The prevention and management of medical complications are important for improving outcomes after subarachnoid hemorrhage (SAH). Fever, anemia requiring transfusion, hyperglycemia, hyponatremia, pneumonia, hypertension, and neurogenic cardiopulmonary dysfunction occur frequently after SAH. There is increasing evidence that acute hypoxia and extremes of blood pressure can exacerbate brain injury during the acute phase of bleeding. There are promising strategies to minimize these complications. Randomized controlled trials are needed to evaluate the risks and benefits of these and other medical management strategies after SAH.
Scope of the problem
Aneurysmal subarachnoid hemorrhage (SAH) is a devastating disease with high disability and mortality rates. Poor clinical grade on admission, age, large aneurysm size (>10 mm), and aneurysm rebleeding have the strongest impact on outcome after SAH. Delayed cerebral ischemia (DCI) from vasospasm, which affects 20% to 45% of patients, is also associated with poor neurologic outcome and mortality and has traditionally been the primary focus of postoperative management.
In addition to the direct effects of the initial hemorrhage and secondary neurologic complications, SAH predisposes to medical complications that can have an impact on outcome and increase hospital length of stay. In the placebo arm of the Cooperative Aneurysm Study investigating the effects of nicardipine, the five most frequent non-neurologic complications were anemia, hypertension, cardiac arrhythmia, fever, and electrolyte abnormalities. The proportion of deaths directly attributable to medical complications (23%) was comparable to that of vasospasm (23%) and rebleeding (22%). Advances in aneurysm treatment and neurologic intensive care, with increasing emphasis on aggressive treatment of poor-grade patients, have in all likelihood increased the relative importance of medical complications after SAH.
Physiologic derangements after subarachnoid hemorrhage
Abnormalities of oxygenation, glucose metabolism, and hemodynamic instability within 24 hours of onset can potentially exacerbate the initial brain injury caused by SAH. Claassen and colleagues created a SAH–Physiologic Derangement Score (SAH-PDS), range 0–8, from the most abnormal measurements of physiologic variables (listed in Table 1 ) within 24 hours of admission after SAH. The SAH-PDS was independently associated with death or moderate-to-severe disability ( Fig. 1 ) and was found superior to the Acute Physiology and Chronic Health Evaluation-2 (APACHE-2) score and the systemic inflammatory response syndrome (SIRS) score for quantifying the immediate impact of physiologic derangements on outcome after SAH. Interventions to correct these abnormalities, such as tight blood pressure control, brain tissue oxygen tension–directed therapy, or continuous insulin infusion, are reasonable therapeutic options given the current state of knowledge and are promising targets for future safety and feasibility trials.
Physiologic Derangement | Pathophysiology | Points |
---|---|---|
Arterioalveolar gradient >125 mm Hg | Oxygen deficits from neurogenic pulmonary edema, aspiration pneumonia, or neurogenic stunned myocardium with pump failure | 3 |
Serum bicarbonate <20 mm Hg | Lactic acidosis due to acute severe peripheral vasoconstriction and skeletal muscle glycolsysis | 2 |
Serum glucose >180 mg/dL | Elevated blood glucose exacerbates ischemic brain injury, increases the risk of infection and critical illness myopathy, or may be a marker of severe brain injury | 2 |
Mean arterial pressure of <70 or >130 mm Hg | Hypotension may be related to neurogenic stunned myocardium or vasodilatory shock triggered by brainstem compression, and can aggravate ischemic injury when autoregulation is impaired. Hypertension reflects the initial severity of brain injury and may provoke autoregulatory breakthrough and aggravate intracranial hypertension | 1 |
Maximum score | 8 |
Physiologic derangements after subarachnoid hemorrhage
Abnormalities of oxygenation, glucose metabolism, and hemodynamic instability within 24 hours of onset can potentially exacerbate the initial brain injury caused by SAH. Claassen and colleagues created a SAH–Physiologic Derangement Score (SAH-PDS), range 0–8, from the most abnormal measurements of physiologic variables (listed in Table 1 ) within 24 hours of admission after SAH. The SAH-PDS was independently associated with death or moderate-to-severe disability ( Fig. 1 ) and was found superior to the Acute Physiology and Chronic Health Evaluation-2 (APACHE-2) score and the systemic inflammatory response syndrome (SIRS) score for quantifying the immediate impact of physiologic derangements on outcome after SAH. Interventions to correct these abnormalities, such as tight blood pressure control, brain tissue oxygen tension–directed therapy, or continuous insulin infusion, are reasonable therapeutic options given the current state of knowledge and are promising targets for future safety and feasibility trials.
