The central nervous system (CNS) has evolved as an immune-privileged site to protect its vital functions from damaging immune-mediated inflammation. There must be a CNS-adapted system of surveillance that continuously evaluates local changes in the nervous system and communicates to the peripheral immune system during an injury or a disease. Recent advances leading to a better understanding of the CNS disease processes has placed microglia, the CNS-based resident macrophages, at center stage in this system of active surveillance. Evidence points to microglia cells contributing to the immunosuppressive environment of gliomas and actually promoting tumor growth. Microglia accumulation exists in almost every CNS disease process, including CNS tumors. This article discusses the role of microglia in CNS immunity and highlights key advances made in glioma immunology.
The central nervous system (CNS) has evolved as an immune-privileged site to protect its vital functions from damaging immune-mediated inflammation. With the protective shield of the blood-brain barrier and lack of lymphatic system, the CNS strictly regulates the entry of most peripheral immune cells, soluble factors, and plasma proteins in homeostasis. However, during a CNS injury or disease such as multiple sclerosis (MS), T-cell and peripheral antigen-presenting–cell (APC) infiltration are involved in the observed pathogenesis. Thus, there must be a CNS-adapted system of surveillance that continuously evaluates local changes in the nervous system and communicates to the peripheral immune system during an injury or a disease. Recent advances leading to a better understanding of the CNS disease processes has placed microglia, the CNS-based resident macrophages, at center stage in this system of active surveillance. Microglia are tightly regulated by their local environment, including neuron-microglia interactions during homeostasis, and they remain quiescent. In the steady-state CNS, microglia cells express the macrophage marker CD11b and exhibit a resting phenotype characterized by a low-level expression of major histocompatibility complex (MHC) class I and II and co-stimulatory molecules like CD86 and CD40. Conversely, in the event of an insult or injury, microglia cells increase mobility, phagocytosis, and proliferation, and they contribute to resulting immune responses and inflammation by releasing various reactive oxygen intermediates, cytokines, and chemokines. In comparison to macrophage responses in the peripheral tissues, microglia responses on activation are restricted, spatially and temporally, to preserve an immunologically silent CNS environment. Microglia as APCs also contribute to activating T-cell responses by upregulating MHC and co-stimulatory molecules, generating specific CD4 and CD8 memory responses. Therefore, microglia are essential components of the innate and adaptive aspects of immune responses in the CNS, similar to macrophages in the peripheral tissues.
Considering these broad immune functions, it is not surprising to see microglia accumulation in almost every CNS disease process; examples include CNS tumors, particularly malignant gliomas, which account for nearly half of primary CNS neoplasm and are known to accumulate a large number of microglia and a small population of lymphocytes. Initial observation of this high percentage of microglia in gliomas led many to deduce their role in antitumor activity and in cell death (necrosis) associated with many gliomas like glioblastoma multiforme (GBM). However, in recent years, the accumulating evidence strongly suggests that microglia cells contribute to the immunosuppressive environment of gliomas and can actually promote tumor growth. This article discusses the role of microglia in CNS immunity and highlights key advances made in glioma immunology.
Origin of microglia
Microglia constitute 5% to 20% of total glial cell population in the CNS, making them as abundant as neurons. They were first recognized by Nissl, who named them “Staebchenzellen” or rod cells for their rod-shaped nuclei and considered them to be reactive neuroglia suggesting a capacity for migration and phagocytosis. In 1913, Santiago Ramon y Cajal described microglia as part of 3 elements of the CNS. It was del Rio Hortega, after his studies on young animals, who established microglia as distinct from other non-neuronal cell types, astrocytes and oligodendrocytes. Hortega described microglia’s origin from mononuclear cells of the circulating blood and believed that these cells have the ability to transform from resting ramified form into amoeboid phagocytic macrophages. These conclusions were based on Hortega’s observations from stab wounds made in the mature brains of various animal species. In 1925, Wilder Penfield further usedthe special silver-staining method utilized by Hortega to provide the first detailed descriptions of microglia in glioma tissue. After a long debate over the role and origin of microglia in the CNS, they have been established as a phenotypically, developmentally, and functionally distinct population of glial cells that are of myelomonocytic origin. The microglia precursor cells of monocyte-macrophage lineage are derived from mesodermal hematopoietic cells, which in mammals originate from the yolk sac. These circulating precursor cells invade the developing brain during perinatal stages and transform into microglia cells that express several macrophage-specific markers, including Toll-like receptors (TLRs), the integrin CD11b, and the glycoprotein of unknown function, F4/80, but they have lower levels of the leukocyte common antigen, CD45, when compared with macrophages. These findings together with the phagocytic activity of microglia strongly suggest that microglia are closely related to peripheral monocytes.
