Fig. 14.1
Illustration showing the four segments of the vertebral artery (V1-V4) as well as its relationship to adjacent structures. BA basilar artery, PICA posterior inferior cerebellar artery, SA subclavian artery (Used with permission from Barrow Neurological Institute, Phoenix, Arizona)
The PICA is the second branch vessel to arise from the VA (Fig. 14.2) [3]. This vessel is divided into five segments, designated p1 through p5. The p1 segment (the anterior medullary segment) arises from the VA and travels anterior to the medulla to the hypoglossal rootlets at the medial edge of the olive. The p2 segment (the lateral medullary segment) courses from the medial edge of the olive to the rootlets of the lower cranial nerves at the lateral edge of the olive. The p3 segment (the tonsillomedullary segment) begins at the lateral edge of the olive and extends to the inferior extent of the cerebellar tonsil, coursing rostrally to the midpoint of the medial tonsil. The p4 segment (the telovelotonsillar segment) begins at the midpoint of the medial tonsil, courses past the roof of the fourth ventricle, and extends to the tonsillobiventral fissure. The p5 segment (the cortical segment) is the remainder of the artery distal from the tonsillobiventral fissure and is responsible for irrigating the cerebellar hemispheres.


Fig. 14.2
Illustration showing the five segments (P1-P5) of the posterior inferior cerebellar artery (PICA) , as well as its relationship to adjacent structures. AICA anterior inferior cerebellar artery, BA basilar artery, VA vertebral artery (Used with permission from Barrow Neurological Institute, Phoenix, Arizona)
At the pontomedullary junction, the VAs join to form the BA (Fig. 14.3) [4]. The BA extends from the pontomedullary junction to the mesencephalopontine junction where it bifurcates to form the PCAs. In addition to a rich array of perforating vessels arising from the dorsal surface of the basilar apex, the BA gives rise to large pontine vessels, including the pontomedullary arteries, the posterolateral arteries, and the long lateral pontine branches that supply the brainstem. The height of the basilar bifurcation relative to the bony anatomy of the clivus is of important clinical consideration because the level of the bifurcation can render approaches to the basilar apex challenging in some cases.


Fig. 14.3
Illustration showing the anatomy of the basilar artery and its relationship to adjacent neural structures (Used with permission from Barrow Neurological Institute, Phoenix, Arizona)
The BA gives rise to three paired vessels: these include the AICAs, SCAs, and PCAs.
The AICAs arise from the lower half of the BA, most commonly from a single trunk (Fig. 14.4) [5]. There are four segments to the AICA: the anterior pontine (a1), the lateral pontine (a2), the flocculopeduncular (a3), and the cortical (a4) segments. The a1 extends from its origin to the midpoint of the inferior olive. The a2 extends from the inferior olive to the flocculus and consists of multiple named branches, including the labyrinthine, the subarcuate, the cerebellosubarcuate, and recurrent perforating arteries. The a3 extends from the flocculus to the cerebellopontine fissure. The a4 constitutes the vessel distal to the cerebellopontine fissure.


Fig. 14.4
Illustration showing the anatomy of the anterior inferior cerebellar artery (segments a1-a4) and its relationship to adjacent neural structures (Used with permission from Barrow Neurological Institute, Phoenix, Arizona)
The SCAs originate from the BA proximal to or within 2.5 mm of the PCAs (Fig. 14.5) [6]. The SCA is divided into four segments: the anterior pontomesencephalic (s1), the lateral pontomesencephalic (s2), the cerebellomesencephalic (s3), and the cortical (s4) segments. The s1 extends from the origin to the anterolateral margin of the brainstem. The s2 extends from the anterolateral margin of the brainstem to the cerebellomesencephalic fissure. The s3 is the portion that resides within the cerebellomesencephalic fissure. The s4 is the portion of the vessel distal to the cerebellomesencephalic fissure.


Fig. 14.5
Illustration showing the segments of the superior cerebellar artery (SCA, segments s1-s4) and its relationship to adjacent neural structures. PCA posterior cerebral artery (Used with permission from Barrow Neurological Institute, Phoenix, Arizona)
The PCAs constitute the termination of the BA (Fig. 14.6) [7]. The PCAs have complementary calibers with their associated posterior communicating arteries (PCoA) , a remnant of their development in the embryo (i.e., the caliber of the PCA and PCoA will vary but complement each other to serve the vascular territory). The PCA also consists of four named segments, with branches of clinical importance. The P1 segment is the portion of the vessel from where it branches from the BA to its point of insertion on the PCoA. This portion of the PCA is rich in thalamoperforating arteries, which if injured can result in devastating effects, particularly during surgical treatment of basilar apex aneurysms. The P2 segment is further divided into an anterior (P2a) and a posterior (P2p) segment. The P2a segment is the portion of the PCA from the insertion of the PCoA to the posterior border of the peduncle. It gives rise to the peduncular perforating arteries, the thalamogeniculate arteries, the medial posterior choroidal artery, the anterior temporal artery, and the hippocampal arteries. The P2p is the portion of the vessel from the posterior peduncle to the calcarine fissure. It gives rise to thalamogeniculate arteries, the lateral posterior choroidal artery, and the middle and posterior temporal arteries. The P3 segment is the portion of the PCA from the posterior border of the midbrain to the calcarine fissure. Many cortical arteries branch from the P3 and P4 segments, including the parieto-occipital, calcarine, and splenial arteries.