Physiologic Derangement | Pathophysiology | Points |
---|---|---|
Arterioalveolar gradient >125 mm Hg | Oxygen deficits from neurogenic pulmonary edema, aspiration pneumonia, or neurogenic stunned myocardium with pump failure | 3 |
Serum bicarbonate <20 mm Hg | Lactic acidosis due to acute severe peripheral vasoconstriction and skeletal muscle glycolsysis | 2 |
Serum glucose >180 mg/dL | Elevated blood glucose exacerbates ischemic brain injury, increases the risk of infection and critical illness myopathy, or may be a marker of severe brain injury | 2 |
Mean arterial pressure of <70 or >130 mm Hg | Hypotension may be related to neurogenic stunned myocardium or vasodilatory shock triggered by brainstem compression, and can aggravate ischemic injury when autoregulation is impaired. Hypertension reflects the initial severity of brain injury and may provoke autoregulatory breakthrough and aggravate intracranial hypertension | 1 |
Maximum score | 8 |
Fever
Fever (≥38.3°C) is a frequent event in patients with SAH (41%–54% [ Fig. 2 ]) and in neurocritical care patients in general. In patients with acute brain injury, fever leads to worsening of cerebral edema and intracranial pressure (ICP), exacerbation of ischemic injury, increased oxygen consumption, and depressed level of consciousness. Fever after SAH is associated with an increased risk of symptomatic vasospasm, an increased length of intensive care unit (ICU) and hospital stay, and death and poor functional outcome at 3 months ( Fig. 3 ). Fever after SAH has been shown to have adverse effects on the outcome of good- and poor-grade patients. Infection (pneumonia, urinary tract infection, catheter-related bacteremia, upper respiratory tract infection, or meningitis) can be identified in approximately 34% to 75% of febrile SAH patients, meaning that in the remainder, the cause of fever may be central or neurogenic in etiology. In the Columbia University SAH Outcomes Project, only pneumonia was significantly associated with fever burden. In a cohort of SAH patients, fever was classified as noninfectious in 48% and infectious in only 18% based on rigorous review of cultures and diagnostic studies. Noninfectious fever tended to present earlier in the hospital course (<72 hours after NICU admission). It has been hypothesized that altered rhythmic daily temperature variability may help differentiate central from infectious in SAH patients.
Risk factors for fever burden in the Columbia University SAH Outcomes Project patient group included poor Hunt-Hess scale grade at admission, loss of consciousness at ictus, thick cisternal clot, intraventricular hemorrhage, and aneurysm size greater than or equal to 10 mm. Fever is also a common component of the SIRS, which has been shown to predict poor outcome in SAH patients. Brain stem herniation, hydrocephalus treated with external ventricular drainage, cerebral infarction, respiratory failure, anemia requiring transfusion, and hyperglycemia are neurologic and medical complications with a significant association with fever burden. In one study, coiling as method of aneurysm repair was related to a higher fever burden than clipping or no repair, for reasons that are unclear.
Fever control can now be achieved by means of core temperature–controlled surface or endovascular cooling devices. Feasibility studies have demonstrated safe and effective fever control in febrile SAH patients refractory to acetaminophen treatment using the Celsius Control System (Innercool, San Diego, California), and a single-center randomized trial showed a 75% reduction in fever burden with the systemic surface cooling system Artic Sun temperature management system (Medivance, Louisville, Colorado) compared with regular water-circulating cooling blankets. This confirms the results of a previous multicenter fever control trial comparing a catheter-based heat exchange system, the CoolGard/CoolLine system (Alsius, Irvine, California) plus standard fever management (using acetaminophen, ibuprofen, and cooling blankets) to standard therapy alone, in which a 64% reduction in fever burden was shown. Induction of normothermia with ice packs and external surface cooling devices in poor-grade SAH patients significantly reduced episodes of metabolic crisis in the brain assessed by microdialysis markers of metabolic stress (lactate/pyruvate ratio) and ICP.
