At “usual” body weight, there is little difference (F) between actual energy stores, predominantly adipose tissue, and a centrally perceived “ideal.” Perturbations in F induce changes in both energy intake and output as well as in partitioning of stored calories, which act coordinately to restore calorie storage to the ideal, i.e., the point at which F = 0.
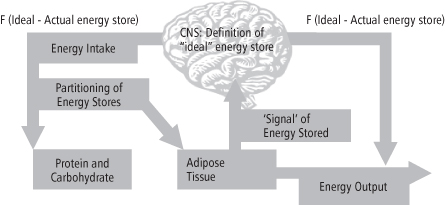
Genetic Influences on Body Fatness
The heritability (the fraction of the variance in a given trait within a population that is attributable to genes) of obesity can be examined by looking at monozygous (identical) vs. dizygous (fraternal) twins. This assumes that the twins, whether identical or fraternal, share the same environment. Therefore, any greater similarity of the trait (in this case, body fatness) that exists in monozygous vs. dizygous pairs can be attributed to the additional 50% of the genotype shared by the monozygotes. Using this type of calculation, the heritability of body fatness is found to be between 65% and 85%, i.e., the same as the heritability of height and greater than the heritability of many diseases such as schizophrenia and breast cancer that are commonly thought of as “inherited” (see Table 20-1). Heritability of body type can also be examined by comparing adoptees with their biological vs. their adoptive parents. This type of study reveals a high correlation of body fatness of adoptees with that of their biological parents (50% shared genotype with each parent, no common environment) and no significant correlation with their adoptive parents (no shared genotype, shared common environment) (5–7).
Table 20-1 Heritability* Estimates Based on Studies of Body Fatness vs. Other Medical Conditions
Condition | Heritability (G±SEM) |
Childhood body fatness (age 7) | 0.77 ± 0.17 |
Adult body fatness (age 45) | 0.64 ± 0.13 |
Schizophrenia | 0.68 ± 0.14 |
Hypertension | 0.57 ± 0.25 |
Epilepsy | 0.50 ± 0.33 |
*Heritability: the proportion of the variance in a trait in the population that can be attributed to genes.
Adapted from ref. (5).
The heritability of body fatness in this context does not refer to absolute levels of body weight or body composition. What is heritable is the rank order of body fatness within a given population of similar lifestyle peers. Thus, if both parents are at the 75th percentile for body fatness compared to individuals with a similar lifestyle, then it is likely that their offspring will also fall at approximately that percentile. The absolute level of body fatness is determined by the interaction of a genetic proclivity towards relative body fatness with the population/lifestyle in which that proclivity is allowed to express itself (8).
There are also significant genetic influences (heritability greater than 30%) on multiple behaviors and physiological measures of energy expenditure relating to body weight homeostasis and adiposity-related morbidity. The list includes body fat distribution, resting metabolic rate, feeding behavior, food preferences, and changes in energy expenditure that occur in response to overfeeding (9, 10).
The pivotal role of genetics in the control of body weight is confirmed by the existence of single gene mutations capable of producing profound increases in body fat content. Some are “syndromic” (e.g., Prader-Willi, Bardet Biedl, Ahlstrom, Cohen), in association with characteristic phenotypes in addition to obesity (11). However, in most humans, body fatness is a continuous quantitative trait reflecting the interaction of genotype with development and environment.
Systemic Energetics
Medical cognoscenti of the 18th century maintained that living organisms possessed a vitalistic energy that inanimate objects did not. The scientific study of bioenergetics began in 1773 with the work of Lavoisier and LaPlace who shattered these vitalistic views with their demonstration of the chemical identity of combustion and respiration. In the ensuing 225 years, a distinguished succession of physicists, chemists, and physiologists established the principles of thermodynamics and their applicability to biological systems.