Recent studies have classified microglia into 3 types according to their morphologic appearance: resting ramified microglia, activated reactive microglia, and amoeboid phagocytic microglia. Furthermore, consistent with the immunologically silent nature of the CNS, the invading amoeboid microglia in perinatal life transform into ramified resting microglia during postnatal life, which represent a fairly permanent population with slower turnover when compared with macrophages of other tissues. According to studies done by Lawson and colleagues, the resting ramified microglia are less numerous in white matter than in gray matter, and they adapt the morphology of their cell bodies, processes, and expression of cell surface markers to their microenvironment. The microglia remain in the resting state until a stimulus from an injury, infection, or other neurodegenerative process activates them to transform back into activated amoeboid phagocytic cells.
Microglia as a system of active surveillance
In the steady state, the fairly quiescent-looking microglia cells undergo continuous pinocytosis to sample the surrounding microenvironment, as a way of conducting routine surveillance of the CNS. Neuron-to-microglia communication also plays a key role in the surveillance, and it shapes the quiescent and reactive states of microglia. In vitro studies have provided evidence that electrically active neurons inhibit T H 1 cytokine interferon (IFN)-γ-induced expression of major histocompatibility complex (MHC) class II molecules on astrocytes and microglia, whereas some neurotransmitter molecules like substance P enhance the pro-inflammatory phenotype of microglia. These opposing actions of various neurotransmitters suggest the existence of a complex set of interactions between local inhibitory and stimulatory signals in shaping microglia responses. In vivo studies conducted by Hoek and colleagues have led to the characterization of a previously identified membrane-bound glycoprotein termed OX2 or CD200 expressed on neurons as a key regulator of microglia. In OX2-deficient mice, microglia exhibit an activated phenotype with enhanced CD45 and complement type-3 receptor (CR3) along with less ramified morphology. In addition, OX2- deficient mice show an accelerated reactive response to an injury. Thus, neurons deliver inhibitory signals to microglia through OX2 receptor signaling pathway. These findings in combination strongly support the idea of microenvironment regulation of microglia function.
Moreover, the plasticity of microglia in function and morphology directly correlates with its interactions with the microenvironment. Granulocyte-macrophage colony-stimulating factor (GM-CSF) and M-CSF are known to play a crucial role in the terminal differentiation of tissue macrophages. In Alzheimer disease and MS, it has been shown that levels of GM-CSF are elevated in addition to upregulation in M-CSF receptor. With in vitro studies, Fischer and Reichmann have demonstrated that the incubation of purified microglia with GM-CSF increases microglia cell size, and it generates a heterogeneous population that contains cells resembling other tissue macrophages and microglia cells expressing CD11c and MHC class II molecules. These studies indicate that microglia cells are poised to use their range of plasticity in response to their microenvironment changes.
