The inherited metabolic myopathies are traditionally classified by their underlying biochemical abnormalities as disorders of (1) carbohydrate, (2) lipid, and (3) adenine nucleotide metabolism. A fourth possible category includes the mitochondrial encephalomyopathies. As mitochondrial disorders do not cause defects in a specific biochemical pathway, they are discussed in a separate chapter. The immediate source of energy for muscles comes from the hydrolysis of adenosine triphosphate (ATP). At rest, the major substrate for muscle in terms of ATP production comes from the metabolism of long-chain fatty acids. Therefore, any disorder impairing β-oxidation of long-chain fatty acids in the mitochondria can lead to a myopathy. During exercise, ATP is derived from the metabolism of carbohydrates, fatty acids, and ketones. Early in the course of exercise (e.g., up to 45 minutes), energy is derived mainly from free glucose or glucose made available via glycogenolysis. Subsequently, there is a shift toward the metabolism of fatty acids such that after a few hours 70% of energy is derived from lipid breakdown.
Metabolic myopathies can also be viewed as static or dynamic disorders. The static myopathies are defined by the presence of fixed or progressive weakness. On the other hand, the dynamic myopathies are associated with exercise intolerance (i.e., exertional myalgias, cramps, and myoglobinuria) as the dominant clinical features. Some metabolic defects are associated with both a dynamic and a static myopathy.
Carbohydrates are stored in liver and muscle as glycogen, a highly branched polymer of glucose. Normal synthesis and breakdown of glycogen is essential to maintain adequate glucose concentration in muscle that can be further metabolized and provide energy in the form of ATP. There are 16+ recognized glycogen storage diseases (GSDs), also called glycogenoses (Table 29-1). This is somewhat a misnomer because some of these glycogenoses do not result in the accumulation of glycogen in tissues.
Disorder | Enzyme Defect | Inheritance | Clinical Features |
---|---|---|---|
Type 0 | Glycogen synthase 1 | Autosomal recessive | Childhood onset weakness, hypertrophic cardiomyopathy |
Type I (von Gierke disease) | Glucose-6-phosphate | Autosomal recessive | No neuromuscular signs or symptoms |
Type II (Pompe disease) | Acid maltase (α-1,4-glucosidase) | Autosomal recessive | Infancy: hypotonia, cardiomyopathy, respiratory failure, generalized weakness Childhood–adult: progressive weakness, respiratory failure |
Type III (Cori–Forbes disease) | Debranching enzyme (amylo-1,6-glucosidase) | Autosomal recessive | Infancy: hypotonia, generalized weakness Childhood/adult: proximal or distal weakness, spasticity, dementia, incontinence |
Type IV (Anderson disease) | Branching enzyme (amylo-1,4–1,6-transglucosidase) | Autosomal recessive | Infancy: hypotonia, generalized weakness Childhood/adult: proximal or distal weakness |
Type V (McArdle disease) | Myophosphorylase | Autosomal recessive | Infancy: rare weakness Childhood/adult: Exercise intolerance, rare weakness |
Type VI | Liver phosphorylase | Autosomal recessive | No muscle involvement |
Type VII (Tarui disease) | Phosphofructokinase | Autosomal recessive | Childhood: exercise intolerance, rare weakness |
Type IXB | aPhosphorylase b kinase B subunit | Autosomal recessive | Infancy to adult: exercise intolerance, rare weakness |
Type IXD | aPhosphorylase b kinase A subunit | X-linked | |
Type X | Phosphoglycerate mutase | Autosomal recessive | Childhood–adult: exercise intolerance |
Type XI | Lactate dehydrogenase | Autosomal recessive | Childhood–adult: exercise intolerance |
Type XII | Aldolase A | Autosomal recessive | Infancy–childhood: exercise intolerance and weakness |
Type XIII | β-Enolase | Autosomal recessive | Childhood–adult: exercise intolerance |
Type XIV | Phosphoglucomutase | Autosomal recessive | Childhood: exercise intolerance and weakness |
Type XV | Glycogenin 1 | Autosomal recessive | Childhood onset of weakness |
Other GSDs | |||
Phosphoglycerate kinase 1 | X-linked | Childhood: exercise intolerance, rare weakness, hemolytic anemia, mental retardation, seizures | |
Triosephosphate isomerase | Autosomal recessive | Infancy: hypotonia, generalized weakness mental retardation |
The glycogenoses predominantly affect liver and muscle. Since there is differential metabolism of carbohydrates in these two tissues, the individual GSDs may produce strictly liver or muscle disease, or some combination of the two. Types I (glucose-6-phosphatase deficiency) and VI (liver phosphorylase deficiency) only cause liver disease and are not further discussed. Types II (lysosomal α-glucosidase deficiency), V (phosphorylase deficiency), VII [phosphofructokinase (PFK) deficiency], X [phosphoglycerate mutase (PGAM) deficiency], and XI (lactate dehydrogenase deficiency) produce almost exclusively muscle disease, while the remaining types produce a varying mixture of muscle disease with systemic disease.
The pathophysiological basis by which the varied enzymatic defects lead to muscle dysfunction remains largely unknown. The inability to metabolize a substrate reduces the ability of muscle cells to form ATP necessary for normal energy production. Further, the enzymatic defects may result in accumulation of metabolites, which may be toxic to muscle.
The exercise forearm test can be helpful diagnosing various disorders of glycolysis. The test can be adequately performed without blood pressure cuff insufflation. In fact, performing this test with the limb ischemic may be hazardous to the patient because it can induce profound muscle damage and myoglobinuria.1 A butterfly needle is placed in the antecubital fossa and draw baseline lactate and ammonia levels are drawn. The forearm muscles are then exercised by having the patient rapidly and strenuously open and close the hand for 1 minute. Immediately after exercise and then 1, 2, 4, 6, and 10 minutes postexercise, blood samples are again taken and analyzed for lactate and ammonia. The normal response is for lactate and ammonia levels to raise three to four times the baseline levels. If neither the lactate nor the ammonia level increases, the test is inconclusive and implies that the muscles were not sufficiently exercised. A rise in lactate levels, but not ammonia, is diagnostic for myoadenylate deaminase (MAD) deficiency. In myophosphorylase, PFK, PGAM, phosphoglycerate kinase (PGK), phosphorylase b kinase (PBK), debranching enzyme, and lactate dehydrogenase deficiencies, the ammonia levels rise appropriately, but the lactic acid does not.