In all studies of bioenergetics, the first law of thermodynamics is inviolably applicable:
Any change in the energy stored (more than 100,000 kcal in a 70 kg man) must reflect a difference between energy taken in as food and energy expended in various forms of work (see below). If energy intake and output were not regulated by interlocking control mechanisms that work concordantly to maintain energy stores, then a very small perturbation in this equation would, over time, lead to substantial gain or loss of stored calories. Reducing intake by 150 kcal/day (an 8 oz glass of whole milk) below energy expenditure would result in a net decrease of approximately 55,000 kcal of stored energy, or about 12 kg of weight, per year. Yet, the average US adult gains only 0.5–1 kg of weight per year (more in older individuals, African Americans, Native Americans, and Hispanic Americans) (12). The remarkable constancy of body weight and composition over long periods of time, despite ingestion of approximately 900,000–1,000,000 kcal/year, suggests that energy intake and expenditure vary directly such that when energy expenditure is increased there is a corresponding increase in energy intake to maintain constant energy stores. The responses of metabolic, neuroendocrine, and autonomic systems in the setting of attempts to sustain weight loss demonstrate the potency of redundancy of the systems that favor regain of lost weight.
The integrated function of systems regulating energy intake and expenditure is evident in the inability of individuals who are successful at weight reduction to maintain a reduced body weight. Long-term studies of weight-reduced children and adults have shown that 80–90% return to their previous weight percentiles. Studies of those successful at sustained weight loss indicate that the maintenance of a reduced degree of body fatness probably requires a lifetime of attention to energy intake and expenditure (13–16).
Numerous studies of the physiology, neuroendocrinology, and molecular biology of body weight regulation indicate that control of energy stores is achieved by coordinated regulation of energy intake and expenditure mediated by signals emanating from adipose, gastrointestinal, and endocrine tissues, and integrated by the liver and by central nervous system functions ranging from the most basic hypothalamic brainstem functions to the most complicated higher cortical functions (see Table 20-2).
Table 20-2 Summary of Approximate Changes in Factors Related to Energy Expenditure (A), Autonomic Functions (B), and Neuroendocrine Functions (C) in Individuals Maintaining a Reduced Body Weight (3)
A. Components of energy expenditure normalized to body composition: | |
24-hour energy expenditure | Decreased (∼ –15%) |
Resting energy expenditure | Decreased or unchanged |
Thermic effect of feeding | Unchanged |
Non-resting energy expenditure | Decreased (∼ –30%) |
Skeletal muscle efficiency | Increased (−20%) |
B. Autonomic Nervous System Tone | |
Sympathetic nervous system tone | Decreased (∼ –40%) |
Parasympathetic nervous system tone | Increased (∼80%) |
C. Neuroendocrine Function | |
Thyroid stimulating hormone | Decreased (∼–18%) |
Triiodothyronine | Decreased (∼–7%) |
Thyroxine | Decreased (∼–9%) |
Cortisol | Increased or unchanged |
Gonadotropins | Decreased, especially at very low fat mass |
Leptin | Decreased in proportion to fat lost |
Short-term (daily) food intake is not closely correlated to energy expenditure or energy stores in adults (17) or children (18). Accordingly, some means of integrating these short-term changes in energy balance over longer periods of time is needed to account for the overall stability of body weight. Thus, longer-term effects on food intake operate to integrate signal(s) regarding somatic energy stores with ingestive behavior and energy expenditure. The interlocking control mechanisms preserving energy stores via longer-term sensing are illustrated in studies of children given ad libitum access to a variety of foods in a controlled research setting or using a non-energy fat substitute in lieu of fat. With presumably no knowledge of nutrition, these children would ingest approximately the same daily calorie intake of self-selected foods that were different each day (18, 19). While the short-term, meal by meal, calorie content varied widely, there was little difference in longer-term calorie intake.
Some investigators have hypothesized that this integration of energy intake and output is achieved by direct CNS “sensing” of some signal reflective of body fat stores. Perturbations in this signal result in downstream alterations in systems regulating energy intake and output (neuroendocrine, autonomic, and metabolic functions) so as to maintain body energy stores constant over time (3, 20, 21). Others have suggested that the long-term constancy of body weight is mediated by fuel fluxes (circulating concentrations of fatty acids, amino acids, glycerol, or glucose; hepatic glycogen stores; or rates of hepatic oxidative metabolism) resulting indirectly from changes in fat mass (22–25). These hypotheses are not mutually exclusive and both are probably correct. Regardless of the underlying molecular mechanisms, it is clear that the systems regulating energy intake and output are synergistic and redundant at almost every level from the adipocyte to the cerebral cortex.