Microglia as a system of active surveillance
In the steady state, the fairly quiescent-looking microglia cells undergo continuous pinocytosis to sample the surrounding microenvironment, as a way of conducting routine surveillance of the CNS. Neuron-to-microglia communication also plays a key role in the surveillance, and it shapes the quiescent and reactive states of microglia. In vitro studies have provided evidence that electrically active neurons inhibit T H 1 cytokine interferon (IFN)-γ-induced expression of major histocompatibility complex (MHC) class II molecules on astrocytes and microglia, whereas some neurotransmitter molecules like substance P enhance the pro-inflammatory phenotype of microglia. These opposing actions of various neurotransmitters suggest the existence of a complex set of interactions between local inhibitory and stimulatory signals in shaping microglia responses. In vivo studies conducted by Hoek and colleagues have led to the characterization of a previously identified membrane-bound glycoprotein termed OX2 or CD200 expressed on neurons as a key regulator of microglia. In OX2-deficient mice, microglia exhibit an activated phenotype with enhanced CD45 and complement type-3 receptor (CR3) along with less ramified morphology. In addition, OX2- deficient mice show an accelerated reactive response to an injury. Thus, neurons deliver inhibitory signals to microglia through OX2 receptor signaling pathway. These findings in combination strongly support the idea of microenvironment regulation of microglia function.
Moreover, the plasticity of microglia in function and morphology directly correlates with its interactions with the microenvironment. Granulocyte-macrophage colony-stimulating factor (GM-CSF) and M-CSF are known to play a crucial role in the terminal differentiation of tissue macrophages. In Alzheimer disease and MS, it has been shown that levels of GM-CSF are elevated in addition to upregulation in M-CSF receptor. With in vitro studies, Fischer and Reichmann have demonstrated that the incubation of purified microglia with GM-CSF increases microglia cell size, and it generates a heterogeneous population that contains cells resembling other tissue macrophages and microglia cells expressing CD11c and MHC class II molecules. These studies indicate that microglia cells are poised to use their range of plasticity in response to their microenvironment changes.
Microglia as mediators of inflammation
One key similarity of microglia and peripheral macrophages is the ability to contribute significantly to innate and adaptive immune responses. The resting microglia cells are activated by various stimuli, such as lipopolysaccharide (LPS), β amyloid, IFN-γ, thrombin, and some proinflammatory cytokines, involved in infection, neurodegenerative diseases, and CNS injury. Upon stimulation, the resting ramified microglia undergo a series of morphologic and functional changes to mobilize the cellular and molecular defense of the CNS. For example, it has been demonstrated that microglia express TLRs that interact with bacterial cell wall components to initiate innate response such as production of cytokines, chemokines, and nitric oxide. The molecules released by microglia in response to stimuli include (1) proinflammatory cytokines interleukin (IL)-1, IL-6, and tumor necrosis factor (TNF)-α ; and (2) monocyte chemoattractant protein-1 (MCP-1), macrophage inflammatory protein 1 (MIP-1), and regulated on activation normal T cell expressed and secreted (RANTES) ; and (3) chemokines involved in lymphocyte recruitment. This implicates microglia as a critical first line of defense because the adaptive immune cells typically take longer to respond to pathogens present in the CNS. Once the microenvironment of the CNS has become activated, the local cells produce proinflammatory cytokines and chemokines and upregulate immunomodulatory surface markers to contribute to local inflammatory response in addition to decreasing the stringency of the blood brain barrier. This allows the entry of soluble factors and immune effector cells from the periphery, such as macrophages, natural killer cells, and lymphocytes. Early activation of microglia before peripheral cell infiltration is supported by bone marrow chimera studies demonstrating that microglia activation precedes the entry of peripheral macrophages. Microglia also exhibit phagocytic and cytotoxic functions during CNS infection and injury. On microglia activation, there is upregulation of opsonic receptors, including complement receptors (CR1, CR3, CR4) and FcγR (I,II,III), which enhances phagocytosis by binding to complement components and immunoglobulin fragments respectively. Microglia are known to function as transitory phagocytes during CNS ontogeny to clear apoptotic neuronal cell bodies, and in experimental autoimmune encephalitis (EAE), the animal model of MS, T-cell debris is phagocytosed by microglia. Phagocytosis by microglia directly induces the production of reactive radicals to degrade cellular debris. Moreover, microglia secrete superoxide radicals and nitric oxide into their microenvironment in response to pathogens and cytokine stimulation.