Only a few patients have been reported with this rare disorder.2–4 Affected individuals presented with childhood onset of exercise intolerance and recurrent attacks of syncope of presumed cardiac origin. Prolonged QT syndrome and sudden cardiac death have occurred. However, loss of consciousness was gradual in one patient suggesting metabolic dysfunction within the central nervous system (CNS) as opposed to pure cardiac etiology.4 Mild proximal weakness may be evident.
One report noted that exercise forearm test shows failure of lactate elevation and skeletal muscle magnetic resonance imaging (MRI) shows fatty degeneration of the gluteal and flexor muscles of the thigh.4 Serum creatine kinase (CK) levels have not been reported.
Skeletal muscle and cardiac muscle as well as skin fibroblasts have depletion of glycogen. Phosphorylase activity may also be deficient in muscle fibers.2–4
Synthesis of glycogen requires glycogen synthase 1 which catalyzes the addition of glucose monomers to the growing glycogen molecule through the formation of α-1,4-glycoside linkages. GSD 0 is caused by mutations in the GYS1 gene that encodes glycogen synthase 1. Deficiency of this enzyme leads to the depletion of glycogen in skeletal and cardiac muscles.
There are no specific medical therapies.
GSD II is an autosomal-recessive disorder caused by a deficiency of lysosomal acid α-glucosidase (Table 29-1,Fig. 29-1). GSD II, more commonly referred to as Pompe disease or acid maltase deficiency, may present in three major forms: a severe infantile form, a juvenile-onset type, and an adult-onset variant.5–28 The incidence of infantile Pompe disease ranges from 1 in 31,000 to 1 in 138,000. The incidence of later onset forms has been purported to be as high as 1 in 53,000, but we think it is far less common in our experience.
Figure 29-1.
Glycolytic pathways. Glycogen metabolism disorders are caused by specific enzyme deficiencies involving each of the pathways illustrated with a Roman numeral. The enzymes corresponding to each numbered glycogen storage disorder are listed in Table 29-1. Diseases I and VI are not included, as these do not involve muscle. (Adapted with permission from Amato AA: sweet success—a treatment for McArdle’s disease. N Engl J Med. 2003;349(26):2481–2482.)

Infantile Pompe disease is characterized by generalized weakness and hypotonia, cardiomegaly, and mild-to-moderate hepatomegaly, with an onset in the first several months of life.10,15–17 Infants often have an enlarged tongue (i.e., macroglossia). The weakness and cardiomyopathy are progressive. Feeding difficulties and ventilatory muscle weakness are common. The disease is invariably fatal by 2 years of age secondary to cardioventilatory failure.
The juvenile-onset acid maltase deficiency usually manifests in the first decade of life.9,13,15,18,28 Motor milestones may be delayed. Weakness is slowly progressive and involves proximal greater than distal muscles in the legs and arms. Children often have hypertrophy of the calf muscles, a waddling gait, and significant lumbar lordosis and demonstrate a Gower maneuver to arise from the floor. Thus, affected children are not uncommonly misdiagnosed with Duchenne or some other form of limb-girdle muscular dystrophy. Rarely, acid maltase deficiency presents with rigidity of the spine.21 Unlike the infantile-onset acid maltase deficiency, cardiomegaly, hepatomegaly, and macroglossia are uncommon. Nevertheless, it is relentlessly progressive, and ventilatory muscles are invariably affected leading to death in the second or third decade of life.
Adult-onset acid maltase deficiency usually manifests in the third or fourth decade (up to the eighth decade, mean 36.5 years).7,9–11,15,21–24,28 Patients develop generalized proximal greater than distal muscle weakness resembling polymyositis or limb-girdle dystrophy. Some patients have a scapuloperoneal distribution of weakness.3 Weakness is occasionally asymmetric and may involve the face or tongue.23 Nearly half of the affected individuals complain of muscle pains, particularly in the thighs. Muscle stretch reflexes may be reduced. Hepatomegaly and cardiomegaly do not typically occur; however, electrocardiographic abnormalities and arrhythmias can be seen. As in the infantile and juvenile forms of the disease, there is a predilection for the involvement of ventilatory muscles. In this regard, 16–33% of patients present with symptoms related to ventilatory insufficiency (e.g., dyspnea, frequent nocturnal arousals, morning headaches, and excessive daytime sleepiness).23,28
Serum CK levels are moderately elevated in infantile-onset, but adults may have normal CK levels. α-Glucosidase activity may be assayed in muscle fibers, fibroblasts, leukocytes, lymphocytes, and urine. The reduction of activity generally correlates with the severity of the myopathy. Infantile-onset disease is associated with a severe deficiency of α-glucosidase activity, while the less severe adult-onset form has residual activity, up to 30% in muscle and 53% in lymphocytes.24 Importantly, false-negative results on leukocyte assay can occur due to contamination with granulocytes or other sources of neutral glucosidase. A dried blood spot analysis of α-glucosidase activity is now the preferred initial screening test.25–28 Of note, activity levels by dried blood spot do not correlate with severity of the myopathy. If the dried blood spot shows reduced enzyme activity, confirmatory testing may be performed by measuring α-glucosidase activity in cultured fibroblasts or muscle tissue or by genetic testing.