Energy Homeostasis Following Weight Reduction
In his book The Fire of Life, Max Kleiber proposed that every animal could be placed on the same regression line relating an approximation of lean body mass (i.e., the energy-consuming tissues of the body, especially skeletal muscle and brain tissue) to 24-hour energy expenditure (26). In agreement with Kleiber’s view, there is little difference in 24-hour energy expenditure (TEE, weight maintenance calories) normalized to body composition between otherwise healthy lean and obese individuals at “usual” weight (27). However, this relationship does not hold true when comparing someone who is maintaining a reduced body weight to someone of the same body composition without any perturbations from usual weight.
Almost anyone who has ever intentionally lost weight will report that there is even greater difficulty in sustaining weight loss than in losing it in the first place. The problem is not only creating a state of energy expenditure that is greater than energy intake, in order to decrease body calorie stores and lose weight. It is also to maintain a balance of energy intake and expenditure following weight loss to avoid weight regain. Studies of the responses of subjects to experimental perturbations in body weight indicate that body weight is regulated against a centrally perceived “ideal” by similar, if not identical, means in lean and obese individuals. There is potent “opposition” to the maintenance of reduced body weight.
Maintenance of a 10% or greater reduction in body weight in lean and obese individuals is associated with an approximately 20–25% decline in 24-hour energy expenditure, i.e., a decline in weight maintenance calories of 10–15% beyond what is predicted solely on the basis of fat and lean mass lost (27–30). Thus, a formerly obese individual will require approximately 300–400 fewer kcal/day to maintain the same body weight as a never-obese individual of the same body weight and composition.
As noted above, studies of individuals successful at sustaining weight loss indicate that reduced weight maintenance requires long-term lifestyle alterations including dietary restriction, increased exercise, and decreased time spent watching television compared to individuals who are “naturally” at the same weight (13–15). The necessity for these long-term changes is consistent with the observation that the reduction in TEE persists in subjects who have sustained weight loss for extended periods of time (6–24 months) in circumstances of enforced calorie restriction, in the biosphere 2 project (31), through lifestyle modification (32, 33), or from bariatric surgery (34). As discussed below, attempts to sustain weight loss are met by declines in energy expenditure and increases in hunger that do not appear to abate over time (32, 33, 35).
Changes in Energy Expenditure Following Weight Reduction
Twenty-four hour energy expenditure (TEE) is the sum of resting energy expenditure (REE, cardiorespiratory work and the work of maintaining transmembrane ion gradients at rest; approximately 60% of TEE), the thermic effect of feeding (TEF. the work of digestion; approximately 5–10% of TEE), and non-resting energy expenditure (NREE, energy expended in physical activity above resting; approximately 30–40% of TEE). Partitioning of the effects of reduced weight maintenance on each of these components of energy expenditure also suggests the type and amount of long-term lifestyle changes that might be most helpful in keeping weight off.
Some studies (36–39) have reported no changes in REE following weight loss, while others have reported that the maintenance of a reduced body weight is associated with a reduction in REE accounting for about 10–15% of the decline in TEE beyond that predicted on the basis of weight lost (27, 29, 30, 38, 40, 41). There is no significant decline in TEF following weight loss (27–29). Therefore, NREE is the compartment of energy expenditure that is most affected by changes in body weight (27, 29), as is also indicated by the importance of regular exercise in those who are successful at weight maintenance after weight reduction (14, 15).
The pre-eminence of NREE in accounting for as much of 85–90% of the decline in TEE below predicted values in weight-reduced subjects (29, 42) could be due to declines in the amount of physical activity performed. In rodents, maintenance of a reduced body weight is associated with an increase, rather than decrease, in the amount of time spent in physical activity (43). Similarly, studies of humans following weight loss have revealed no change or as much as a 30% increase in the amount of time that subjects spend moving each day (27, 39).
Since maintenance of reduced weight is not associated with a decline in the amount of physical activity performed, the decreased energy expended in physical activity must reflect significantly increased efficiency with which that activity (both exercise and non-exercise activities such as fidgeting) is performed. Studies of skeletal muscle chemomechanical efficiency (calories expended above resting per unit of work performed) in in-patient weight-reduced subjects indicate that maintenance of a reduced body weight is associated with approximately 20% increase in skeletal muscle work efficiency at low levels of exercise whether measured by whole-body ergometry, NMR spectroscopy during exercise, or the ATP consumption during a single muscle fiber contraction (29). Outpatient studies by Weinsier and colleagues indicate that women maintaining a 10% reduction in weight are able to increase the amount of time spent each day in physical activity without increasing NREE, supporting the view that skeletal muscle work efficiency is increased (44, 45) following weight loss. Since these effects are most evident at low levels of work (i.e., those commensurate with activities of daily living) it can be presumed that some of the opposition to reduced weight maintenance can be diminished by exercising at higher workloads (29, 46).