Fig. 14.6
Illustration showing the segments (P1-P4) of the posterior cerebral artery (PCA) and its relationship to adjacent neural structures. a artery, aa arteries, CN cranial nerve, SCA superior cerebellar artery (Used with permission from Barrow Neurological Institute, Phoenix, Arizona)
Posterior Circulation Aneurysms
Incidence of Aneurysms of the Posterior Circulation
One to five percent of individuals harbor cerebral aneurysms [8]. Aneurysms of the posterior circulation constitute 15–20% of all intracranial aneurysms [9]. The most common location of posterior circulation aneurysms is the BA apex (49–72% of cases), followed by aneurysms of the VA and PICA (which constitute 18–20% of all infratentorial aneurysms). Less commonly, aneurysms arise from the SCA (7–9%), the basilar trunk (2–8%), or the vertebrobasilar junction (9–13%) [10–16]. The mean age of patients harboring aneurysms is between 50.5 and 53.9 years old, and 60–70% of these patients are women [10–16]. Most posterior circulation aneurysms are small (≤11 mm; 47–63%), followed by large aneurysms (12–24 mm; 25–40%) and giant aneurysms (≥25 mm; 6–9%). Women are also more likely to have multiple aneurysms [17].
Presentation of Aneurysms of the Posterior Circulation
The three most common presentations of posterior circulation aneurysms include subarachnoid hemorrhage (SAH) , mass effect, and ischemia [18, 19]. Posterior circulation aneurysms commonly present with sudden onset of headache from SAH. SAH is the presenting symptom in 55–81% of cases [18–20]. Because of the proximity of the vasculature to the brainstem, especially the proximity of the VA to the medulla, these patients may present with sudden onset of loss of consciousness, respiratory or cardiac arrest, or lower cranial nerve dysfunction from aneurysm rupture [20]. Large or giant aneurysms may become symptomatic due to mass effect on the brainstem or the lower cranial nerves or due to hydrocephalus caused by compression of the fourth ventricle. Fusiform or dissecting aneurysms can present with ischemic episodes, likely caused by thromboembolic phenomena resulting in hemiparesis. Smaller aneurysms can be discovered incidentally during imaging workup for other symptoms. In general, 22–45% of posterior circulation aneurysms are unruptured when discovered [10–16]. Due to the proximity of the PCA and SCA to the third cranial nerve, patients with aneurysms arising from these vessels may present with a partial or complete third cranial nerve palsy. Alternatively, the proximity of the SCA to the fifth cranial nerve may result in trigeminal neuralgia as a presenting symptom. Patients with AICA aneurysms may present with facial weakness or hearing loss.
Natural History of Aneurysms of the Posterior Circulation
Aneurysms of the posterior circulation have a more aggressive course and natural history than their counterparts in the anterior circulation [21, 22]. The International Study of Unruptured Intracranial Aneurysms (ISUIA) found that regardless of size, aneurysms of the posterior circulation had a higher rate of rupture over a 5-year period than aneurysms of the cavernous sinus or other anterior circulation locations [21] (Table 14.1). The outcomes for patients with ruptured aneurysms treated conservatively are poor, with rebleeding rates approaching 40% at 4 weeks and mortality from a repeat hemorrhage approaching 50% [23–25]. The common risk factors for development and rupture of aneurysms in the posterior circulation include female sex, increasing age, family history of aneurysms, tobacco use, connective tissue disorders, hypertension, and prior history of SAH.
Table 14.1
Relationship between size and location of aneurysms and the annual and cumulative risk of rupture after 5 years
Aneurysm location | Aneurysm size (% of aneurysms) | ||||
---|---|---|---|---|---|
<7 mm group 1a | <7 mm group 2b | 7–12 mm | 13–24 mm | ≥25 mm | |
Cavernous carotid artery (n = 210) | 0 | 0 | 0 | 3.0 | 6.4 |
AC/MC/IC (n = 1037) | 0 | 1.5 | 2.6 | 14.5 | 40 |
Post-P comm (n = 445) | 2.5 | 3.4 | 14.5 | 18.4 | 50 |
Indications for Interventions for Aneurysms of the Posterior Circulation
The decision to intervene and treat any lesion depends on the natural history of the lesion, the risk associated with treatment versus observation, the experience of the surgeon, intrinsic patient factors (such as health and life expectancy), and the wishes of the patient and family. Because of the aggressive nature of posterior circulation aneurysms compared to anterior circulation aneurysms, posterior circulation aneurysms should be considered for treatment if they occur in patients with a history of SAH, if they exhibit growth, if they are present in a patient with a family history of SAH, if the patient is symptomatic and the symptoms could be attributed to the aneurysm, and if they are of a size that place them at significant risk for rupture based on the ISUIA data. Factors such as patient age, sex, comorbidities, patient wishes, and other risk factors should be taken into account when deciding whether the patient should undergo treatment and the type of treatment that would best suit the patient.