Computer tomography (CT) and MRI scans confirm the early and severe involvement of the adductor magnus and semimembranosus in the early stage of the disease and later fatty infiltration of the long head of the biceps femoris, semitendinosus, and the anterior thigh muscles. In advanced phases, selective sparing of sartorius, rectus, femoris, and gracilis muscles, and peripheral portions of the vastus lateralis are also evident.29,30 Skeletal muscle MRI and CT also reveal early involvement of paravertebral and abdominal trunk muscular.31
Motor and sensory nerve conduction studies (NCS) are normal. Electromyography (EMG) reveals increased insertional and spontaneous activity in the form of fibrillation potentials, positive sharp waves, complex repetitive discharges, and even myotonic discharges. In mild forms of the disease, these irritative discharges may be evident only in the paraspinal muscles. Motor unit action potentials (MUAPs) are myopathic in appearance and recruit early.
Electrocardiograms (EKG) may demonstrate nonspecific abnormalities including left axis deviation, short PR interval, large QRS complexes, inverted T waves, ST depression, and persistent sinus tachycardia in both the severe and mild forms of GSD II.23 Wolfe–Parkinson–White syndrome occurs in infantile and adult forms of the disease.32,33 Echocardiograms may show hypertrophic cardiomyopathy. Pulmonary function tests show a restrictive defect with decreased forced vital capacity, reduced maximal inspiratory and expiratory pressures, and early fatigue of the diaphragm.23,28
Biopsies characteristically demonstrate glycogen-filled vacuoles within muscle fibers (Fig. 29-2).15,24,28 These vacuoles are very prominent in the infantile form, but in the childhood and adult forms, these are apparent in only 25–75% of fibers in clinically affected muscles and may be absent in clinically unaffected muscle groups. Muscle biopsy may show only slight, nonspecific abnormalities in late-onset cases. When present, the vacuoles react strongly to periodic acid–Schiff (PAS), are sensitive to diastase, and stain intensely with acid phosphatase, confirming that the vacuoles are secondary lysosomes filled with glycogen. Glycogen can also be found free in the cytoplasm on electron microscopy (EM). Muscle biopsies also reveal necrotic and regenerating muscle fibers, variation in fiber size and fiber splitting. In later stages, muscle fiber atrophy and increased endomysial connective tissue may be present. Occasionally, fiber-type grouping and group atrophy may be evident, owing to motor neuron degeneration. In this regard, glycogens accumulate in anterior horn cells and bulbar nuclei as well as Schwann cells accounting for the superimposed neurogenic findings in some patients.34,35
Figure 29-2.
Pompe disease. Muscle biopsy in a patient with adult-onset acid maltase deficiency reveals one or more vacuoles within many muscle fibers, hematoxylin and eosin (H&E) (A). These vacuoles are filled with glycogen, which stains intensely red on periodic acid–Schiff (PAS) stain (B) and are digested by diastase (C).

Missense, nonsense, and frame-shift mutations have been identified in the α-glucosidase gene, GAA, located on chromosome 17q21–23 in infantile-, childhood-, and adult-onset cases.36,37 Prenatal diagnosis is possible with amniocentesis or chorionic villus sampling.36–38 Acid α-glucosidase is a lysosomal enzyme, which cleaves 1,4 and 1,6 linkages in glycogen, maltose, and isomaltose. Glycogen within lysosomes is degraded to glucose by α-glucosidase, and the deficiency of the enzyme results in glycogen accumulation. There appears to be an inverse correlation between residual acid α-glucosidase activity and the clinical severity. However, there are cases associated with an adult-onset Pompe disease that have very little residual enzyme activity, so the relationship between disease activity and clinical severity is not 100% accurate. Interestingly, there may be variability in phenotype and severity within families.16,39
How acid α-glucosidase leads to muscle fiber dysfunction is not completely understood. The accumulating glycogen that results from the deficiency may displace or replace important cellular organelles. Alternatively, the lysosomes filled with glycogen may rupture, thereby releasing proteases that degrade myofibrils and other important muscle proteins. Muscle catabolism is increased by 31% in Pompe disease compared to normal controls, and mean protein balance is reduced.14 Furthermore, resting energy expenditure in Pompe disease is increased.14 Patients do not exhibit exercise intolerance or myoglobinuria because metabolism of non–membrane-bound glycogen and glucose for energy metabolism is not impaired.
In the past, there were no specific treatments for acid maltase deficiency other than supportive therapy for associated cardioventilatory complications. Low carbohydrate and ketogenic diets are ineffective. A small study reported that 4 out of 16 patients treated with high-protein diet demonstrated improvement in muscle and respiratory function.14
Enzyme replacement therapy (ERT) with intravenous recombinant α-glucosidase enzyme appears to be safe and beneficial in classic infantile Pompe disease though the results of clinical trials in late-onset acid maltase deficiency have demonstrated only a modest benefit in distance traveled in the 6-minute walk test and in forced vital capacity between the α-glucosidase and placebo-treated patients.40–43 The recommended dose of α-glucosidase is 20 mg/kg every 2 weeks. As alluded above, not all patients, including infants with classic Pompe disease, respond to ERT. A poor prognostic factor among infants is cross-reactive immunologic material (CRIM) status; CRIM-negative is strongly correlated with a poor outcome.44,45 Although most CRIM-negative infants initially respond to continuous use of ERT, a resurgence of the natural progression of weakness subsequently ensues. These CRIM-negative infants develop antibodies directed against the infused recombinant α-glucosidase. Presumably, those infants who do not produce even minute amount of α-glucosidase are at increased risk of mounting an antibody response against α-glucosidase, as it is seen to be a foreign protein by the immune system.
GSD III, also known as Cori–Forbes disease, accounts for approximately 25% of GSD (Table 29-1,Fig. 29-1).46–49 GSD III is caused by the deficiency of debranching enzyme. This enzyme has two separate catalytic functions: (1) oligo-1,4-1,4-glucanotransferase activity and (2) α-1,6 glucosidase activity. Both the transferase and the glucosidase activities are vital in breaking down glycogen into glucose, and a deficiency in either or both enzymatic functions lead to myopathy.
