Neurologic
• Depressed consciousness/inability to protect airway
• Need for sedatives to control intracranial pressure
• Status epilepticus
Pulmonary
• Acute respiratory distress syndrome
– Sepsis
– Aspiration
– Transfusion-related acute lung injury
– Pneumonia
– Fat emboli
• Pulmonary edema
– Neurogenic
– Cardiac contusion
– Fluid overload
• Trauma-related
– Pulmonary contusion
– Pneumothorax
– Hemothorax
– Flail chest/rib fractures
• Venous thromboembolism
Airway
• Head/neck trauma
Other
• Need for procedures/operative intervention
• Need for pain control
• Increased metabolic demands from acidosis/neurohormonal response to injury
One condition that deserves specific mention is neurogenic pulmonary edema (NPE), which is reported to complicate approximately 20 % of cases of TBI [10]. The underlying pathogenesis of NPE is poorly understood, but it is believed that increases in intracranial pressure (ICP) lead to the activation of sympathetic nervous system and release of catecholamines with resultant changes in cardiopulmonary hemodynamics and starling forces leading to rapid onset of pulmonary edema [18]. The onset of NPE may be early, minutes to hours after the neurologic insult, or delayed, occurring 12–24 h following injury [18].
In addition to direct insults to the lung, traumatic injury increases cellular metabolism leading to increased oxygen utilization and carbon dioxide production [19]. Furthermore, metabolic acidosis from shock results in increased work of breathing for carbon dioxide clearance [19]. These increases in metabolic demands, particularly when combined with primary or secondary lung injury, may result in hypercapnic respiratory failure.
Intracranial Physiology and the Effects of Mechanical Ventilation
An understanding of basic intracranial physiology and how it is affected by MV are essential in caring for the severe TBI patient. As mentioned in the introduction to this chapter, avoidance of secondary injury is the cornerstone of TBI management. Secondary injury may result from hypoxemia or inadequate cerebral blood flow (CBF). CBF is determined by the cerebral perfusion pressure (CPP) divided by the cerebrovascular resistance (CVR). As monitoring of CBF is impractical clinically, CPP is typically used as a surrogate marker for adequacy of CBF. CPP is the difference between mean arterial pressure (MAP) and ICP. Given this relationship, inadequate CPP may result from decreased MAP or increased ICP. Hypotension, elevated ICP, and low CPP are associated with worsened clinical outcomes in TBI [20].
Mechanical ventilation (MV) influences cerebral oxygen delivery in a number of ways. The fraction of inspired oxygen (FiO2) directly affects arterial oxygen tension and in turn, cerebral oxygen delivery. Severe arterial hypoxemia also causes cerebral vasodilation resulting in an increase in ICP [21]. Multiple studies have demonstrated an association between hypoxemia and adverse outcomes in TBI [22–24]. Minute ventilation alters PCO2, which acts as a potent modulator of CBF. Increases in PCO2 result in vasodilation, and in turn, increased cerebral blood volume. In an injured brain with decreased intracranial compliance, this can increase ICP and reduce cerebral perfusion. Decreases in PCO2 result in vasoconstriction and decreased cerebral blood volume. While hyperventilation is sometimes utilized as a short-term management strategy to reduce ICP in a patient with uncontrolled intracranial hypertension and impending brainstem herniation, routine hyperventilation should be avoided as the resultant vasoconstriction may lead to inadequate cerebral blood flow and secondary injury [25]. Studies of prehospital ventilation have found that both hypo- and hypercapnia are associated with increased in-hospital mortality [5, 26].
MV also impacts CPP via positive end expiratory pressure (PEEP). PEEP improves oxygenation and ventilation by recruiting collapsed lung units; however, the increase in intrathoracic pressure may potentially be associated with deleterious effects on the injured brain. PEEP increases intrathoracic pressure, causing decreased thoracic venous return, which: (1) increases cerebral blood venous volume and thus ICP and (2) reduces cardiac preload and cardiac output, and thus reduced CPP [27]. Additionally, when applied incorrectly alveolar overdistension from PEEP can result in hypercapnia, resulting in cerebral vasodilation and raised ICP [28]. While theoretical concerns exist regarding the application of PEEP in the brain-injured patient, the bulk of available studies suggest that the effects of PEEP on ICP are relatively modest [29–34]. Figure 23.1 summarizes the potential adverse effects of MV on the injured brain.