Posterior Fossa Arteriovenous Malformations
Incidence of Arteriovenous Malformations of the Posterior Fossa
AVMs constitute 2% of all hemorrhagic strokes [26]. They are ten times less common than aneurysms but cause 38% of all intracerebral hemorrhages in patients between 15 and 45 years old, and they are a disproportionate cause of morbidity and mortality [27–29]. Posterior fossa AVMs represent 7–15% of all intracranial AVMs, with cerebellar AVMs being the most common subtype (75–82%) [30, 31]. Brainstem AVMs constitute 12.5–23% of cases [30, 31]. The mean age at presentation for all AVMs is 32.8 ± 15 years, and the mean age of patients with AVMs in the posterior fossa is 42 years [32, 33]. There is no gender predilection.
Presentation of Arteriovenous Malformations of the Posterior Fossa
Unlike supratentorial AVMs, AVMs in the posterior fossa rarely present with seizures. Instead, these lesions are more likely to present with hemorrhage (60–86% of cases compared to 34–55% for supratentorial AVMs) [33–35]. Hemorrhage from AVMs in the posterior fossa may be subarachnoid, intraventricular, or intraparenchymal. Hemorrhage into the ventricular space can result in hydrocephalus.
The second most common presentation of posterior fossa AVMs is progressive neurological deficit (seen in nearly 1/3 of cases) [34]. These symptoms may be due to ischemia, mass effect, or hydrocephalus. Less common presentations include cranial nerve palsies, gait instability, cerebellar symptoms, hemiparesis, and headache.
Natural History of Arteriovenous Malformations of the Posterior Fossa
As discussed above, posterior fossa AVMs are more likely to present with hemorrhage than their intracranial counterparts [36–38]. The annual risk of hemorrhage for all AVMs is estimated to be between 2% and 4%, but rates as low as 1% have been reported [32, 36, 37, 39–44]. Each episode of hemorrhage is associated with a 15–20% risk of significant morbidity and mortality [32, 38, 42–44]. Mortality associated with hemorrhage has been reported to be as high as 67% for patients with posterior fossa AVMs [45]. In one of the largest data sets on the natural history of AVMs, Hernesniemi et al. determined that posterior fossa AVMs have a relative rupture risk of 3.07 compared to supratentorial AVMs [32]. The risk of hemorrhage increases in patients with a history of a prior hemorrhage. Ruptured AVMs have an annual rerupture rate of 6–7%, compared to a rate of 2–3% for previously unruptured AVMs. The 5-year risk of rupture of a previously ruptured AVM can approach 26%, while that of an unruptured AVM is 10% [32]. Other features that could increase the likelihood of hemorrhage include the presence of flow-related and intranidal aneurysms [46–48], venous outflow stenosis [49], and high feeding artery pressures [50, 51].
Indications for Interventions for Arteriovenous Malformations of the Posterior Fossa
Patient selection is critical in order to optimize outcomes for AVM treatment. Although a full discussion of patient selection is beyond the scope of this chapter, we refer the reader to the excellent reviews on patient selection and considerations in AVM surgery that are available [52–56]. The Spetzler-Martin grading scale provides a paradigm for selecting an appropriate treatment algorithm and ascertaining the likely risk of morbidity from surgical treatment [57]. In general, patients with low-grade AVMs (Spetzler-Martin grade I and grade II lesions) should be considered for treatment with surgery, regardless of presentation. Patients with high-grade lesions (Spetzler-Martin grade IV and grade V lesions) whose lesions are identified incidentally should be monitored closely, although a subset may undergo multimodality treatment with good outcomes [58]. Patients with intermediate-grade AVMs (Spetzler-Martin grade III lesions) should be considered on a case-by-case basis [57, 59, 60]. In patients who present with hemorrhage, the surgeon may be forced to intervene, despite a high-grade lesion, in order to prevent devastating consequences from the hemorrhage [61]. In many cases, the surgeon may evacuate the hematoma in the acute setting to ameliorate symptoms caused by mass effect until definitive treatment can be considered. Patients who present with incidental lesions that demonstrate high-grade features may undergo selective treatment of the high-risk component of their AVM, but selective treatment, especially with endovascular techniques, is not without risk [62, 63]. Although rare, patients with posterior fossa AVMs may present with seizures due to vascular steal. In this cohort with high-grade lesions, selective embolization may relieve the symptoms caused by steal [64].