There are two principal and two less-common forms of GSD III. In GSD IIIa, debranching enzyme is deficient in both the liver and the muscle. In contrast, enzyme activity is abnormal only in the liver in GSD IIIb, and a myopathy does not occur in this form of the disease. In rare cases, selective loss of only one of the two debranching enzyme activities [glucosidase (type IIIc) or transferase (type IIId)] has also been demonstrated.50
Deficiency of the debranching enzyme in muscle leads to weakness in patients with GSD IIIa. Onset of muscle weakness may be appreciated in infancy or childhood, although it usually does not manifest until the third to fourth decade of life.46–49,51–57 Severe atrophy and weakness of distal extremity muscles, particularly the peroneal and calf muscles, occur in about 50% of patients.48 Tight heal cords are common, and patients may have the tendency to toe walk. This distal involvement can lead to an initial misdiagnosis of motor neuron disease or a peripheral neuropathy. Some patients do, in fact, have a superimposed mild sensorimotor polyneuropathy. Pseudohypertrophy, particularly of the more proximal muscle groups, may be seen.54 Generalized muscle weakness can also occur. In addition, some patients develop progressive ventilatory muscle weakness with or without extremity weakness. Ventilatory failure can evolve fairly rapidly. Less commonly, some patients develop a cardiomyopathy with or without extremity weakness.52,58–61 Finally, rare patients manifest with myalgias, cramps, exercise intolerance, or myoglobinuria.48,53,55,56,62
Deficiency of debranching enzyme can be demonstrated with biochemical assay of muscle, fibroblasts, or lymphocytes. Serum CK levels are elevated 2–20 times normal. Exercise forearm testing reveals normal increase in serum ammonia but not in lactate levels. EMG demonstrates abnormalities similar to that described with acid maltase deficiency. Pulmonary function tests show reduced forced vital capacity in patients with ventilatory muscle involvement. Echocardiogram reveals findings suggestive of hypertrophic obstructive cardiomyopathy in most patients with GSD IIIa, while conduction defects and arrhythmias are apparent on EKG.46,58,61,62
Muscle biopsies demonstrate a vacuolar myopathy with abnormal accumulation of glycogen in the subsarcolemmal and intermyofibrillar regions of muscle fibers.51,53,54 These vacuoles stain intensely with PAS and are digested by diastase. In contrast to acid maltase deficiency, these vacuoles do not stain with acid phosphatase, suggesting that glycogen does not primarily accumulate in lysosomes. On EM, free pools of glycogen are apparent. Some glycogen appears in lysosomes, but not to the same extent as seen in acid maltase deficiency. Autopsy studies of the heart have revealed fibrosis, moderate to severe vacuolization of cardiac myocytes, mild-to-severe glycogen accumulation in the atrioventricular (AV), and glycogen accumulation in smooth muscle cells of intramyocardial arteries associated with smooth muscle hyperplasia and profoundly thickened vascular walls.63 Abnormal glycogen accumulation can also be found in skin and peripheral nerves.64–66
The mutations in the debranching enzyme gene, AGL, on chromosome 1p21 cause both GSD IIIa and GSD IIIb.67–70 Prenatal diagnosis is possible.71 The AGL gene is composed of 35 exons spanning 85 kb of genomic DNA. Alternative splicing and differential RNA transcription result in at least six distinct isoforms and underline the differential expression of the debranching enzyme.72 Tissue-specific expression of different isoforms results from the presence of at least two promoter regions. Of note, mutations within exon 3 mutations appear to be specific for GSD IIIb.70
Deficiency of the enzyme leads to the accumulation of glycogen in muscle; the exact mechanism of muscle weakness is not known. Similar amounts of glycogen accumulation in muscle can be demonstrated in patients who do not manifest weakness. Accumulation of glycogen in peripheral nerves may account for some degree of weakness and atrophy, particularly of the distal muscles.
Frequent low-carbohydrate meals and maintaining a high-protein intake may prevent fasting hypoglycemia. High-protein nocturnal intragastric feedings led to apparent improvement in exercise tolerance, muscle strength and mass, electromyographic findings, and growth in one patient,57 but this observation has not been subsequently confirmed. Supportive therapy is required for patients with congestive heart failure. Liver transplantation has been done on patients with cirrhosis and hepatocellular carcinoma.72 However, debranching enzyme activity has remained absent in leukocytes after transplantation and is not likely to normalize in muscle.
GSD IV is rare and caused by the deficiency of the enzyme that helps make the branched glycogen molecule (Table 29-1,Fig. 29-1).73–89,90 There are several forms of branching enzyme deficiency. The classic and most common type of GSD IV, also known as Andersen disease, presents in infancy as progressive liver dysfunction with hepatomegaly, splenomegaly, and failure to thrive. Muscular weakness, atrophy, hypotonia, hyporeflexia, and contractures may occur but are overshadowed by the liver disease.79,84 Most children succumb to severe liver failure by 5 years of age. There is also a benign hepatic form of GSD IV in which the liver disease does not progress.70,88 Some patients with GSD IV manifests primarily with muscle weakness, atrophy, and cardiomyopathy in childhood or adult life.70,74,82,83 Either proximal or distal muscle groups can be preferentially affected. In addition, a fatal infantile form is associated with congenital onset of severe weakness.84–87 Finally, there is a variant of branching enzyme deficiency, known as polyglucosan body neuropathy, which usually presents in adults as progressive upper and lower motor neuron loss, sensory nerve involvement, cerebellar ataxia, neurogenic bladder, and dementia.75,77,89,90 Occasionally, polyglucosan body neuropathy manifests in children.78 There is a predilection for polyglucosan body neuropathy in the Ashkenazi population.