Antigen presentation is a critical event involved in the generation of T-cell responses against the pathogen, as part of pathogen-specific adaptive immune response. The antigen presentation requires interaction between the T-cell receptor and the processed antigen peptide presented on MHC molecules on the surface of APCs. MHC class I and MHC class II molecules stimulate CD8 cytotoxic cells and CD4 T-helper cells, respectively. Additional key interactions between co-stimulatory molecules, such as B7-1 (CD80), B7-2 (CD86), and CD40, expressed on the surface of APC and specific counter-receptors on T cells are required for optimal T-cell–APC adhesion and activation. Within the CNS parenchyma under steady-state conditions, MHC class I and II expression is generally minimal or absent, and when present, it is restricted to microglia in low levels. According to the studies done by Ford and colleagues in normal rodent brains, microglia behave as poor APCs under resting conditions. However, in inflammatory and neurodegenerative conditions, microglia readily upregulate MHC expression along with co-stimulatory molecules. In particular, the interaction of CD80 and CD86 molecules on microglia with CD28 expressed on T cells is required for inducing T-cell cytokine secretion, growth, and survival. Additionally, microglia CD40 interaction with CD40L on T cells enhances expression of MHC class II, nitric synthase and CD80/CD86 molecules on microglia, which in turn further promote T-cell activation. In EAE, the animal model of MS, both microglia and blood-derived macrophages have been shown to express MHC class II and co-stimulatory molecules. Additionally, in vivo and in vitro studies have demonstrated that IFN-γ, a cytokine secreted by pre-activated CD4 T cells and NK cells, induces and maintains MHC class II and adhesion/co-stimulatory molecule expression on microglia to maintain stimulation of T cells.
In addition to initiating innate and adaptive immune responses, microglia contribute to downregulating inflammatory responses. In the absence of sufficient co-stimulatory molecules, the interaction of Fas ligand (FasL) on APCs with the Fas receptor on the T cell will lead to activation-induced T cell apoptosis. In vitro and in vivo studies of EAE have detected FasL expression on microglia. Cytotoxic molecules like nitric oxide produced by microglia can also contribute to the death of immune effector response. Additionally, microglia can express Fas molecules on their surface that can interact with FasL-expressing cells, leading to their own apoptosis. These studies indicate that microglia activity in a neuropathology can be self-limiting in addition to regulating other immune effector cells.
Microglia in CNS gliomas
Microglia Chemoattraction and Proliferation in Glioma
Histopathologic studies of glioma tissue have consistently identified a high infiltrating population of microglia within gliomas. Badie and colleagues using flow cytometry showed one-third of glioma cells to be expressing resident microglia markers, and this exceeds the number of other immune cells in gliomas. It has been proposed that microglia accumulation at the site of a CNS tumor is due to the local production of chemoattractants and growth factors. For example, MCP-1 is produced by glioma cells, and microglia have been found to express the MCP-1 receptor, CCR2, on their surface. Thus, local production of MCP-1 may help recruit microglia to the site of glioma. Furthermore, growth factors such as colony stimulating factor-1 (CSF-1), granulocyte colony-stimulating factor (G-CSF) and hepatocyte growth factor/scatter factor (HGF/SF) have been known to be secreted by various gliomas, and these growth factors along with serving chemokine signals also promote proliferation of microglia. Therefore, gliomas are actively promoting microglia trafficking and proliferation by secreting chemokines and growth factors.