Neuroendocrine Function
The regulation of food intake and body weight is accomplished with the participation of numerous neural pathways in the brain which act on or interact with hormones produced by organs and tissues in the body. The neuroendocrine changes potentially associated with the maintenance of a reduced body weight include increased activity of the hypothalamic-pituitary-adrenal (HPA) axis and decreased activity of the hypothalamic-pituitary-thyroid (HPT) and hypothalamic-pituitary-gonadal (HPG) axes, and decreased circulating concentrations of leptin (see below). The hypothalamic pro-opiomelanocortin (POMC)-melanocortin-4 receptor (MC4R) pathway, by virtue of its constituent neuronal outflow tracts to the ANS, neuroendocrine axes, and cortical tracts subserving food intake, may provide a central nexus for summing the integrated effects on energy expenditure and intake that are seen following weight loss (3, 47–49).
The importance of the HPA axis in regulating body fat stores is illustrated by the effects of adrenalectomy on genetically obese rodents. The leptin-deficient or leptin-resistant mouse is hyperphagic, hypometabolic (much like the weight-reduced human discussed below), and ultimately obese and diabetic. These phenotypes are abolished by chemical or surgical adrenalectomy (50, 51). Hypercortisolemia results in loss of lean body mass and increases the storage of calories as fat (52). Studies of the HPA axis in which subjects were assessed following variable weight loss regimens and lengths of time maintaining a reduced weight have reported increases (53), decreases (54), and no change (55, 56) in indices of cortisol production following weight loss. Discrepancies among studies of the effects of reduced weight on the HPA axis may reflect differences in subject populations, weight loss regimens, or degrees of weight stability.
Thyroid hormone acts to increase energy expenditure by increasing heart rate, blood pressure, and muscle ATP consumption (largely by stimulating production of muscle ATPase), and decreases hepatic glycogen stores (potentially favoring the oxidation of fat as fuel since less carbohydrate is available). The thyroid hormone-deficient patient is hypotensive, bradycardic, and lethargic and tends to gain weight, while the hyperthyroid patient is hypertensive and tachycardic and tends to lose weight (57–62). Both weight loss and the maintenance of a reduced body weight are associated with small but significant decreases in the circulating concentration of triiodothyronine (T3) and increases in the circulating concentration of its bioinactive enantiomer, reverse T3 (rT3), suggesting that weight loss results in increased peripheral conversion of thyroxine (T4) to rT3 (63, 64). The release by the pituitary of thyroid stimulating hormone (TSH) upon administration of thyroid-releasing hormone is not diminished during caloric restriction (65) or after weight loss (66) in humans. The lack of increase in TSH with weight loss, despite the decrease in circulating concentrations of T3, indicates that hypothalamic TRH release is decreased following weight loss. Decreased hypothalamic alpha-MSH (α-MSH), a proteolytic product of POMC, decreases activity of hypothalamic pro-thyroid releasing hormone (pro-TRH) neurons in rats (67), thus providing a possible mechanism for the decrease in HPT axis activity following weight loss (68).
There are many other signals relevant to fuel homeostasis, in particular those originating in the gut. Some of these signals, like insulin, not only affect appetite but also the partitioning of stored fuel. Many of these signals originate in the gastrointestinal tract and are probably more relevant to regulation of short-term intake, responding quickly to cues such as gastric distention with more subtle long-term effects following weight reduction (69, 70). Signaling systems regulating short-term energy intake and output are discussed in Chapter 8.
Autonomic Nervous System Function
The autonomic nervous system (ANS), both parasympathetic and sympathetic, includes major neural outflow tracts linking afferent biochemical signals regarding energy stores to efferent tracts regulating energy expenditure via the CNS’s regulating body weight. Increased parasympathetic nervous system (PNS) activity slows the heart rate and decreases resting energy expenditure. The sympathetic branch (SNS) of the autonomic nervous system modulates feeding behavior, directly increases heart rate, and increases the rate of secretion of thyroid hormone (71–74). Sympathetic denervation of the arm in humans with palmar hyperhidrosis improves skeletal muscle work efficiency (75), and deactivation of sympathetic neurons attenuates leptin-mediated increases in energy expenditure in rats (76). Daily urinary norepinephrine excretion accounts for a significant proportion of the variance in energy expenditure and its subcomponents.