Depending on the subtype of GSD IV, deficiency of branching enzyme may be demonstrated in muscle, peripheral nerve, fibroblasts, or leukocytes.74,75,80,89,90 In patients with primary neuromuscular involvement, the deficiency may be noted only in muscle.74 Branching enzyme activity can be normal in the muscle in patients with adult polyglucosan body neuropathy.75,77 The serum CK may be normal or slightly elevated. EMG reveals myopathic features and muscle membrane instability similar to that observed with GSDs II and III. In patients with polyglucosan body neuropathy, an axonal sensorimotor neuropathy is apparent, while the EMG abnormalities reflect a superimposed polyradiculopathy. EKG can demonstrate progressive conduction defects leading to complete AV block.82 Echocardiogram may reveal a dilated cardiomyopathy.82
Routine light microscopy and EM reveals deposition of varying amounts of finely granular and filamentous polysaccharide (polyglucosan bodies) in the CNS, peripheral nerves (axons and Schwann cells), skin, liver, and cardiac and skeletal muscles.74,77,78,81–83 These polyglucosan bodies are PAS-positive and diastase resistant, suggesting the accumulation of polysaccharides other than glycogen (Fig. 29-3). They are not specific for this disorder and can be seen occasionally in nerve biopsies from patients with other diseases. This polysaccharide resembles amylopectin in that it has longer than normal peripheral chains and few branch points.
Figure 29-3.
Type IV glycogenoses/Anderson disease. Muscle biopsy reveals vacuolated fibers on H&E (A) and modified Gomori trichrome (B) that appear to contain amorphous debri, which are periodic acid–Schiff (PAS)-positive (C), and diastase resistant (D) suggestive of a filamentous polysaccharide that is not glycogen (i.e., polyglucosan). Semithin plastic sections counterstained with PAS demonstrate increased polysaccharide deposition within muscle fibers (E).

Autopsy studies have demonstrated abnormal polysaccharide material in the liver, heart, skeletal muscle, and in neurons of the brain and the spinal cord. The abnormal polysaccharide material is more abundant in the motor neurons than in other nerve cells and affects all motor neurons of the brainstem and spinal cord.91
The disease is inherited in an autosomal-recessive manner. Deletions, nonsense, and missense mutations within the glycogen branching enzyme (GBE1) gene on chromosome 3p12 have been identified in the severe hepatic, benign hepatic, and the neuromuscular forms of GSD IV, including adult polyglucosan body disease.73,76,89,90 There are phenotypic variability and differential expression of branching enzyme activity. The mechanism by which the abnormal accumulation of polysaccharide results in muscle damage is not known.
Liver transplantation has been performed in some children with GSD IV with beneficial results.92–94 Apparently, systemic microchimerism occurs after liver allotransplantation and can ameliorate pancellular enzyme deficiencies in this disease. Most of the patients became free of liver, neuromuscular, and cardiac dysfunction, with reduced polysaccharide accumulation in these tissues on long-term follow-up (mean 42 months). However, at least one child died from cardiomyopathy due to massive deposition of polysaccharide in the heart 2½ years after transplantation.92 No other medical therapies have been demonstrated to be effective.
Glycogenosis type V (myophosphorylase deficiency), more commonly known as McArdle disease, is the most common neuromuscular disorder of carbohydrate metabolism. McArdle disease is an autosomal-recessive disorder that usually presents with exercise intolerance in childhood or young adults (Table 29-1,Fig. 29-1).95–98 Patients complain of exertional muscle pain and cramps induced by brief, but very intense, activities (e.g., weight lifting and sprinting), but these can also occur following prolonged low-intensity exercises (e.g., swimming and jogging). If individuals who are affected ignore these symptoms and continue to exercise at a high level, the muscle pain and cramping can become quite intense and electrically silent contractures may develop. Some patients present with fatigue following exercise without associated cramps or muscle pain. Many patients note a second-wind phenomenon, in which after the onset of mild exertional myalgias or cramps (usually after 10 minutes of exercise), the muscle pain and sense of intolerance may dissipate. Subsequently, the individual may continue with the exercise at the previous or a slightly reduced level.98 The second-wind phenomenon is the result of mobilization and use of blood-borne glucose.
Not everyone develops myoglobinuria and only about 50% of attacks of myoglobinuria are exertionally related. Myoglobinuria may not occur until the second or third decade, although it has developed in the 1st decade of life.96,99 As many as 10% of attacks may be accompanied by acute renal failure, but this may be high as there probably are subclinical bouts of myoglobinuria that go unreported.96
Most patients have normal motor examinations between attacks of muscle cramping. However, fixed proximal weakness develops in as many as one-third of patients, perhaps as a result of recurrent bouts of rhabdomyolysis. Rare patients present with progressive proximal muscle atrophy and weakness in late-adult life rather than exercise intolerance.100 Weakness may involve the arms more than the legs and can be asymmetric. Finally, a few cases have been reported with congenital weakness, some of which were rapidly progressive, leading to ventilatory failure within the first year of life.101,102
Serum CK levels are invariably elevated even while patients are asymptomatic. The exercise forearm test reveals a normal rise in serum ammonia but no significant rise in lactic acid.103 EMG is usually normal in patients with McArdle disease.
Muscle biopsies demonstrate variability in fiber size, scattered necrotic and regenerating fibers, excessive accumulation of glycogen in the subsarcolemmal and intermyofibrillar areas, and absent myophosphorylase staining (see Fig. 3-6).104,105 Biochemical assay for myophosphorylase reveals absent or significantly reduced activity.