Microglia’s Role in Tumor Progression
The recruitment of microglia in brain tumors was postulated as an attempt by the CNS to destroy invading neoplastic cells. However, in recent years, it has become increasingly evident that the immune defense functions of microglia against glioma are compromised because there is no strong evidence of tumor rejection, and tumors grow despite increased microglia invasion over time. Also, the microglia, instead of being localized to the area of necrosis, are found diffusely throughout tumor parenchyma and do not appear to be phagocytosing tumor cells or debris. Growing evidence suggests that glioma infiltrating microglia/macrophages may actually be promoting tumor growth. For instance, though MHC molecule expression has been detected on microglia cells in gliomas, they appear to be deficient in proper antigen presentation for cytotoxic and helper T-cell activation. The number of microglia cells expressing MHC class II antigen is further reduced in high-grade gliomas, despite the abundance of microglia in these CNS neoplasms. Schartner and colleagues demonstrated that microglia isolated from tumor-expressing mice do not show MHC class II upregulation following in vivo stimulation, thereby preventing the induction of an efficient antitumor immune response. Additionally, the expression of MHC class II and co-stimulatory B7 molecules was significantly increased when freshly isolated microglia were cultured in the absence of glioma cells. Alternatively, the interaction of microglia with T cells can inhibit T-cell function by increased expression of inhibitory molecules. In particular, increased expression of co-stimulatory molecule B7-H1 on APCs induces T cell apoptosis. Microglia express B7-H1 in homeostasis, but the expression is upregulated in pathologic conditions including gliomas. Thus, it is likely that upregulation of inhibitory molecules like B7-H1 may contribute to inhibition of T-cell activation, and even apoptosis, regardless of the presence of MHC molecules.
FasL is another inhibitory molecule expressed on tumor-associated microglia that may play a key role in limiting the ability of T cells to recognize and respond to tumor cells. FasL-expressing cells induce apoptosis of CD8 T cells and thus, the upregulation of FasL on tumor-associated microglia may serve as another mechanism to limit tumor recognition by cytotoxic cells. Microglia in intracranial tumors express FasL and the inhibition of FasL activity has been demonstrated to dramatically increase the number of peripheral immune effector cells in tumors. In a study by Parney and colleagues, coculturing human peripheral blood mononuclear cells with human glioma lines caused glioma-conditioned monocytes to reduce phagocytosis and induce apoptosis of activated lymphocytes when added to the glioma-monocyte culture. Kostianovsky and colleagues studied microglia/monocyte activation in GBM. They found that the presence of GBM tumor cells downregulated the production of the proinflammatory cytokine TNF-α by microglia after LPS stimulation and instead, anti-inflammatory cytokine IL-10 was induced. The immunosuppression by microglia was maintained with different stimulus signals such as β amyloid, suggesting that GBM tumor cells affect microglia/monocyte activation regardless of the nature of stimuli. Moreover, glioma cells produce anti-inflammatory cytokines, such as IL-6, transforming growth factor (TGF)-β2, and prostaglandin E2, and tumor-growth–promoting cytokines, such as IL-1 and bFGF. TGF-β2 in particular inhibits proliferation and secretion of pro-inflammatory cytokines by microglia and lymphocytes. These studies together suggest that the phagocytosis, pro-inflammatory cytokine secretion, and antigen presentation functions of microglia are strongly suppressed by glioma cells.
Microglia, under the influence of an immunosuppressive environment, also secrete anti-inflammatory cytokines such as IL-10 that enable rapid proliferation of tumor cells by inhibiting cytotoxic T cell. Additionally in vitro studies have demonstrated that IL-10 suppresses microglia antigen presentation function by inhibiting IFN-γ–induced MHC class II expression. Thus, microglia IL-10 secretion further contributes to the maintenance of an immunosuppressive environment. Microglia are known to be the cellular source of matrix metalloproteinase-2 (MMP-2), extracellular matrix-degrading enzymes, and their release into the tumor environment can help increase the spread of tumors because in the absence of extracellular matrix, tumor cells are free to proliferate. Microglia also secret tumor proliferation promoting factors including epidermal growth factor (EGF) and vascular endothelial growth factor (VEGF), which are well known to be involved in glial cell proliferation and tumor angiogenesis. These studies in combination suggest that microglia play an integrative role in the tumor progression by supporting migration (MMP-9), angiogenesis (VEGF), and proliferation (EGF) of glioma cells.