With all forms of cooling, the main barrier to achieving target temperature is insufficient control of shivering, which increases the systemic rate of metabolism and resting energy expenditure and may potentially adversely affect cerebral oxygenation and ICP. Shivering is a natural mechanism that allows the body to create heat if the body temperature falls below the hypothalamic set point. Potentially effective antishivering interventions are listed in Table 2 . Treatment guidelines almost universally advocate maintenance normothermia for all febrile patients with SAH, despite little evidence to support this practice in the form of randomized controlled trials. Prospective clinical trials are needed to assess the impact of fever control with systemic surface or intravascular cooling devices on development of vasospasm and outcome after SAH.
Method | Dosage | Mechanism |
---|---|---|
Basic management | ||
Acetaminophen | 650–1000 mg orally every 6 h | Inhibits prostaglandin synthesis |
Buspirone | 30–60 mg orally every 8 h | 5HT-1A partial agonist |
Skin counterwarming | Bair Hugger polar air cooling system (Arizant Healthcare, Eden Prairie, Minnesota) | Vasodilatation |
Advanced management for persistent shivering | ||
Magnesium sulfate | 0.5–1.0 g/h for target serum magnesium of 1–2 mmol/L (3–4 g/dL) | Vasodilatation, muscle relaxant |
Clonidine | 15–60 μg/h (1.5–3 μg/kg/h) | α2-Receptor agonist |
Dexmedetomidine | 0.2–1.5 mcg/kg/h | α2-Receptor agonist |
Meperidine (Pethidine) | 25–100 mg IV every 4 h (or 0.5–1.0 mg/kg/h) | Opioid receptor agonist |
Fentanyl | 50–200 μg/h | Opioid receptor agonist |
Advanced management for refractory shivering | ||
Propofol | 75–300 mg/h | Impairs vasoconstriction and shivering threshold |
Rocuronium/Vecuronium | Usually not needed and should be avoided because of increased incidence of critical illness polyneuropathy | Paralysis |
Anemia
Anemia after SAH most likely results from the combined effects of an SAH-related reduction in red blood cell mass, combined with blood losses due to phlebotomy and invasive procedures and hemodilution from fluid administration. In the Columbia University SAH Outcomes Project, anemia (defined as hemoglobin <9 g/dL requiring blood transfusion) occurred in 36% of 580 patients and was the second most common medical complication (see Fig. 2 ). Anemia treated with blood transfusion is associated with an increased risk of mortality and poor functional outcome after SAH (see Fig. 3 ). Administration of blood during the hospital course after aneurysm surgery has also been associated with an increased risk of asymptomatic and symptomatic angiographically-confirmed vasospasm. In another study, blood transfusions were significantly related to poor outcome among SAH patients with vasospasm. Multimodality monitoring in SAH patients demonstrated local brain tissue hypoxia (partial pressure of brain tissue oxygen [PbtO 2 ] <20 mm Hg) and cell energy dysfunction (lactate/pyruvate ratio >40) when hemoglobin values were less than 9 g/dL; however, the relationship to functional outcome was not investigated.
It is unclear whether anemia after SAH reflects general illness severity or whether the treatment for anemia—blood transfusion—directly contributes to poor outcome. History of blood transfusion was an independent risk factor for intracerebral hemorrhage and mortality after SAH in the Japan Collaborative Cohort Study and Miyako Study. Higher hemoglobin values have been associated with a lower risk of cerebral infarction and poor outcome 3 months after SAH in two different SAH cohorts. Blood transfusions were related to poor functional outcome but not to mortality in one of these studies and were not considered in the other. Kramer and colleagues investigated the effect of anemia as opposed to blood transfusion on secondary complications and outcome after SAH. Blood transfusion, but not anemia, was an independent risk factor for poor functional outcome and was associated with the development of nosocomial infections but not symptomatic vasospasm. The extent of hemoglobin decline during the first 2 weeks after SAH predicted unfavorable outcome (severe disability or death) and was more pronounced in patients with poor-grade SAH, thick cisternal clot, and intraventricular hemorrhage.