Fig. 23.1
Effects of mechanical ventilation on intracranial physiology
Mechanical Ventilation Strategies
Selection of appropriate MV settings in polytrauma TBI patients requires a comprehensive assessment of the patient’s injuries. Selection of the mode of MV should take into consideration the severity of hypoxemic respiratory failure and the presence of intracranial hypertension . The mode of MV selected should correct hypoxemia and hypercapnia, while at the same time limiting plateau pressure and tidal volume to a level that does not potentiate ventilator-induced lung injury. For the majority of TBI patients, we recommend use of volume-assisted control ventilation, as it is the MV mode utilized in the bulk of the ARDS medical literature. It should be recognized that volume-assisted control has not been conclusively demonstrated to be superior to other modes of MV in randomized, controlled trials. Patients who cannot be adequately oxygenated or ventilated with volume assist control may require additional rescue therapies. In this section, we will discuss the rationale for selection of tidal volume; PEEP and FiO2 in patients on volume assist control ventilation. We will then discuss rescue therapies including use of paralytics, prone positioning, nitric oxide, and extracorporeal support.
Selection of Optimal Rate and Tidal Volume
In 2000, the Acute Respiratory Distress System Network (ARDSNet) published a landmark trial comparing outcomes in patients with ARDS ventilated with a low volume strategy of 6 mL/kg of ideal body weight (IBW) versus 12 mL/kg IBW. The trial was terminated early as mortality was significantly lower in the 6 mL/kg IBW group (31 versus 39.8 %, p = 0.007) [35]. The methods of this trial represent the only ventilatory strategy known to reduce mortality in ARDS. Given this, considerable attention has been paid to the limitation of tidal volumes in ventilated patients and some speculate that a “lung protective ” strategy may even be beneficial in a non-ARDS population [36]. In fact, high tidal volume ventilation has been associated with subsequent development of ARDS [6, 37].
A low tidal volume “lung protective strategy” is not without risks in the brain-injured population; however, as it has the potential to cause hypercapnia and raise ICP. The low tidal volume also requires heavy sedation in some patients, which limits the utility of the neurological assessment, making an ICP monitor necessary. In light of these concerns and the unclear benefit of tidal volumes of 6 mL/kg IBW when plateau pressure is <30 cm H2O, a balanced strategy targeting a tidal volume of 6–8 mL/kg IBW and a plateau pressure <30 may be more suitable in the TBI patient [38, 39]. Arterial blood gases should be closely monitored and changes made to target a PCO2 of 35–40 mm Hg.
The injured brain, particularly with brainstem lesions, may induce periods of rapid or irregular breathing, resulting in ventilator dyssynchrony, contributing to overdistension and effecting gas exchange due to autopeep [40]. In such cases, sedation and possible paralysis will be necessary. As a result of the loss of neurologic exam, ICP monitoring may be necessary in these patients.
In cases of increased extrapleural pressure, such as from a hemothorax, pleural effusion, or abdominal compartment syndrome, plateau pressures >30 mm Hg may not cause ventilator-induced lung injury since transpulmonary pressure is not increased [41]. However, the effects of such pressures may decrease CPP and increase ICP. Surgical interventions such as decompressive laparotomy may be necessary to lower ICP [42].
Optimization of Oxygenation—Positive End Expiratory Pressure (PEEP) and Fraction of Inspired Oxygen (FiO2)
Positive End Expiratory Pressure (PEEP)
PEEP therapy is necessary to prevent lung injury due to repetitive opening and collapse of alveoli, or atelectrauma. PEEP may improve outcomes, but only in patients with moderate or severe ARDS by the current definition, suggesting a preferential effect based on lung compliance [7, 43]. As mentioned earlier, increases in PEEP have the potential to reduce CBF and raise ICP, although the effects demonstrated in the limited available studies are variable and mild. In a small clinical series of TBI patients with ICP < 20 mm Hg, an increase in PEEP from 0–15 cm H2O did not increase ICP [34]. Yet another study suggested that effect on ICP is based on alveolar recruitment. Mascia, et al. studied the effects of increased PEEP in 12 severely brain-injured patients. They found that when PEEP resulted in alveolar recruitment, ICP was unchanged; however, when increased PEEP failed to result in alveolar recruitment, ICP was increased. They hypothesized this was due to alveolar hyperinflation and resultant hypercapnia [28]. A similar study found that increasing PEEP from 0 to 12 mm Hg in mixed neurocritical care patients resulted in decreased CPP and MAP in patients with normal lung compliance, but had no effect on these parameters in patients with poor lung compliance [44].