Therapeutic Potential of Microglia in Glioma
Microglia are potent immune effector cells that display a broad range of functionality, mediating both innate and adaptive responses during CNS injury and disease while remaining quiescent in the steady state. In addition to their significant numbers in gliomas, their versatility in bridging the gap between the immune-privileged CNS and the peripheral immune system makes them a potential candidate in immunotherapy treatment. Microglia associated with CNS tumors do not seem to be active in inducing antitumor T-cell response; there is potential for overcoming glioma-induced immunosuppression, so that activated microglia can enhance CNS immunity against tumors. For instance, signal transducer and activator of transcription (Stat) 3 protein inhibitors have been found to activate microglia and T cell immune responses in malignant glioma. Signal transducers and activators of transcription proteins are a family of transcription factors activated by tyrosine kinases such as epidermal growth factor receptor (EGFR) in response to tumor-secreted factors including VEGF, IL-6, and IL-10. On activation, the Stat proteins translocate to the nucleus and bind to specific elements within target promoters to regulate gene expression. Malignant gliomas are well known for their dysregulation of the EGFR pathway via amplification, mutation of EGFR, and upregulation of Stat3 activation. Stat signaling, in particular Stat3, regulates immune activation and tolerance by altering transcription of several genes that control tumor cell survival, resistance to apoptosis, cell cycle progression, and angiogenesis in glioma cells. These findings suggest that Stat3 is abnormally activated through EGFR dysregulation and contributes to glioma proliferation and immunosuppression.
In vitro studies, Iwamaru and colleagues demonstrated that a novel small molecule, WP1066—a pharmacologic derivative of the natural compound caffeic acid—inhibits Stat3 activation. Stat3 signaling inhibition downregulates gene expression of Bcl-XL, Mcl-1, and c-myc and induces apoptosis resulting in significant inhibition of tumor cell growth in human malignant glioma cell lines U87-MG and U373-MG. Studies by Hussain and colleagues demonstrated that Stat3 inhibition by WP1066 reversed immune tolerance in GBM by upregulating co-stimulatory molecules CD80 and CD86 in glioma-infiltrating microglia and peripheral blood monocytes and inducing effector T cell–stimulating cytokines, such as IL-2, IL-4,IL-12, and IL-15. Collectively, these studies indicate that the inhibition of Stat3 activity could be a promising therapeutic strategy for the treatment of patients with malignant gliomas due to its potent immune adjuvant responses.
Additionally, studies by Carpentier and colleagues demonstrated long-term survival in animals with established CNS glioma using single intratumoral injection of CpG oligodeoxynucleotide, an immunostimulatory sequence that signals through TLR9 to induce production of IFN-α, IFN-β, IL-12, and TNF-α. However, animals with macrophage/microglia depletion were unable to reject tumor after CpG treatment, showing that microglia/macrophages are a critical component of antitumor response. Though CpG is fairly safe in humans, an improved understanding of microglia function in gliomas can provide better methods of manipulating the glioma microenvironment to allow effective antigen presentation to T cells.