Brainstem and Cerebellar Cavernous Malformations
Incidence of Cavernous Malformations of the Brainstem and Cerebellum
The incidence of cavernous malformations in the general population is estimated to be on the order of 0.5%, meaning that 1 in 200 individuals harbor a cavernous malformation [65]. Cavernous malformations are distributed in the supratentorial and infratentorial compartments in proportions roughly equal to the volume of the brain. Supratentorial lesions constitute 60–90% [66, 67] of all cavernous malformations, while infratentorial lesions account for 8–36% of all cases [68–72]. In the posterior fossa, the brainstem [73] is the involved site in 4–35% of cases [67]. Brainstem cavernous malformations account for 13% of all vascular malformations of the posterior fossa [73]. The remainder of the cavernous malformations of the posterior fossa are located in the cerebellum, and cerebellar cavernous malformations account for 1–12% of all intracranial cavernous malformations and 9.3–52.9% of all infratentorial lesions [67, 74].
Presentation of Cavernous Malformations of the Brainstem and Cerebellum
The mean age of patients who present with symptomatic cavernous malformations ranges between 32 and 38 years [66, 75, 76]. In patients who present with bleeding from a posterior fossa cavernous malformation, the most common symptoms are headache, nausea, vomiting, gait disturbances, and symptoms attributable to various cranial nerve palsies. The site of the hemorrhage determines the type of neurological deficit. These deficits can include hemiparesis, facial and abducens nerve palsies, internuclear ophthalmoplegia, and sensory disturbances, among others [66, 71, 76]. Fatal hemorrhage from brainstem cavernous malformations is rare, and with few exceptions, most patients experience improvement of their symptoms after hemorrhage, making the decision on the timing of surgery a rather complicated point of discussion with patients.
Natural History of Cavernous Malformations of the Brainstem and Cerebellum
A discussion of the natural history of cavernous malformations is beyond the scope of this chapter. Readers are referred to other recent sources for an extensive discussion [77]. In short, the annual risk of hemorrhage from a cavernous malformation without a previous history of hemorrhage ranges between 0.6% and 1.1% per year [72, 78]. This rate increases significantly in patients who have had a previous episode of hemorrhage, and in the posterior fossa, this risk may be as high as 25% per year in the year immediately after hemorrhage [79]. Evidence also exists for clustering of hemorrhagic events, which may explain this rather high rate in the year immediately after the initial hemorrhage [65, 77, 80, 81]. Although patients may suffer from neurological deficits after a hemorrhage, it is important to remember that neurological recovery is the rule rather than the exception after a hemorrhage, and this fact should be considered when advising patients regarding surgery. Samii et al. [82] reported that 16.7% of the patients in their series of surgically treated brainstem cavernous malformations had completely recovered before surgery. Kupersmith et al. [83] reported that 37% of patients in their series had recovered completely, while Li et al. [84] reported complete recovery in more than a quarter of their patients (28.7%).
Indications for Intervention for Cavernous Malformations of the Brainstem and Cerebellum
Because of the more aggressive natural history of posterior fossa cavernous malformations, surgical resection is indicated in patients whose lesions abut a pial or ependymal surface or in patients with fixed and permanent deficits [85]. The goal of surgery is to eliminate repeated episodes of hemorrhage that may cause the patient to suffer additional morbidity or even death; therefore, resection should be complete when possible. In patients in which the lesion does not abut a pial or ependymal surface or in patients in which the lesion is identified incidentally, conservative monitoring is a perfectly reasonable approach. However, we do recommend resection for lesions that may not abut a pial plane but that can be accessed using a safe-entry zone [86] with acceptable morbidity or for lesions that have undergone repeated episodes of hemorrhage . For surgical timing, there is no consensus regarding when lesions should be resected after a hemorrhage. As previously stated, most patients improve and recover most of their neurological function after a hemorrhage, so the decision may be made to follow the patient after a hemorrhage to see how much function is regained. Alternatively, surgery immediately after a hemorrhage may relieve mass effect caused by the hemorrhage and may allow a patient to recover more readily from the bleed by removing blood and toxic blood products from the eloquent structures of the brainstem.
Preoperative Evaluation
Patient History and General Considerations
The preoperative evaluation of patients with posterior circulation vascular lesions should begin with a thorough history and physical examination [87]. The history should consist of family history of aneurysms or vascular malformation, personal history of prior hemorrhage, predisposing factors such as hypertension, fibromuscular, and collagen vascular disorders, and disorders such as Osler-Weber-Rendu syndrome. The patient’s social history, specifically smoking and illicit drug use, should be obtained. History of recent infection, trauma, cancer, or immunosuppression should also be obtained, as these can predispose patients to the risk of rare aneurysm types, such as infectious or traumatic aneurysm formation. The patient should be asked to describe symptoms and durations because some patients may have experienced a sentinel bleed, a forbearer of a larger eventual hemorrhage from an aneurysm or vascular malformation. The patient should also be questioned regarding nausea, vomiting, or a history of transient weakness, which may be indicative of compressive or ischemic etiology. A review of patient medications often reveals much about other underlying conditions that could predispose the patient to the formation of aneurysms or ischemic lesions in the posterior circulation. Additionally, medications such as antiplatelet and antithrombotic medications should be noted. Allergies to medications can also reveal much about patient physiology. Specifically, any allergy to common antiplatelet medications should be noted.