The maintenance of a reduced body weight is associated with significant declines in SNS tone (whether measured by analysis of heart rate variability or 24-hour urinary catecholamine excretion) and increases in PNS tone (63, 74, 77, 78). Changes in ANS tone associated with weight loss, in particular the decline in sympathetic nervous system tone, may account for a significant fraction of the hypometabolic state through direct effects on skeletal muscle, or indirectly via effects on circulating concentrations of thyroid hormones (63, 79–82). Thus, weight-loss mediated changes in the autonomic nervous system may link weight-loss-associated changes in energy to neuroendocrine homeostasis.
Energy Intake
Decreased energy expenditure following weight loss would have little consequence if it was easy to sustain a corresponding reduction in energy intake in order to maintain a reduced body weight. However, this is not the case, as anyone who has attempted to sustain a reduced body weight will attest. Individuals maintaining a reduced body weight are inclined toward greater rather than diminished energy intake (83). They experience delayed satiation and do not diminish the number of calories per meal despite the decrease in energy expenditure following weight loss (84, 85).
Functional magnetic resonance (fMRI) signals obtained as subjects are shown food vs. non-food items reflect activation/suppression of neuronal activity in regions throughout the brain that mediate vegetative as well as hedonic and executive responses to food (86). Following weight reduction, characteristic fMRI changes occur that are consistent with current understanding of the contributions of specific brain regions/circuits to responses to food. They suggest that in weight-reduced subjects there may be an increase in cognitive and emotional responses to food, coupled with decreased activity in brain areas associated with restraint and control of food intake (86). The combination of decreased energy expenditure and an increased drive to consume energy create “the perfect storm” for weight regain following otherwise successful weight loss. As noted above, studies of individuals successful at sustained weight loss indicate that they must maintain significantly greater levels of exercise (energy expenditure) and significantly lower daily energy intake compared to weight-matched never-overweight controls in order to sustain their weight loss (14, 15, 87, 88). fMRI studies demonstrate significantly greater activity in brain areas involved in restraint and control of food intake in individuals able to sustain a reduced weight, i.e., the same brain areas that tend to diminish in activity after weight loss (89, 90). It is unclear if this increased activity in brain areas involved in restraint in response to food can be learned or if these are individuals in whom this trait was present prior to weight loss.
Leptin in Energy Homeostasis
Leptin is an adipocyte-derived molecule that circulates in weight-stable individuals in direct proportion to fat mass (91, 92). The hyperphagic, hypometabolic phenotype of weight-reduced humans is similar to that of leptin-deficient or leptin-unresponsive humans and rodents (93, 94). Circulating leptin concentrations are inversely correlated with hunger ratings in humans during weight loss, independent of the amount of weight or body fat lost (95, 96). Leptin administration reverses the hyperphagia associated with leptin deficiency in leptin-deficient mice and humans (97–99) and acts synergistically with sibutramine to reduce food intake in rodents (100). At the level of neuroendocrine function, leptin exerts a stimulatory effect on POMC production and an inhibitory effect on neurons expressing the potent anorexiant neuropeptide Y (NPY) and agouti-related peptide (AgRP). As schematized in Figure 20-1, assuming that leptin is a prominent “signal” to the brain regarding fat stores, decreased leptin as a result of reduced fat mass would have the net effect of stimulating food intake via both the POMC and the NPY/AgRP axes (24).
The hypothalamic POMC-melanocortin-MC4R pathway is highly sensitive to circulating leptin concentrations and POMC expression is decreased in low-leptin states (48, 101). Briefly, POMC is cleaved to from alpha-melanocyte stimulating hormone (α-MSH) and beta-endorphin (β-EP). α-MSH stimulates release of hypothalamic pro-TRH, while β-EP inhibits the release of hypothalamic corticotropin releasing factor (CRF). Therefore, one would predict that a low leptin state, such as that induced by weight loss, would be associated with decreased HPT and increased HPA axis activity. Mice overexpressing the MC4R antagonists agouti or agouti-related peptide (AgRP) (102), as well as rodents and humans with mutations in MC4R (103) or disruptions of POMC gene expression (104, 105) or of prohormone processing (106, 107), are obese. The importance of leptin in mediating these effects is shown in the observation that fasting in rodents causes hypoleptinemia which is associated with increased arcuate NPY and AgRP mRNA expression and decreased hypothalamic POMC mRNA in lean animals, but not in leptin-receptor deficient animals (101, 108, 109).