In a small study of nine TBI patients with polytrauma with hypoxia and elevated ICP, incremental increases in PEEP from 0 to 21 mm Hg improved oxygenation (PaO2/FiO2 increased from 128 to 245), while ICP only marginally increased (27–29 mm Hg) and CPP remained stable [45]. A study in mixed neurocritical care patients with raised ICP (18 mm Hg), PEEP up to 15 mm Hg did not significantly alter ICP or CPP [33].
High levels of PEEP for short periods of time, or recruitment maneuvers, as a salvage therapy for refractory hypoxemia have been shown to improve oxygenation [46]. However, in one study, the tradeoff for improved oxygenation in TBI patients was a reduction in jugular venous saturation, concerning for cerebral ischemia, despite an improvement in PaO2 [47].
Given the paucity and heterogeneity of studies, firm conclusions regarding the use of PEEP in TBI are difficult. Modest amounts of PEEP (5–10 mm Hg) are unlikely to be associated with detrimental intracranial effects. High PEEP (>10 mm Hg) should be avoided with mild ARDS and patients exhibiting minimal improvement of PaO2/FiO2 with increasing PEEP, but can be cautiously applied in patients with severe hypoxemic respiratory failure . As PEEP is increased, ICP and CPP should be closely monitored. Decreases in MAP secondary to PEEP should be addressed with fluid loading or vasopressors to prevent resultant decreases in CPP. Few studies have assessed the effect of PEEP >15 mm Hg; so extreme caution should be exercised in applying this strategy.
Fraction of Inspired Oxygen (FiO2)
As mentioned previously, hypoxemia is associated with worsened outcomes in the brain-injured patient [22–24]. At a minimum, FiO2 should be set to avoid hypoxia (PaO2 < 60 mm Hg). Hyperoxia may also be detrimental, though a clear threshold at which negative effects ensue has yet to be defined [48–50]. Based on the available data, it is reasonable to target a PaO2 of 80–100 mm Hg in the TBI population to provide some “buffer” against transient insults that may cause hypoxemia while avoiding the possible detrimental effects of hyperoxia as well.
Rescue Modalities for Severe Hypoxemic Respiratory Failure
Salvage treatments can be considered in hypoxemic patients not responding to or not tolerating higher PEEP . Such treatments include airway pressure release ventilation (APRV), prone positioning, paralysis, nitric oxide, and extracorporeal membrane oxygenation.
Airway Pressure Release Ventilation (APRV)
APRV is a pressure-limited, time-cycled mode of ventilation that allows a patient to breathe spontaneously during the application of continuous positive airway pressure [51]. The mode maintains a high pressure for the majority of the respiratory cycle with periodic releases to a low pressure. It allows a very high mean airway pressure to be achieved without high peak pressures which may result in barotrauma. A single case report by Marik and colleagues describes the successful use of this mode in the setting of increased ICP. No significant detrimental effects on ICP or CPP were observed and oxygenation improved significantly [51]. Clarke, et al. reported a similar experience with inverse ratio pressure control ventilation in nine patients with head injury [52]. If inverse ratio ventilation or APRV is utilized in the setting of TBI, it should be done so in the setting of hypoxemic respiratory failure refractory to conventional ventilator settings by a practitioner experienced with the modality. The effects on PCO2, CPP and ICP should be monitored closely.
Prone Positioning
Prone positioning recruits collapsed lung regions and has lowered mortality in severe ARDS(PaO2/FiO2 < 100) particularly when added to tidal volumes <8 mL/kg IBW [53]. However, proning TBI patients with elevated ICP can raise intrathoracic and intraabdominal pressures, increasing ICP. In an observational study of 29 neurocritical care patients with ICP monitors, prone positioning improved oxygenation at the expense of higher mean ICP and more frequent ICP elevation >20 mm Hg [54]. As with high PEEP, prone positioning has to be undertaken with caution.
Nitric Oxide
In multiple studies, inhaled nitric oxide has been shown to improve oxygenation in ARDS, but without improving outcomes or mortality [55]. Several case reports have described the use of inhaled nitric oxide in the setting of ARDS associated with TBI [56–59]. Some have postulated that nitric oxide may have beneficial effects on both the lung and injured brain in the setting of ARDS in TBI [60]. Further study is required to determine if inhaled nitric oxide truly has a role in the management of TBI complicated by ARDS.