In the Columbia University SAH Outcomes Project, blood transfusions were related to symptomatic vasospasm and were a significant predictor of mortality and poor functional outcome at 3 months; anemia alone did not influence long-term functional outcome in this model. Packed red blood cells (PRBCs) may be depleted of nitric oxide, an endogenous vasodilator that can reverse vasoconstriction of cerebral arteries and arterioles during vasospasm. Thus, transfusion may result in dilution of this active vasodilatory substance and may subsequently worsen microcirculatory flow or predispose to intraoperative cerebral vasoconstriction. Transfusion of PRBCs increases local PbtO 2 in the majority of patients with SAH and other severe brain injuries independent of cerebral perfusion pressure and peripheral oxygen saturation. Stored PRBCs, however, have proinflammatory effects and may induce immunodysfunction and neutrophilic and polymorphonuclear cytotoxicity, which may exacerbate the inflammatory component of vasospasm and increase the risk of nosocomial infections. The deformability of stored and transfused erythrocytes is reduced, which may lead to microvascular sludging, and adenosine triphosphate and 2,3 diphosphoglycerate are depleted, resulting in altered oxygen binding and release. Transfused erythrocytes also contain free iron, which can increase oxidative processes in its ferrous form and aggravate ischemia. Storage of PRBCs has been found to generate interleukin 1,-6, and -8 and tumor necrosis factor α, which may augment ischemia and edema formation.
Given the potential detrimental effects of PRBC transfusion, efforts directed at more physiologic transfusion triggers derived from brain multimodality monitoring ( Table 3 ) and prevention of anemia after SAH with erythropoietin should be investigated, particularly given its potential neuroprotective properties.
Hemoglobin (g/dL) | Packed Red Blood Cell Transfusion |
---|---|
>10 | No |
<7 | Yes |
7–10 | Yes, if PbtO 2 <20 mm Hg or rS o 2 <60% or cardiopulmonary reserve decreased |
Hyperglycemia
Hyperglycemia is known to have an adverse effect on outcome in patients with acute ischemic stroke and to increase the likelihood of intracranial hemorrhage after thrombolytic therapy. In the authors’ SAH population, hyperglycemia exceeding 11.1 mmol/L (200 mg/dL) occurred in 30% (see Fig. 2 ) and was a significant predictor of poor functional outcome and mortality 3 months after SAH (see Fig. 3 ). Depending on the definition, hyperglycemia can be found in 30% to 100% of SAH patients. When mean daily glucose burden between day 0 and 10 after SAH (defined as the area under the curve above 5.8 mmol/L or 105 mg/dL) was analyzed, hyperglycemia was found to have a stronger association with moderate-to-severe disability (modified Rankin scale [mRS] 4–6) and loss of high-level functional independence than with mortality, suggesting that hyperglycemia may contribute to physical deconditioning. The relationship of admission or sustained hyperglycemia with poor outcome up to 1 year after SAH has also been confirmed in many other studies. In the Intraoperative Hypothermia for Aneurysm Surgery Trial population, glucose concentration at the time of aneurysm clipping did not have an impact on mortality at 3 months but influenced physical impairment measured with the National Institute of Health Stroke Scale, neuropsychologic outcome, and intensive care unit (ICU) length-of-stay. In a smaller study of poor-grade SAH patients, early clinical improvement was seen in patients with an admission glucose level less than 180 mg/dL (10.0 mmol/L). Elevated blood glucose concentrations on arrival at the hospital were associated with aneurysm rebleeding in another SAH patient cohort. Increased glucose levels, however, have not always been found to be significant predictors of poor functional status after SAH.
A retrospective study of 352 SAH patients at Massachusetts General Hospital identified hyperglycemia (mean inpatient blood glucose value ≥140 mg/dL) in 73% of patients and found an association with symptomatic vasospasm and increased ICU length of stay. The risk of symptomatic vasospasm was also increased with hyperglycemia in a cohort of 244 SAH patients. These findings are in contrast to an analysis of 175 patients in which elevated admission glucose was not predictive of DCI, despite an association with poor outcome at 3 months.
