Memory
The next three chapters review important aspects of cognition. Cognition is loosely defined as the ability to do the following:
Attend to external or internal stimuli
Identify the significance of the stimuli
Respond appropriately
This complex processing takes place in the cortices of the brain. It occurs between the arrival of sensory input and the behavioral reaction. In the next two chapters, we discuss intelligence and attention. Here we start with memory.
The ability to store information and access it at some future point is one of the most fascinating aspects of the brain. Indeed, some of the first experiments in psychology were research into learning and memory, which continues to be aggressively studied in modern neuroscience. Learning is defined as new information acquired by the nervous system and observed through behavioral changes. Memory describes encoding, storage, and retrieval of learned information.
TYPES OF MEMORY
Experts in memory have identified different types and subtypes of memory. Many of these subtle distinctions are not relevant for our purposes. One important distinction is separating memory of details from learning procedures. The facts we learn in school or historic events from our lives are called declarative memory (also called explicit memory). This is usually what people are referring to when they speak of memory.
Procedural memory is nondeclarative memory (also called implicit memory or somatic memory). It describes the process of learning a skill or making associations. Examples include learning to ride a bike or playing a musical instrument. This type of memory is outside the conscious thought and actually can deteriorate if one concentrates too hard. Another example includes the exaggerated startle response seen with post-traumatic stress disorder. This reaction is immediate and takes place before the subject is consciously aware of the stimulus.
The importance of separating declarative from nondeclarative memory is that these two types of memory are encoded through different mechanisms in the brain. Likewise, they are disrupted by different central nervous system lesions or disorders—more on this later.
Immediate, Short, and Long
Memories begin to decay as soon as they are formed. The temporal stages of retention are divided into immediate, short term, and long term (Figure 18.1). These are the stages we test in a comprehensive mental status examination. Immediate memory describes the ability to hold a few new facts in mind for a matter of seconds. Looking up a new phone number and successfully dialing the number within seconds is an example of this. We test this function when we ask a person to repeat three objects immediately. Another test of immediate memory is asking a patient to repeat a series of numbers. The average maximum “digit span” is seven numbers plus or minus two.
Short-term memory describes those memories that exist from seconds to minutes. An example of this process is searching the house for a lost item and remembering where you have looked. We test this process when we ask a patient to repeat three objects at 5 minutes. Both immediate and short-term memories are vulnerable to disruption.
Long-term memories are enduring representations that last for days, months, and years: the historic events and facts of our lives. This requires the development of a more permanent form of storage.
The process of moving information from immediate and short-term into long-term memories is called consolidation. The physical representation of memories and the areas of the brain dedicated to this function are the focus of this chapter—what do memories look like in the brain, how are they stored, and how do they fade?
The process of moving information from immediate and short-term into long-term memories is called consolidation. The physical representation of memories and the areas of the brain dedicated to this function are the focus of this chapter—what do memories look like in the brain, how are they stored, and how do they fade?
CELLULAR MECHANISMS
The Canadian psychologist Donald O. Hebb proposed in 1949 that some changes must take place between two neurons for memories to develop. He wrote as follows:
When an axon of cell A is near enough to excite a cell B and repeatedly or persistently takes part in firing it, some growth process or metabolic change takes place in one or both cells such that A’s efficacy, as one of the cells firing B is increased.
This has come to be called Hebb’s postulate and can be more easily stated like this: neurons that fire together, wire together. Almost 60 years of research has affirmed that the brain changes with learning and experience.
ALCOHOLIC BLACKOUTS
Alcoholic blackouts occur when someone has consumed too much alcohol and awakens without memories of what happened the night before. It is believed that the high blood level of alcohol disrupts the consolidation of short-term memories into long-term memories. The drinker failed to preserve lasting images of the party because long-term memories were never formed. This can happen with short-acting hypnotics and benzodiazepines as well.
Amazingly, memories formed before drinking are actually enhanced by alcohol. That is, drinking after learning improves recall compared with not drinking. It is possible that memories already consolidated remain in a more pristine state, because there is less interference from new memories that are never fully formed during the drinking.
Long-term Potentiation
In Chapter 5, we discussed long-term potentiation (LTP), a laboratory procedure with slices of brain tissue that serves as a model for memory formation. In summary:
A series of rapid signals between two neurons results in a greater stimulus in the postsynaptic neuron when normal activity resumes (see Figure 5.6).
Increased activity between two glutamate neurons will open the N-methyl-D-aspartate (NMDA) receptor, which results in molecular signals to the nucleus that induce gene expression (see Figure 5.7).
Gene expression results in structural changes on the neuron such as spine formation on the dendrites (see Figure 5.8).