A detailed physical examination, including a thorough neurological examination , should be performed to evaluate for alterations in mood, cognition, new onset or progressive weakness, gait instability, evidence of cerebellar dysfunction, brainstem symptoms, and cranial nerve deficits.
Patients undergoing microsurgical treatment of vascular pathologies should undergo routine laboratory testing including a basic metabolic panel, a complete blood count, coagulation status, and a chest x-ray. Patients with a history of cardiac disease should be evaluated and cleared by a cardiologist for surgery. An additional group of patients who require close medical workup are those who will undergo surgery in a sitting position because the presence of a patent foramen ovale in this position can result in preventable complications.
Diagnostic Imaging
In patients who present with sudden onset neurological alterations, the initial evaluation should include computed tomography (CT). CT has a sensitivity of nearly 100% for detecting SAH immediately after ictus [88]. When hemorrhage is not shown on CT but the patient has a history concerning for hemorrhage, a lumbar puncture should be performed to evaluate the presence of any blood cells. All patients who are suspected of having vascular lesions in the posterior circulation should undergo a CT angiography (CTA) study. CTA with three-dimensional reconstruction protocols can evaluate the vascular tree, including collateral circulation and can identify aneurysms, dissections, vascular malformations, and vascular malignancies. CTA has a sensitivity of 96% for aneurysms as small as 3 mm, but may underestimate the size of partially thrombosed aneurysms [89, 90]. For cases of suspected vascular lesions, we favor obtaining imaging of the vasculature of the head and neck.
An alternative to CT is magnetic resonance imaging (MRI) and magnetic resonance angiography (MRA). These modalities permit improved visualization of soft tissues and better assessment of aneurysm size compared to CT. MRI can help delineate the proximity of the vascular malformations to eloquent brain regions. Because blood of various ages has different signal intensities, MRI can help identify the age of any hemorrhage from cavernous malformations. Fluid-attenuated inversion recovery (FLAIR) MRI sequences have a sensitivity approaching that of CT for detecting acute hemorrhage [91]. Fine-cut fast imaging employing steady-state acquisition (FIESTA) MRI sequences can help identify vascular compression syndromes in the confines of the posterior fossa [92, 93].
The gold standard for workup of vascular pathologies in the posterior fossa is digital subtraction angiography (DSA) . Biplane rotational DSA with three-dimensional reconstruction provides dynamic views of blood flow, which can assist surgeons with surgical planning. DSA can evaluate collateral circulation, and by applying selective and sequential compression or temporary occlusion of inflow vessels to the brain, by using the Alcock’s test, for example, can provide essential information for planning treatment. In patients with AVMs, DSA can provide important information regarding the extent of shunting, flow-related aneurysms, and evidence of venous outflow obstruction. In some cases, DSA can be combined with endovascular intervention as a part of a multimodality treatment strategy [62], for example, combined endovascular and microsurgical treatment of AVMs and aneurysms.
Operative Adjuncts
Neuromonitoring and Mapping
We routinely use intraoperative neuromonitoring for neurovascular surgery in the posterior fossa, including monitoring of somatosensory evoked potentials, motor evoked potentials, and cranial nerve-specific monitoring, depending on the location of the lesion in the posterior fossa [94, 95]. Intraoperative neuromonitoring provides real-time output of the function of pathways that may be injured during the operation. Caution should be used, however, because of the dependence of these techniques on the type and depth of anesthesia . A neuroanesthesia team that is proficient with the use of monitoring is critical to properly perform the operation. In addition to monitoring the function of pathways, electrophysiological mapping of critical nuclei is possible, especially during operations on the brainstem for intrinsic lesions .
Intraoperative Evaluation of Blood Flow
Several well-accepted adjuncts exist for evaluating intraoperative blood flow. The most common modalities include intraoperative angiography, which provides similar information to traditional DSA, indocyanine green angiography (ICG) , and intraoperative ultrasonography. ICG is now widely used in operating theaters and has replaced the need for intraoperative angiography in many cases [96–98]. In this technique, a fluorescent dye is administered that allows the blood flow in vessels to be visualized in real time. ICG can be used to confirm the patency of vessels and occlusion of flow from an aneurysm dome during aneurysm surgery. ICG can help identify inflow arteries and draining veins during AVM surgery (although ICG is most useful for superficial lesions, and its application for deep AVM surgery is debated). Quantitative ICG can provide flow measurements [99, 100]. Alternatively, handheld Doppler ultrasonography probes can be used to evaluate blood flow measurement in the operating room. The best application of this technology is for the selection of donor and recipient vessels during bypass surgery and for confirming patency of inflow and outflow vessels before and after aneurysm clipping [101, 102].