Acute brain injury may lead to a transient generalized stress response, which may explain the high frequency of hyperglycemia after SAH in patients who do not have a history of diabetes mellitus. Hyperglycemia was significantly linked to a history of diabetes mellitus, older age, poor clinical grade, brainstem compression from herniation, higher APACHE-2 physiologic derangement scores, and pulmonary decompensation (congestive heart failure, respiratory failure, and pneumonia) in the authors’ study. Hyperglycemia is thus just one aspect of a generalized stress response after SAH which can be triggered by a variety of different perturbations. Activation of the sympathetic nervous system increases glucagon, corticosteroids, and somatotropin secretion and decreases insulin release, all of which cause stress-related hyperglycemia. When this acute metabolic response is persistent, hyperglycemia predicts the occurrence of symptomatic vasospasm, DCI, and poor long-term functional outcome.
The proportion of SAH patients with known diabetes mellitus is relatively low (<10%). Although hyperglycemia after SAH could simply reflect pre-existing impaired glucose tolerance, previous multivariate analyses have shown that the relationship between hyperglycemia and poor outcome is independent of other known predictors. In a study utilizing monitoring of cerebral metabolism with microdialysis, SAH patients with an acute focal neurologic deficit from the initial hemorrhage or procedural complications had systemic hyperglycemia associated with low cerebral glucose levels and elevated lactate/pyruvate ratios (indicating cerebral metabolic crisis) and worse functional outcome at 6 months. In another microdialysis study, episodes of hyperglycemia were frequent in patients with an acute neurologic deficit or DCI and were accompanied by elevated cerebral glycerol levels (a marker of cellular membrane degradation). Blood glucose elevations have also been related to increased lactate/pyruvate ratios, which argues for increased anaerobic glycolysis with saturation of normal aerobic glucose metabolism. This process may exacerbate tissue injury from ischemia.
Strict glucose control has been associated with reduced ICP, duration of mechanical ventilation, hospital length of stay, use of vasopressors, frequency of seizures, and diabetes insipidus in critically ill neurologic patients. Intensive insulin therapy has also been shown to reduce mortality in critically ill surgical ICU patients and in medical ICU patients admitted for more than 2 days. The first randomized trial of management of poststroke hyperglycemia with 24-hour glucose-potassium-insulin infusions failed to demonstrate a benefit on mortality or disability 90 days after stroke. Glycemic control in this study, however, was poor and the duration of treatment to short. A small trial of 55 patients with SAH demonstrated the feasibility and safety of continuous insulin infusion for glucose values exceeding 7 mmol/L with glucose assessments performed every 2 hours. Retrospective analyses of changes in clinical practice through introduction of insulin protocols in SAH patients showed that achievement of tight glycemic control significantly reduced the likelihood of poor outcome at 6 months and have identified hypoglycemia (<60 mg/dL) as an independent predictor of mortality at discharge.
Balanced against the potential benefits of tight glycemic control is evidence that normalization of hyperglycemia can lead to critical brain tissue hypoglycemia after severe grain injury. Intravenous (IV) insulin therapy for a target glucose of 140 mg/dL (7.8 mmol/L) resulted in critical decreases of cerebral glucose measured with microdialysis at 3 hours after initiation of treatment in 79% of SAH patients, predominantly in men and in the elderly. At 8 hours after start of insulin infusion, cerebral glycerol increased reflecting tissue damage or cellular distress. Another microdialysis study of patients with SAH and other form of brain injury demonstrated a link between high insulin dosages, brain tissue hypoglycemia, elevated lactate/pyruvate ratios, and increased mortality at discharge. Reductions of cerebral glucose has been found related to peri-ischemic cortical depolarizations.
Bilotta and colleagues conducted the first randomized trial of intensive insulin therapy (target glucose 80–120 mg/dL) versus standard insulin therapy (target glucose 80–220 mg/dL) in 78 SAH patients. Rate of infection was the primary outcome measure and was significantly reduced from 42% to 27% in the intensive insulin group. Mortality at 6 months and the frequency of vasospasm were comparable in the two groups. More safety trials of intensive insulin therapy in SAH with cerebral glucose monitoring and efficacy studies exploring long-term outcomes are needed.