Administration of leptin to leptin-deficient rodents and humans in doses that restore circulating leptin concentrations to their physiological range increases energy expenditure (110, 111), decreases energy intake, increases sympathetic nervous system output (112, 113), and normalizes hypothalamic-pituitary-adrenal, thyroid, and gonadal function (48, 97, 99, 114–118). Yet, in humans and rodents who are not leptin-deficient, induction of weight loss requires doses of leptin that produce plasma leptin concentrations over 10 times normal (118, 119). Recent studies of the short-term administration of leptin to weight-reduced lean and obese subjects suggest that restoration of circulating concentrations of leptin to levels present prior to weight loss reverses the hypometabolism, decline in thyroid hormones, decline in SNS tone, as well as the delayed satiation and fMRI changes in response to food that characterize the weight-reduced state (83, 120). In this sense, the weight-reduced state may be perceived by CNS tracts relevant to energy homeostasis as a state of relative leptin deficiency. In this context, pharmacotherapy activating the leptin-signaling pathway may prove to help weight-reduced individuals sustain their weight loss.
Implications for the Management of Weight Reduction
The potency and long-term nature of the physiological opposition to the maintenance of a reduced body weight has a number of implications directly relevant to the practice of bariatrics. All are based on the observation that the inability to sustain weight loss is, in most cases, a “biological” problem due to energy homeostatic systems that are present in both lean and obese individuals. It is not the product of “psychological” sloth and gluttony. The same processes that make it so difficult for a slender person to keep off 5 kg to fit into last summer’s bathing suit are operant and oppositional in the heavier individual who is attempting to lose and keep off 10 kg to resolve their hypertension.
The maintenance of a reduced body weight is potentially a lifetime undertaking. Rectification of the metabolic compensations for weight loss require restoration of a “eumetabolic” state rather than the imposition of a “hypometabolic” state necessary to induce weight loss. Therefore, therapeutic strategies to maintain a long-term eumetabolic state to sustain weight loss will be distinctly different from those used to induce the temporary hypometabolic state necessary to cause weight loss. Knowing this, anyone who sustains even a small degree of weight reduction, and the health benefits that go along with this, for an extended period of time is deserving of the utmost admiration and respect. This is irrespective of whether they conform to some societal standard of cosmetic thinness.
Summary
Attempts to sustain weight loss invoke a phenotype that has evolved over many millennia to defend energy stores. This phenotype is distinct from that opposing dynamic weight loss per se. The multiplicity of systems regulating energy stores and resisting the maintenance of a reduced body weight illustrate that obesity is a disease in which the human body actively opposes the cure over long periods of time beyond the initial resolution of symptomatology.
The prevalence of obesity in the US is increasing at the rate of 30–40% per decade. This “fattening” in the presence of vigorous and redundant systems regulating body weight emphasizes that obesity is the product of interactions between genetic proclivities to store calories as fat and an environment that favors the consumption of a calorie-dense diet and the maintenance of a sedentary lifestyle. The potent environmental influences on adiposity in the setting of a relatively static genotype indicate that obesity is treatable through alterations of the environment. As noted above, what is heritable is the rank-order of body fatness of a given individual within the context of a peer group of similar lifestyle. That lifestyle and peer group are malleable and can have potent effects on the likelihood of sustaining weight loss is evident from studies of individuals who have successfully maintained a reduced weight and whose success is predicated on essentially “reinventing” themselves. This “reinvention” typically includes long-term decreased energy intake and increased exercise and often includes significant changes in their social milieu to maintain those changes (14, 15, 87).
Summary: Key Points
- Twin studies comparing monozygous to dizygous twins and studies of adoptees compared to their adoptive as well as their biological parents suggest that body fatness is a heritable trait
- The existence of single gene mutations that produce profound increases in body fat content is another line of evidence that body fat is an inherited trait.
- In most humans, body fatness is a continuous quantitative trait reflecting the interaction of genotype with development and environment.