Cerebral Protection and Hypothermia
Cerebrovascular surgical procedures have the potential for significant injury to neural structures. Hypotension, retraction, excessive blood loss, iatrogenic injury, hemodilution, hypo- and hyperglycemia, and hypoxia can all cause ischemic injury. Maneuvers that decrease cerebral metabolism greatly prolong the amount of time that the brain can tolerate ischemia. For that reason, all operations should be performed under pharmacological cerebral protection (using barbiturates or propofol) with mild hypothermia (33 °C) [103–105].
Pharmacological Cardiac Arrest
Circulatory arrest is an important adjunct for the treatment of cerebrovascular lesions . In properly selected patients, circulatory arrest greatly facilitates the surgical treatment of aneurysms and AVMs, but it is associated with a high risk of complications [106]. As a result, the use of hypothermic circulatory arrest has largely been abandoned, particularly due to the introduction of well-tolerated pharmacological alternatives. The introduction of adenosine has transformed circulatory arrest from an invasive procedure to one that is much better tolerated, safer, and more transient than hypothermic circulatory arrest. Moreover, adenosine produces reliably reproducible arrest durations. Adenosine allows the surgeon to decrease flow into an aneurysm (or a ruptured AVM), allowing for vascular control and final intervention for the lesion [107]. In aneurysms, the use of adenosine allows the surgeon to obtain proximal and distal control, or it provides the relaxation necessary to allow proper placement of the clip across the neck of the aneurysm. In AVMs, the use of adenosine allows the rupture point to be identified and controlled. Akin to other methods of cardiac arrest, rapid ventricular pacing allows for a reproducible period of cardiac arrest, necessary for the final steps of aneurysm dissection or for identification of bleeding points, especially in the confines of the posterior fossa. Unlike adenosine arrest, rapid ventricular pacing is titratable, and controlled periods of arrest can be obtained with this technique.
Approaches and Approach Selection to Posterior Fossa Vascular Lesions
The choice of approach for any lesion should take certain basic parameters into consideration. These parameters include the shortest approach (when possible), an approach that allows the surgeon to readily visualize the lesion or lesions while minimally disturbing other eloquent neurovascular structures, thereby limiting patient morbidity, and the approach that is most convenient to the surgeon due to handedness and experience. For intrinsic lesions, such as cavernous malformations of the brainstem, the two-point method is an excellent starting point for approach selection but must be combined with knowledge of safe-entry zones for optimal approach selection [86]. The judicious use of skull base approaches allows for adequate visualization of the contents of the posterior fossa, without undue risk of injury to the critical structures (Fig. 14.7). An important tenet of skull base surgery is the removal of bone and minimization of tissue and brain retraction to achieve the necessary visualization and working trajectory. Some general approach-related considerations are presented below; however, the specific steps of the various surgical approaches are not outlined, and the reader is referred to other material for details of the approaches [85, 86, 108–110].


Fig. 14.7
Skull base approaches to the posterior fossa (Used with permission from Barrow Neurological Institute, Phoenix, Arizona)
Approaches to the Ventral Midbrain/Posterior Fossa
Approaches to the ventral midbrain include the pterional, orbitozygomatic, and anterior petrosectomy approach and their variants. Aneurysms of the basilar apex, proximal PCA, and SCA can be readily exposed using the pterional, subtemporal, supraorbital, modified orbitozygomatic, and full orbitozygomatic approaches (Fig. 14.8) [111]. These ventrolateral approaches allow the surgeon to release cerebrospinal fluid from the basal cisterns and develop working corridors between the carotid artery, optic nerve, and oculomotor nerve to arrive at the region of the basilar apex. These approaches are also well suited for intrinsic lesions that lie in the ventral midbrain. Exposure using these surgical corridors leads the surgeon to two safe-entry zones on the ventral midbrain, the anterior mesencephalic and the interpeduncular safe-entry zones, which can be accessed to remove lesions from the lateral and centeromedian mesencephalon [85, 86, 112]. The addition of an anterior petrosectomy [113] allows the surgeon to expose the basilar trunk down to the level of the sixth cranial nerve. This additional exposure can be used to treat aneurysms of the BA or the AICA, as well as cavernous malformations located at the mesencephalic-pontine junction on the ventral surface of the brainstem.