Protein Synthesis
Real memories in mammals, like LTP, require gene expression and protein synthesis for consolidation. For example, a rat can be taught to quickly find a submerged platform in a tub of water—called a water maze (see Figure 18.2A, B). However, if one group of rats is given intraventricular injections of the protein synthesis inhibitor anisomycin 20 minutes before each test, they cannot remember the location of the submerged platform from one session to the next. These rats spend about the same amount of time each day trying to find the submerged platform (Figure 18.2C). Anisomycin inhibits the production of proteins that are needed to consolidate short-term memories into long-term memories.
Epigenetics
A new line of research suggests that epigenetic mechanisms may play a role in memory formation. As discussed in Chapter 6, acetylation of the histones can open up access to the DNA and allow greater gene expression (see Figure 6.9), and we know gene expression is required for protein synthesis. Several laboratories have shown that fear memory, for example, is associated with acetylation of histone H3 in the hippocampus. Likewise, inhibition of the deacetylase enzymes elevated histone acetylation and enhanced long-term memory formation.
In 2009, a group at the Massachusetts Institute of Technology (MIT) established a link among histone acetylase inhibition, memory formation, and synaptic plasticity. They were able to show with mice that overexpression of the inhibitor (so there was less acetylation, more tightly closed DNA, and less gene expression) resulted in decreased dendritic spine density, synapse number, synaptic plasticity, and memory formation. Conversely, deficiencies of the acetylase inhibitor (more acetylation—more gene expression) resulted in increased synapse number and memory facilitation. These studies establish an epigenetic mechanism for the formation of longterm memories and suggest possible future sites for intervention for those with memory impairment.
Extinction
Extinction is the gradual reduction in the response to a feared stimulus when the stimulus is repeatedly encountered without an adverse experience. For example, a person who is afraid of bridges will have a reduction in fear if they repeatedly cross the bridge without falling off or the bridge collapsing. Extinction is the bedrock of behavioral therapy and one of the most effective treatments for anxiety disorders.
Several lines of evidence suggest that extinction is accomplished through the development of new memories rather than erasing the old memories. Studies have established this by looking at the effect of anisomycin on extinction. Rats given intraventricular injections of anisomycin before repeated exposure to a stimulus (without the negative consequences) fail to show extinction the following day. In other words, the new learning did not erase the old memories.
Faster extinction?
Would faster learning during extinction therapy improve the effectiveness of exposure and response prevention therapy? A group at Emory University is studying just this possibility. Using the understanding of how memories are formed, they proposed that an NMDA agonist might increase the signal to the nucleus, increase the gene expression, and increase learning. The reader should remember that the glutamate neurons (the most common neurons in the brain) have several different types of receptors. Opening the NMDA receptor as well as the α-amino-3-hydroxy-5-methyl-4-isoxazole propionate receptor increases the excitatory postsynaptic potential and the signal to the nucleus (see Figure 5.2).
Researchers at Emory developed a virtual reality simulator that gives participants the sensation of riding an elevator with a glass floor. Patients with fear of heights can extinguish much of their anxiety with many sessions in this device. d-cycloserine, an antibiotic used to treat tuberculosis, is also a partial agonist at the NMDA receptor. d-cycloserine will open the NMDA receptor and the postsynaptic nucleus will receive a heightened signal (see Figure 18.3). The Emory group gave patients d-cycloserine or placebo before just two sessions in the virtual elevator and found a 50% reduction in fear with those on the active medication (Figure 18.3C).
Further research after this study supports the use of d-cycloserine as a “cognitive enhancer” for psychological treatment of anxiety disorders. Studies with social anxiety disorder and panic disorder have shown that D-cycloserine was superior to
placebo when added to cognitive-behavioral therapy. The results for obsessive-compulsive disorder were less impressive. These studies suggest that the right medications administered in conjunction with psychotherapy may improve the learning that seems essential for effective psychological treatment. This may lead to a whole new approach to treating mental illness where exposure, behavior, and cognitive and talking therapies are paired with the appropriate medication or device to produce faster, better, or more durable therapeutic changes.
placebo when added to cognitive-behavioral therapy. The results for obsessive-compulsive disorder were less impressive. These studies suggest that the right medications administered in conjunction with psychotherapy may improve the learning that seems essential for effective psychological treatment. This may lead to a whole new approach to treating mental illness where exposure, behavior, and cognitive and talking therapies are paired with the appropriate medication or device to produce faster, better, or more durable therapeutic changes.
Structural Plasticity
Some memories last an entire lifetime. These longterm memories persist despite surgical anesthesia, epileptic seizures, and drug abuse. Protein molecules are not stable enough to survive these insults. Therefore, long-term memories must be the result of more stable formations such as structural changes (as seen with LTP) or they might be continuously rebuilt throughout one’s life. In Chapter 8, we discussed several examples of cortical strengthening secondary to learning and practicing, for example, monkeys spinning a wheel (see Figure 8.13) and humans playing a musical instrument (see Figure 8.15). These studies show that the cortex changes with learning.