This disorder is inherited in an autosomal-recessive fashion and is caused by mutations in the PYGM gene that encodes myophosphorylase.102,106,107 This enzyme initiates glycogen breakdown by phosphorylytically lysing α-1,4 glucosyl residues from the outer branches of glycogen, generating glucose-1-phosphate. Mutations result in little detectable protein or enzyme activity. Interestingly, the mutations associated with some of the rare cases of fatal infantile myopathy are the same as evident in the more common clinical presentation of McArdle disease.102 A pseudodominant pattern of inheritance has been reported and felt to be secondary to heterozygotes who have low levels of residual myophosphorylase.108 Another mechanism is the mating of a homozygote (or compound heterozygote) with a heterozygote.108
Although exercise intolerance and contractures had been postulated to be due to the inability to generate enough ATP, studies have demonstrated that ATP is not depleted during exercise in McArdle disease, Tarui disease, or in the other disorders of glycogenolysis and glycolysis.109 Exercise is associated with (1) an increase in adenosine diphosphate (ADP); (2) intracellular pH that does not acidify in response to exercise; (3) inorganic phosphate levels in muscle, which are 50% lower than normal muscle tissue; and (4) intracellular calcium concentrations at the onset of contracture, which is more than 10-fold greater than that found in normal control muscle ischemically exercised.110 Perhaps the combination of increased intracellular ADP, reduced inorganic phosphate, and lack of acidification with impaired glycolysis increase sensitivity of the muscle fiber contractile apparatus to intracellular calcium. Further, the increased intracellular ADP may inhibit ADP dissociation from actin-myosin cross-bridges, thereby increasing the time spent in contraction.
In addition, patients with McArdle disease have reduced concentrations of the sodium–potassium ATPases pump, higher exercise-induced serum potassium concentrations, and a greater increase in heart rate during exercise.111 Decreased sodium–potassium ATPases may lead to an exercise-induced increase in extracellular potassium because of impaired reuptake of potassium released during muscle contraction. Further, exercise intolerance leads to reduced physical activity, which may result in downregulation of the pump, and the increased ADP may decrease the transport rate of the remaining pumps. The increased concentration of extracellular potassium partially depolarizes the muscle membrane, thereby inactivating sodium channels and reducing membrane excitability.111 Patients with McArdle disease also develop exaggerated tachycardia with exertion that can limit the exercise capacity of individuals who are affected.111 It is not known why sodium–potassium pump concentrations in skeletal muscle in patients with McArdle disease are reduced. Reduced physical activity may downregulate the number of sodium–potassium ATPase pumps. Alternatively, myophosphorylase deficiency may reduce the pump concentration by disrupting the normal coupling of muscle glycogenolysis and pump activity.111
A single-blind, placebo-controlled, crossover study of oral sucrose (75 g) in 12 patients with McArdle disease demonstrated marked improvement in exercise tolerance, supported by the subjects’ reduced perceived exertion levels and their diminished maximum heart rates.98 The limitation of oral sucrose loading is that the beneficial effect is short-lived. Repeated dosing may lead to weight gain, which in and of itself can reduce exercise tolerance. Furthermore, it can cause inhibition of fatty acid use, which also is an important fuel source with prolonged physical activity. Sucrose loading will also not be helpful in situations of unexpected exertional activity and prolonged physical activity or with static exercise (e.g., weight lifting).
A high-protein diet might help, but supplementing the diet with branched-chain amino acid supplementation can actually lower exercise capacity.112 Creatine monohydrate has been studied but found to be of no significant benefit.113 Surplus calories may lead to weight gain and subsequent decline in cardiovascular fitness. Some small studies have suggested that vitamin B6 supplementation (50 mg/day) can reduce exercise intolerance and enhance performance.106 A recent Cochrane review of clinical trials reported that there was low quality evidence of improvement in some parameters with creatine, oral sucrose, ramipril, and a carbohydrate rich diet, although none indicated significant, clinical benefit.97
We instruct patients to avoid intense isometric exercises (e.g., weight lifting) and maximum aerobic exercises (e.g., sprinting). However, mild-to-moderate aerobic conditioning may be beneficial, as poor cardiovascular fitness results in a diminished delivery of blood-borne substrates necessary for muscle oxidative metabolism.98,112 Patients should be instructed on how to moderate their physical activity in order to obtain a “second-wind” response. Any bout of moderate exercise should be preceded by 5–15 minutes of low-level warm-up activity to promote the transition to the second “wind.”112
PFK deficiency or Tarui disease is an autosomal-recessive disease, caused by a deficiency in PFK in muscle and erythrocytes (Table 29-1,Fig. 29-1). PFK deficiency is much less common than McArdle disease. The clinical features are very similar to McArdle disease with respect to exercise intolerance, muscle pain, contractures, and relief of discomfort by rest. However, PFK deficiency is not associated with the warm-up phenomena, and there is a lower incidence of myoglobinuria.114,115 In addition, some patients develop jaundice (due to mild hemolysis) and gouty arthritis due to PFK deficiency in erythrocytes.
The clinical phenotype can vary, and there are less common presentations. Some individuals who are affected manifest with hemolytic anemia without a myopathy. Others present later in adulthood with fixed weakness, which may predominantly affect the proximal or occasionally the scapuloperoneal muscles.116,117 They may have had only mild exercise intolerance in their younger years but never have a history of cramps or myoglobinuria. In addition, PFK deficiency can present in infancy with severe generalized weakness and cardiomyopathy. Contractures, cortical blindness, and corneal opacifications are evident in some infants, but hemolytic anemia does not occur. Severely affected children may die from cardioventilatory failure in infancy or early childhood.
Serum CK is usually elevated, and mild anemia and increased reticulocyte count are often noted.118 Exercise forearm testing reveals a normal increase in ammonia production but a blunted increase in lactic acid. EMG is usually normal.
Muscle biopsies demonstrate vacuoles and an abnormal accumulation of glycogen.116 In addition, there is also an abnormal accumulation of polysaccharide, which stains intensely with PAS but is diastase resistant, especially in older patients. Muscle biopsies may reveal only nonspecific myopathic features without evidence of abnormal glycogen accumulation in the infantile form of disease. Definitive diagnosis of Tarui disease can be made by biochemical and histochemical analyses of muscle tissue, which reveal the deficiency of PFK activity and staining.