Fig. 14.8
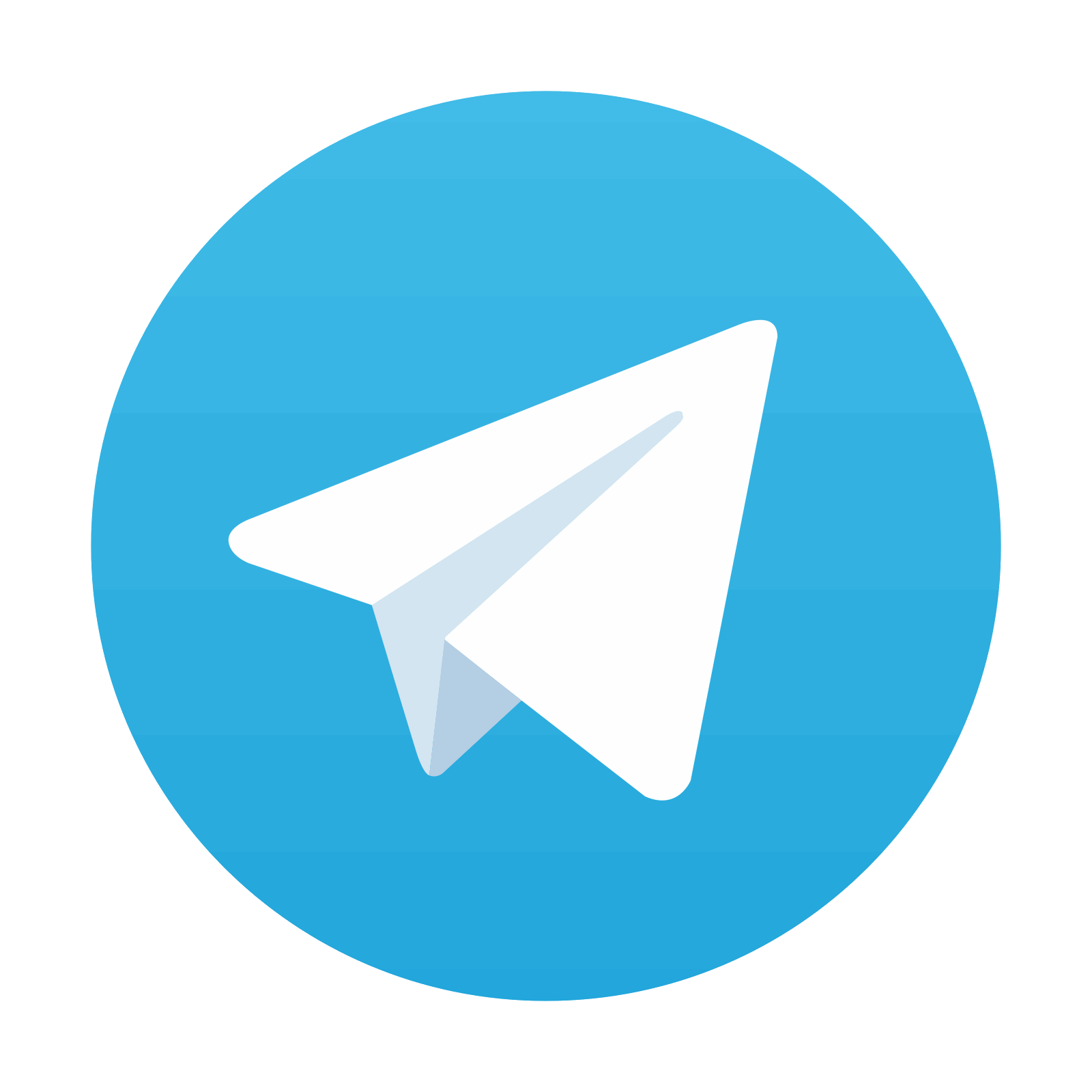
Orbitozygomatic approach to a basilar apex aneurysm. A 61-year-old woman with history of more than 40 pack-years of smoking presented for evaluation after workup for headaches. (a, b) Magnetic resonance angiography revealed two aneurysms, a right middle cerebral artery (MCA) aneurysm (dashed arrow) and a basilar apex aneurysm (solid arrow). The lesions were approached using a right orbitozygomatic craniotomy. After the Sylvian fissure was widely split, three operative corridors to the basilar apex were explored. These included the supracarotid, carotico-oculomotor, and optico-carotid working corridors. Next, the arachnoid on the medial surface of the third nerve was dissected to allow it to fall away with the temporal lobe. (c) After Liliequist’s membrane was opened, the contents of the posterior fossa, including the basilar apex