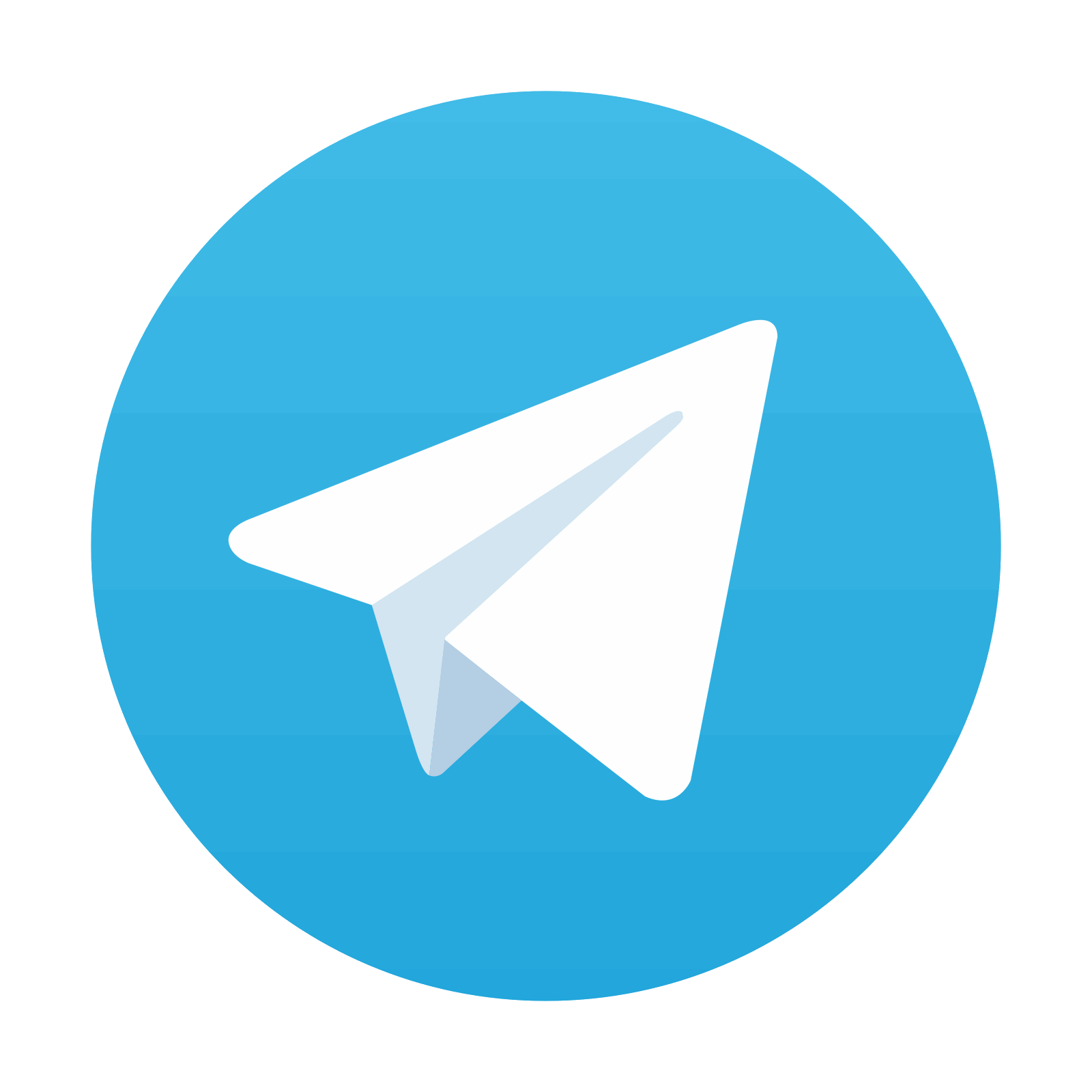
Stay updated, free articles. Join our Telegram channel

Full access? Get Clinical Tree
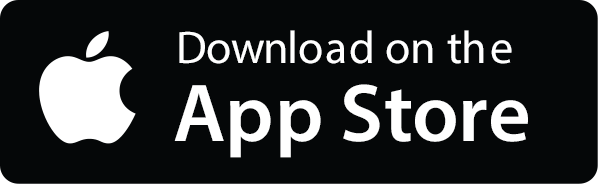
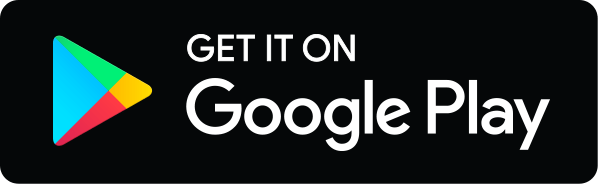