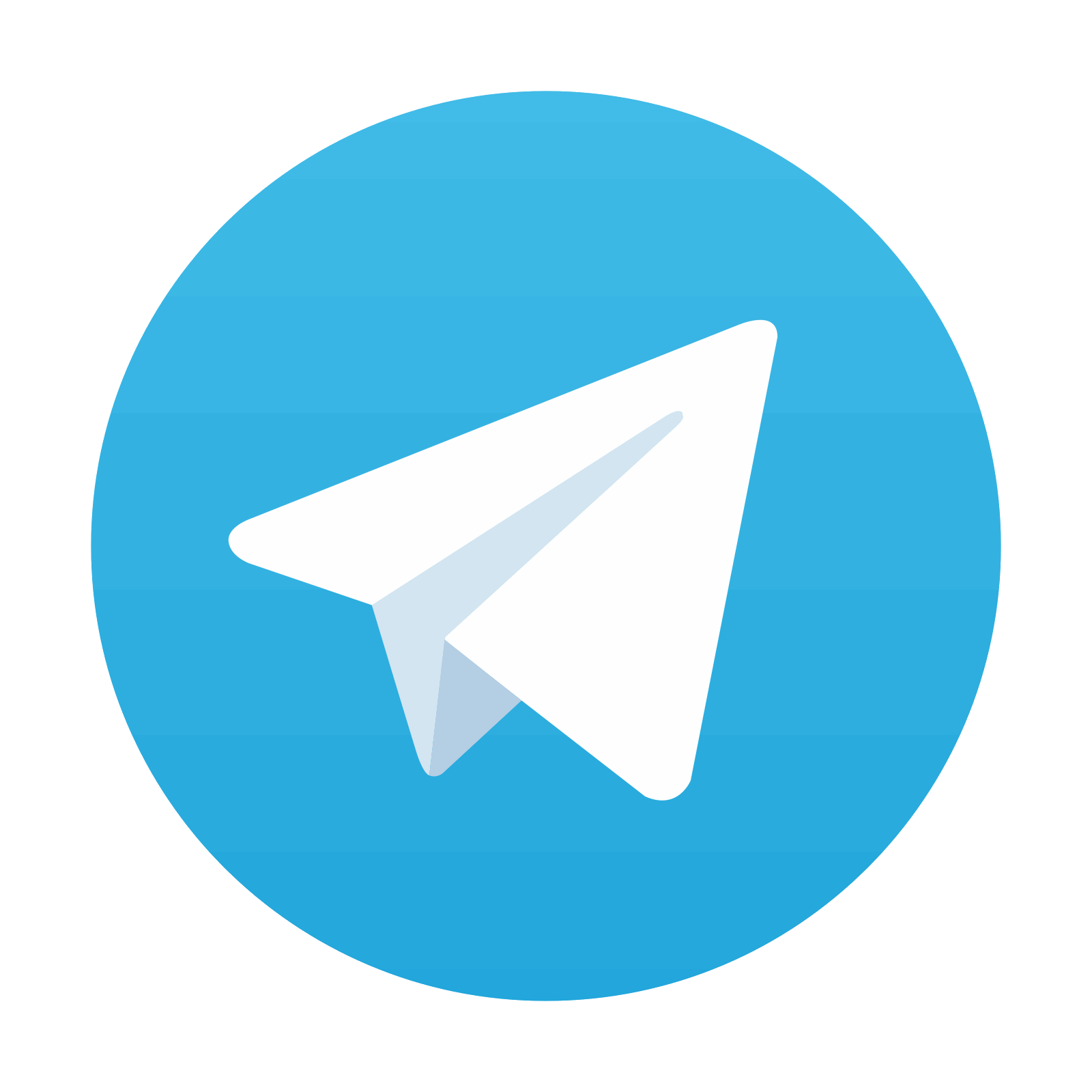
Stay updated, free articles. Join our Telegram channel

Full access? Get Clinical Tree
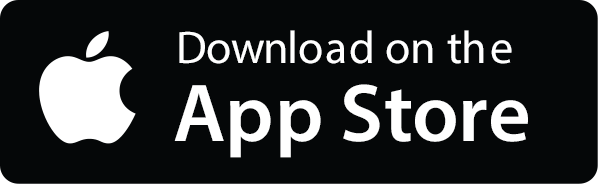
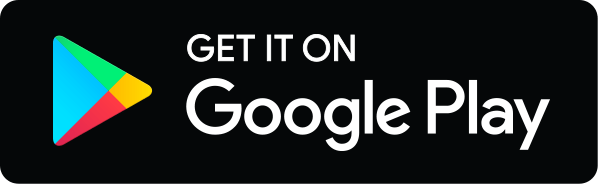