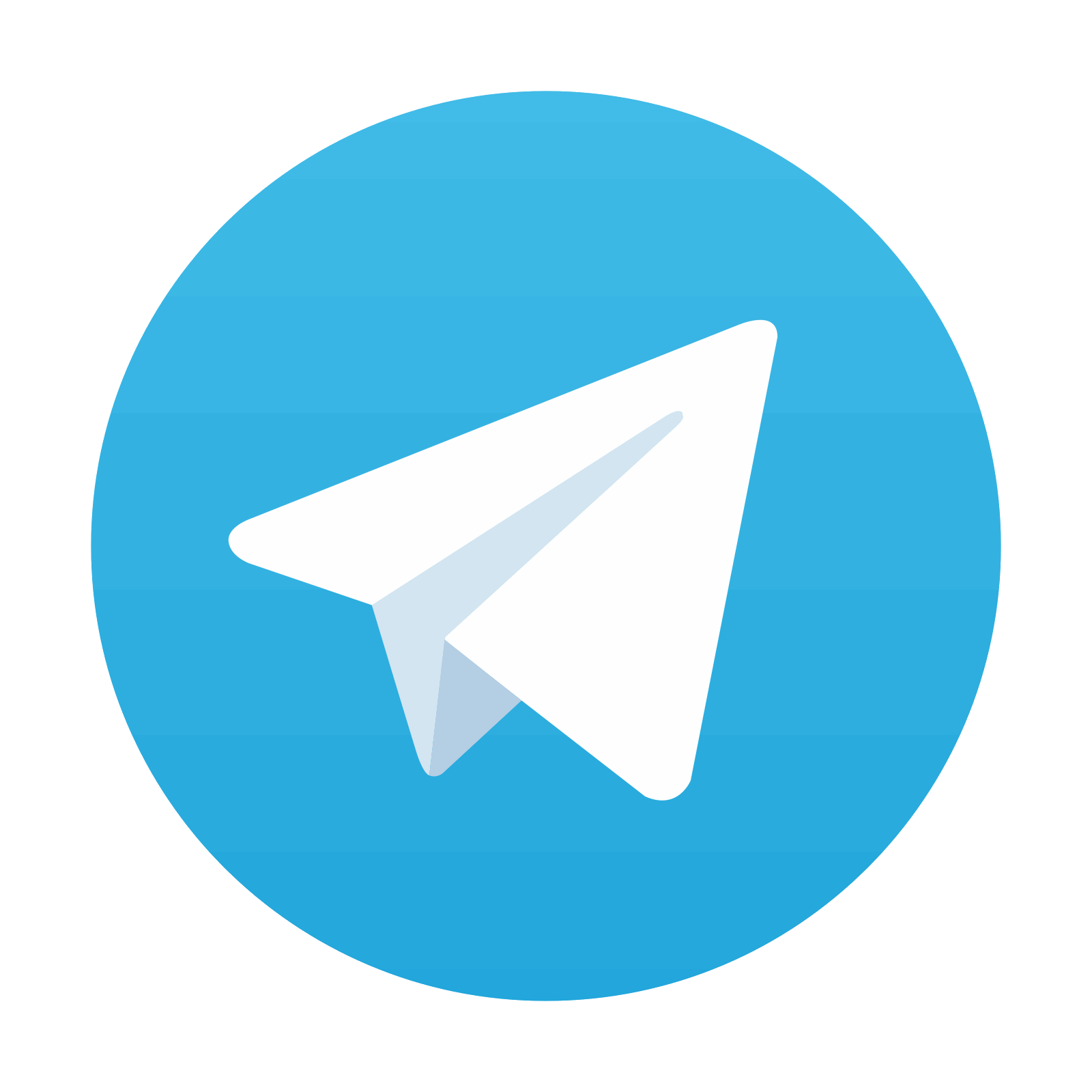
Stay updated, free articles. Join our Telegram channel

Full access? Get Clinical Tree
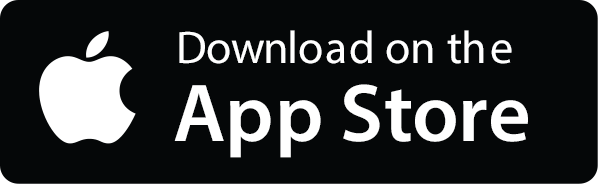
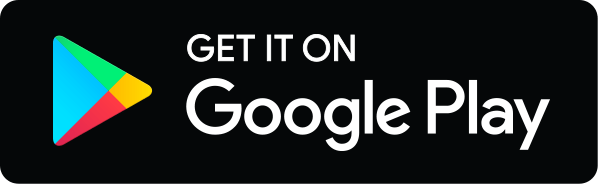