- The energy balance equation is expressed as:
- Numerous weight loss studies in children and adults suggest that 80%-90% return to their previous weight percentiles. This inability to maintain lost weight is interpreted to suggest that energy stores (fat stores) are defended by biological mechanisms. These may include signals emanating from adipose, gastrointestinal and endocrine tissues, and integrated by the liver and central nervous system.
- Studies show decline in total energy expenditure after weight loss that is greater than can be explained by the amount of fat and lean mass lost. Thus, there is a difference between a weight-reduced individual and a matched individual of identical weight and body composition who was never obese.
- There are multiple physiological changes in both the brain and periphery that accompany weight loss and favor weight regain.
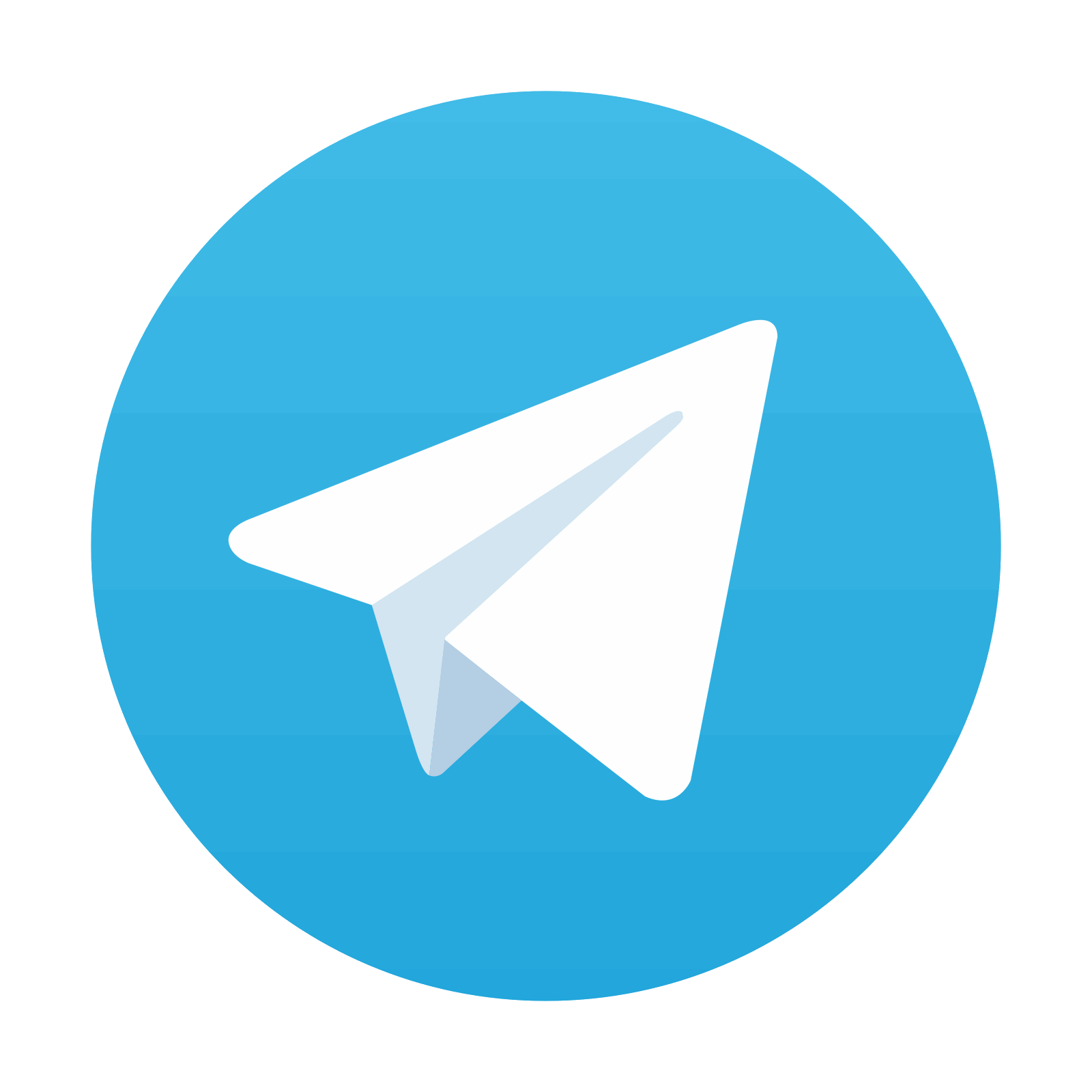