PFK catalyzes the ATPase-dependent conversion of fructose 6-phosphate to fructose 1,6-diphosphate. Human PFK comprises three distinct isoenzyme subunits (M—muscle, L—liver, and P—platelet). Skeletal muscles contain only the M isoform, while erythrocytes contain a hybrid of M and L subunits. The gene responsible for the M isoform, PFK-M, was initially mapped to 1q32 but was subsequently reassigned to 12q13. The symptoms reflect inactivation of PFK in muscle and partial inactivation in red blood cells. Different molecular defects may explain the different clinical presentations; however, the biochemical and molecular basis for clinical heterogeneity remains unclear.
As in McArdle disease, there is ADP accumulation in exercised muscle, but whether or not there is also reduction in sodium–potassium pumps in Tarui disease is not known. The normal coupling of muscle glycogenolysis and sodium–potassium pump activity may be disrupted by increased ADP or reduction in pump concentration, as we described in the section on McArdle disease.
Unlike in McArdle disease, glucose or fructose administration prior to activity does not help; rather it may be deleterious. Patients with PFK deficiency rely on free fatty acids as a fuel substrate during exercise. Therefore, they experience more exercise intolerance, if given a glucose infusion or they consume high-carbohydrate meals, because glucose reduces the blood levels of free fatty acids.119 This is just the opposite of the second-wind phenomena and is sometime called the out-of-wind phenomena. An aerobic conditioning program similar to those given to patients with McArdle deficiency may improve exercise tolerance.
Muscle PBK or phosphorylase kinase (PHK) deficiency was formerly designated GSD VIII but now is more commonly referred to as GSD IX. PHK is a multimeric enzyme composed of four subunits. As will be discussed below, mutations involving PHKA1, encoding subunit α, cause the rare X-linked GSD IXa that is only associated with muscle involvement. Mutations in PHKB, encoding subunit β, cause the more common autosomal-recessive GSD IXb that involves both the liver and muscle. Mutations in other subunits are not associated with muscle involvement.
PHK deficiency is associated with heterogeneous clinical manifestations.120–123 It most commonly manifests as exercise intolerance with cramps and myoglobinuria (Table 29-1,Fig. 29-1). However, PHK deficiency can occasionally present in infancy or childhood with mild weakness and a delay in motor milestones. Rarely, a fatal cardiomyopathy can occur in infancy. Approximately 50% of patients develop proximal or distal weakness in adulthood.
Serum CK may be normal or mildly elevated. The exercise forearm test may be normal or abnormal. EMG is usually normal. Diagnosis is based on clinical findings, assay of PHK activity in erythrocytes, or liver or muscle tissues (depending upon presentation) and confirmatory findings on molecular genetic testing.
Muscle biopsy may be normal or may demonstrate variability in fiber size, scattered necrotic fibers, and slight subsarcolemmal accumulation of glycogen.115 Biochemical analysis reveals decreased PHK activity.
PHK catalyzes the conversion of inactive myophosphorylase to the active form and converts active glycogen synthetase to an inactive form. As mentioned above, PHK is a multimeric enzyme composed of four different subunits. Mutations in PHKA1, encoding subunit α, cause the less-common X-linked GSD IXa that is associated with muscle involvement only, while mutations in PHKB, encoding subunit β, cause autosomal-recessive GSD IXb that involves both liver and muscle.124
There is no specific medical therapy. Patients should be instructed on a mild-to-moderate exercise program and to avoid vigorous activity.
Phosphoglycerate mutatase (PGAM) deficiency presents in childhood or early adult life as exercise intolerance, cramps, and recurrent myoglobinuria (Table 29-1,Fig. 29-1).125,126
Serum CK is mildly elevated. The exercise forearm test is abnormal. EMG is normal.
Muscle biopsies reveal increased glycogen by PAS staining and on EM. Rarely, these are tubular aggregates in type 2B fibers. Biochemical assay demonstrates normal or only mildly elevated glycogen content and markedly diminished activity of PGAM (<10% of normal).
Type X glycogenosis is an autosomal-recessive disorder caused by mutations in the PGAM-M gene encoded on chromosome 7p13–p12.3. PGAM catalyzes the interconversion of 2- and 3-phosphoglycerate. There are two subunits for PGAM: a muscle-specific subunit (PGAMM) and a non–muscle-specific or brain subunit (PGAMB). Mature muscle contains the homodimer MM form of PGAM, which has diminished enzymatic activity in type X glycogenosis.
There is no definitive medical therapy. Dantrolene improved symptoms in one patient with severe cramps and tubular aggregates on muscle biopsy.125 Nevertheless, dantrolene is not recommended as routine therapy. Patients should be instructed on avoiding strenuous activity and placed on a mild-to-moderate aerobic exercise program.
This rare autosomal-recessive disorder manifests as exercise intolerance, cramping, and recurrent myoglobinuria (Table 29-1,Fig. 29-1).127 Muscle strength is normal. Patients may also develop a generalized, scaly, erythematous rash, particularly in the summer. Pregnancies may be complicated by uterine stiffness in early stages of delivery and often requires cesarean section. This complication has not been associated with other glycogenoses. Chronic renal failure can develop secondary to recurrent myoglobinuria.
Serum CK level is elevated. Serum LDH, which is usually markedly elevated during attacks of rhabdomyolysis, is normal in patients with LDH deficiency. A reduction in the LDH-M isoform (<5% of normal) in muscle and blood can be demonstrated on electrophoretic studies. On exercise forearm testing, lactate does not rise; however, there is a normal increase in pyruvate levels, because the enzymatic defect lies distal to the formation of pyruvate in the metabolic pathway. EMG is typically unremarkable.
Muscle biopsies can appear normal, but biochemical assay reveals reduced activity of LDH.
There are five distinct LDH isoenzymes, each comprising tetramers composed of combinations of two different subunits, M and H. Thus far, only mutations involving the muscle M subunits encoded by the LDH gene, LDHA, on chromosome 11p15.4 have been associated with muscle disease.127
There is no specific medical therapy. Obstetricians need to be made aware of potential complications of labor in affected pregnant females.