aneurysm, were identified. The basilar perforators, emanating from the posterior surface of the basilar artery, were carefully dissected prior to application of the clip. (d) Indocyanine green angiography revealed occlusion of the basilar apex aneurysm (long arrows) and preservation of the flow in the perforator. Postoperatively, the patient was neurologically intact. (e) Postoperative computed tomography angiography demonstrated complete occlusion of the aneurysm, and (f) postoperative magnetic resonance imaging revealed no infarction (a, b, e, f are used with permission from Journal of Neurosurgery, and c, d are used with permission from Barrow Neurological Institute, Phoenix, Arizona)
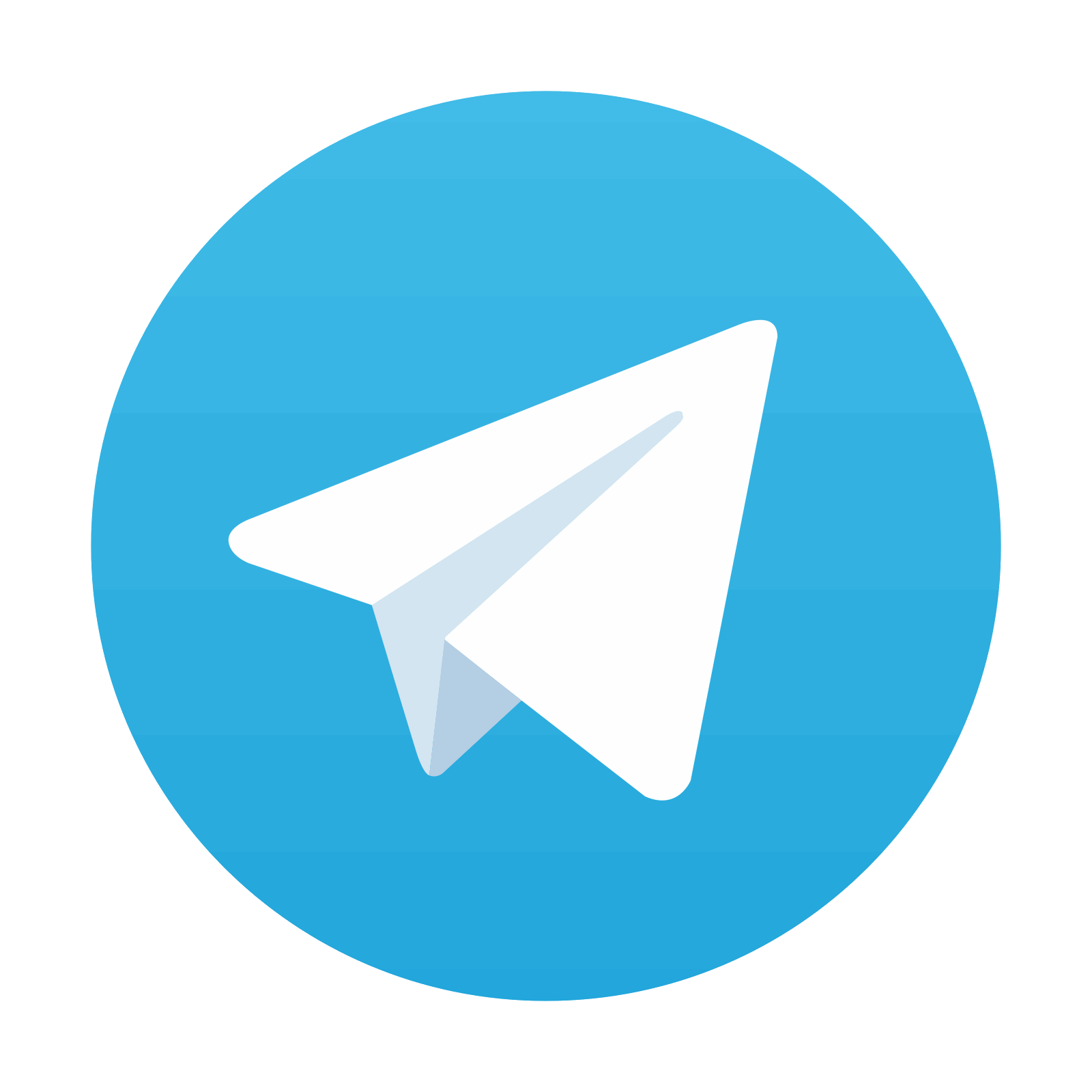
Stay updated, free articles. Join our Telegram channel

Full access? Get Clinical Tree
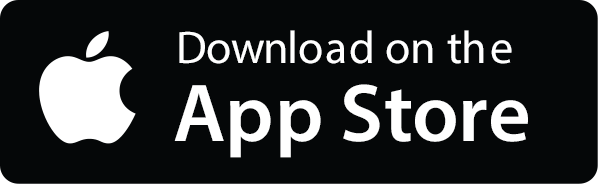
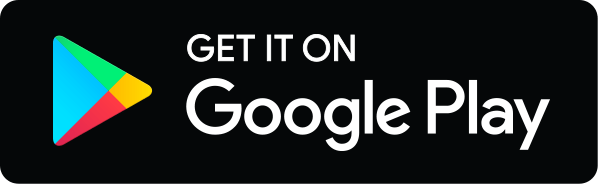