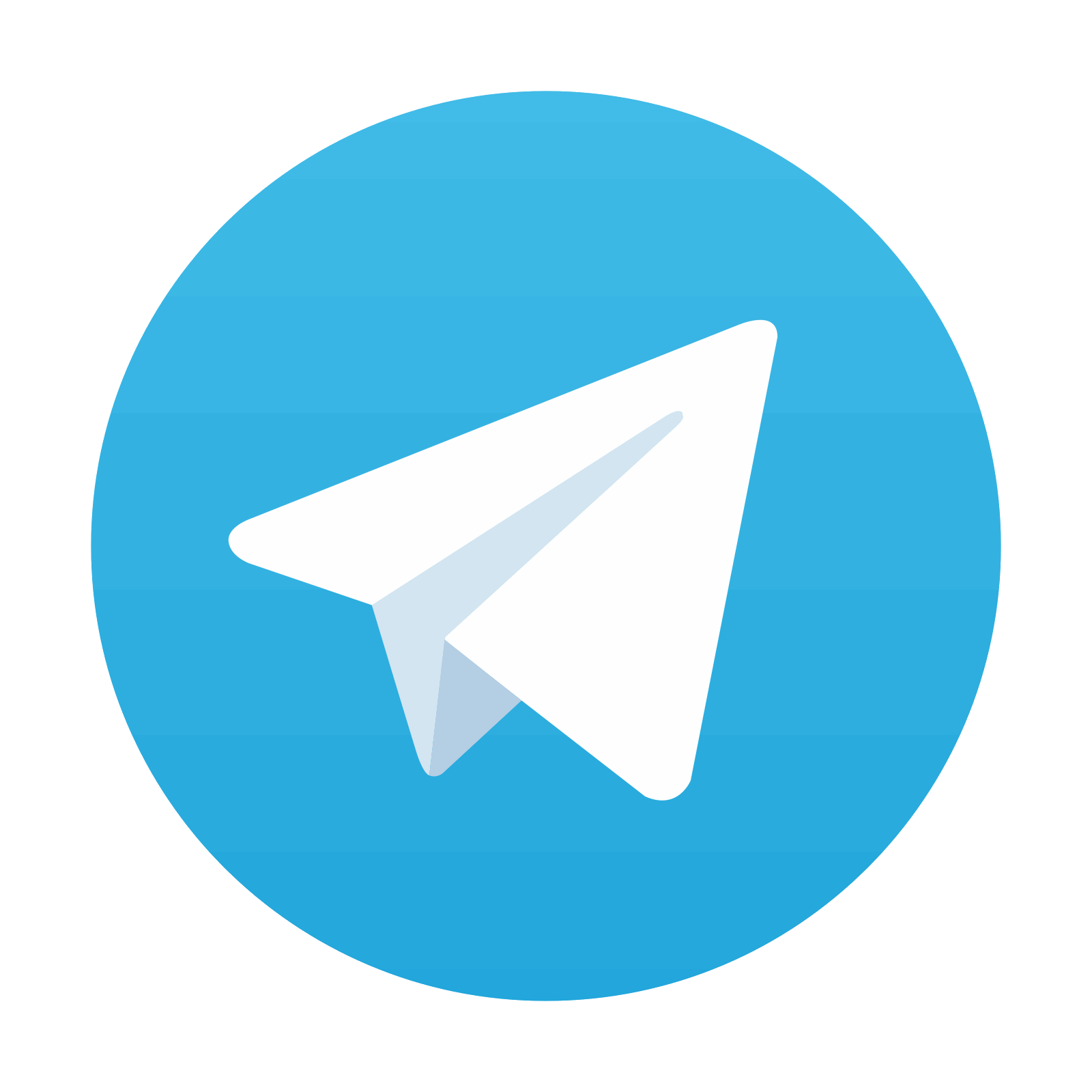
Stay updated, free articles. Join our Telegram channel

Full access? Get Clinical Tree
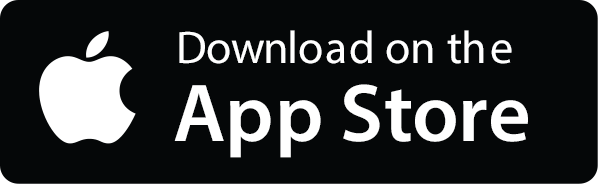
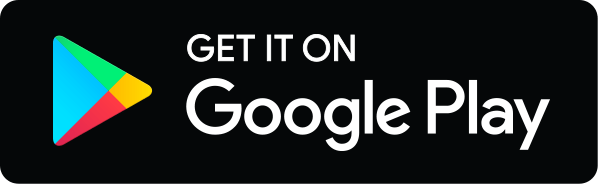