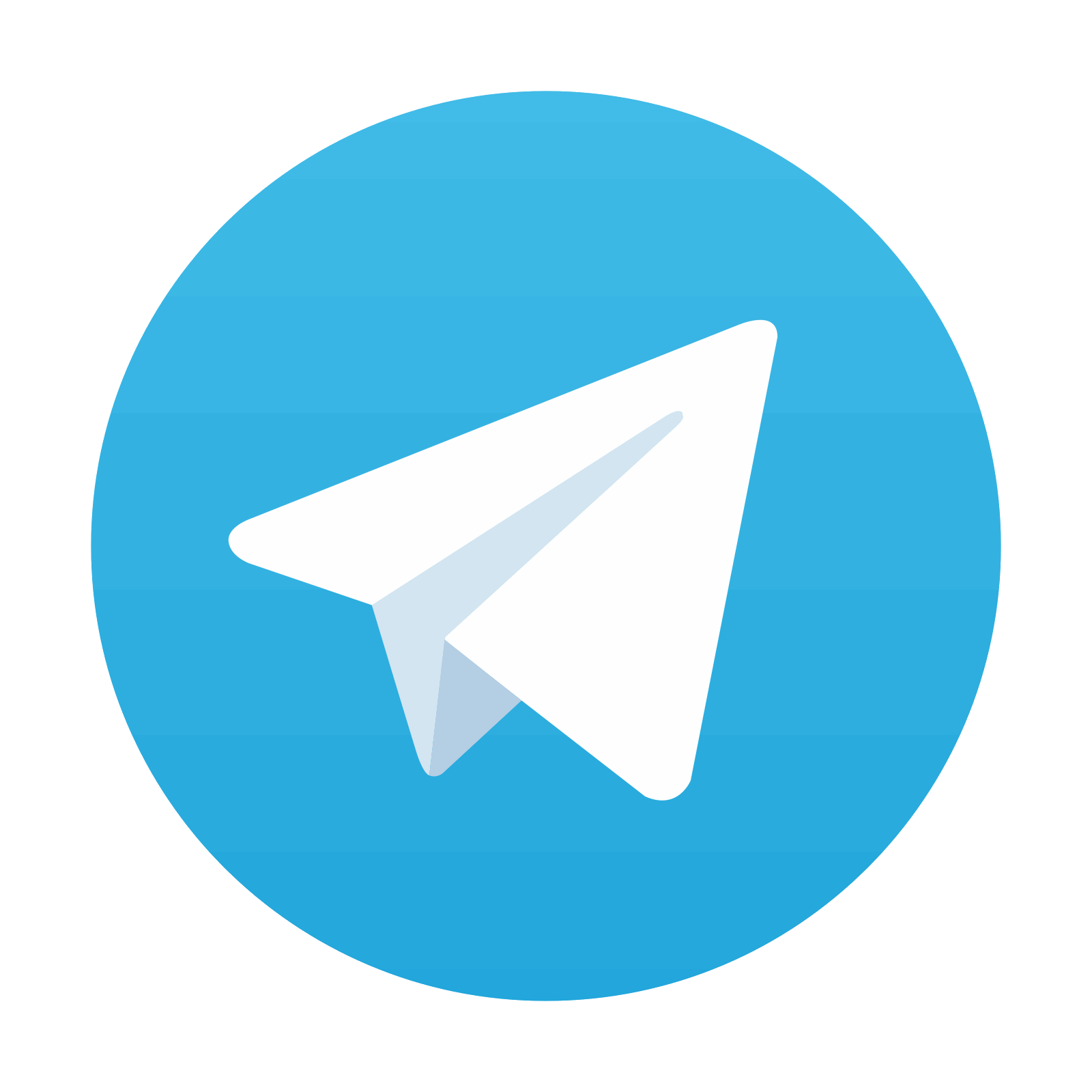
Stay updated, free articles. Join our Telegram channel

Full access? Get Clinical Tree
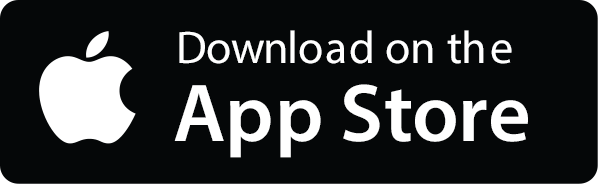
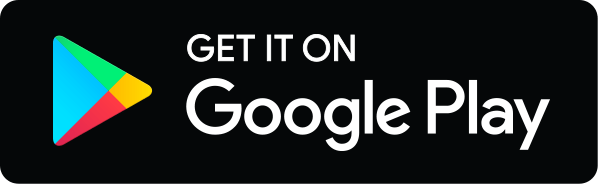