This rare disorder has been reported in two young children who presented with rhabdomyolysis, exercise intolerance, and weakness, and episodes of hemolytic anemia- some following febrile illnesses (Table 29-1,Fig. 29-1).128,129
Serum CK was elevated in this singular case.
Muscle biopsy appeared normal on routine light microscopy, but EM revealed accumulation of lipid. Biochemical analysis revealed markedly reduced aldolase activity.
Mutations in the aldolase gene located on chromosome 16q22–24 were reported.128 Aldolase catalyzes the conversion of fructose 1,6-phosphate to dihydroxyacetone phosphate and glyceraldehyde 3-phosphate. The enzyme is expressed in red blood cells which probably accounts for the episodes of hemolytic anemia.
There are no specific medical therapies.
This rare myopathy was reported in a 46-year-old man with exercise intolerance and myalgias (Table 29-1,Fig. 29-1).130
The serum CK levels were episodically elevated. No rise in lactic acid was noted on an exercise forearm test.
Muscle biopsy revealed abnormal accumulation of glycogen in the sarcoplasm. Selective β-enolase deficiency was demonstrated with immunohistochemistry and immunoblotting.
Heterozygous mutations were identified within the β-enolase gene. β-Enolase catalyzes the step interconverting 2-phosphoglycerate and phosphoenolpyruvate.
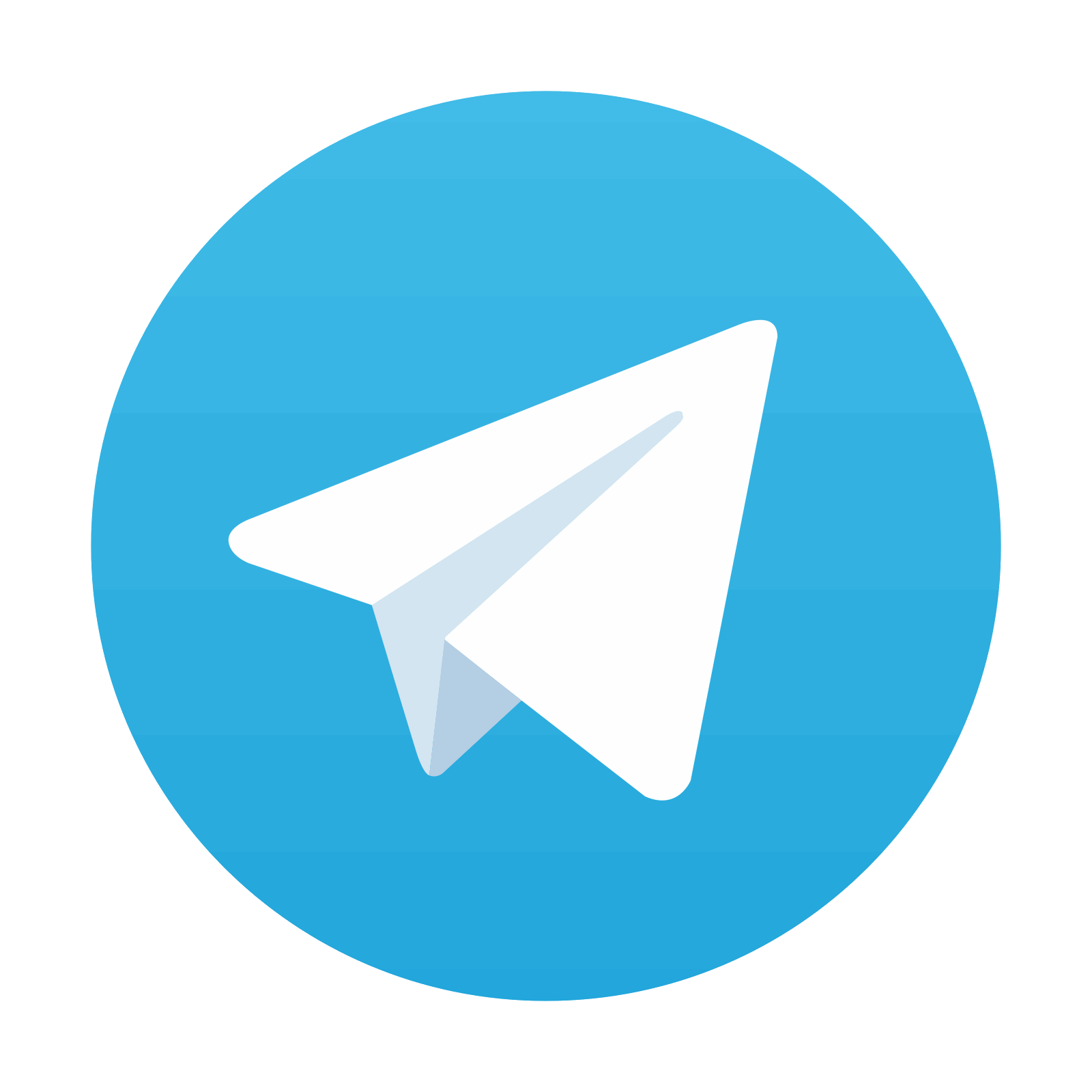
Stay updated, free articles. Join our Telegram channel

Full access? Get Clinical Tree
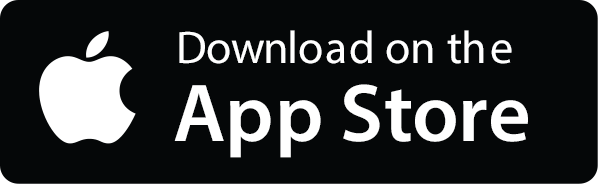
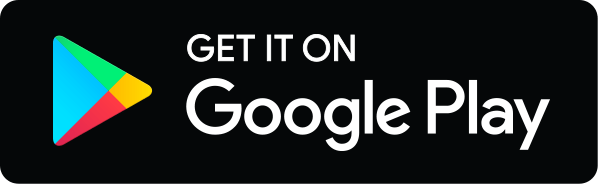