Introduction
Laser interstitial thermal therapy (LITT) is a minimally invasive treatment modality for brain tumors and other central nervous system (CNS) disorders, first introduced by Bown in 1983, which has been revived over the last 2 decades because of recent technological advancements in laser technology and MRI thermography. At that time, the main limitations of this surgical technique were the inability to monitor or predict the extent of ablation with real-time imaging feedback and the lack of an effective cooling system that could prevent overheating followed by tissue carbonization and optical fiber damage. These pitfalls have now been overcome and LITT is an US Food and Drug Administration–cleared treatment option for ablating CNS tissue such as recurrent glioblastoma, and is emerging as a surgical option for upfront treatment of selected patients with malignant gliomas, brain metastatic disease that failed radiosurgery and some forms of epilepsy. LITT is ideally suited, but not limited, to patients with tumors located in deep-seated, hard-to-access areas who could develop significant postoperative neurologic deficits after traditional surgical resection leading to poor performance status. The basic biological effect of LITT is thermal damage. Laser near-infrared photons are absorbed by the surrounding tissue, causing excitation and release of thermal energy. Ultimately, a cascade of events leads to cell breakdown and coagulative necrosis of the target volume. As clinicians move toward a more individualized approach in cancer care based on the biomolecular profile of different types of tumors, neurosurgeons also offer surgical individualized options for each patient based on their clinical history, performance status, tumor characteristics (size, location), and complication profile of different surgical approaches.
Physical and biological basis for the use of laser therapy in the ablation of glioblastoma
LITT exerts its biological effect by inducing thermal damage of the targeted tumor. Laser electromagnetic photons are emitted by the laser source and absorbed by surrounding tissue, causing excitation and release of thermal energy, which is transformed to heat and distributed to nearby structures via convection and conduction. The degree of direct heat penetration into surrounding tissue is determined by the properties of the tissue and the wavelength and power density delivered by the laser. Previous studies have shown that the main determinants of laser absorption by tissues are the water and hemoglobin content present. In terms of depth, the greatest degree of tissue penetration, which is several millimeters, is observed with laser radiation at wavelengths in the near-infrared part of the spectrum (1000–1100 nm). In addition, the depth of interstitial thermal damage and subsequent necrosis depends on the cooling conditions, power density, and exposure time. It is also known that the results obtained after laser interaction with white and gray matter are different. Although white matter displays the lowest level of laser penetration, gray matter shows a higher level of laser absorption. Eggert and Blazek were able to show that, within the near-infrared spectral range, glioblastomas and meningioma had the highest degree of laser absorption, whereas low-grade gliomas had optical properties similar to gray matter. LITT triggers a cascade of cellular events that include enzyme induction, denaturation of proteins, cellular membrane breakdown, coagulation necrosis, and blood vessel sclerosis. Two phenomena should ideally be avoided when applying LITT: tissue carbonization and vaporization. Rapid increases in temperature can result in tissue carbonization, preventing adequate laser absorption. Overheating can also cause tissue vaporization, which, if sufficiently severe, could lead to increased intracranial pressure with potentially disastrous consequences. A goal of LITT is to achieve coagulation necrosis of the target volume without provoking carbonization or vaporization of the treated area. Coagulation necrosis occurs at temperatures in the range of 50°C to100°C. Carbonization and vaporization are usually seen at temperatures greater than 100°C.
MRI thermography provides real-time thermal data allowing surgeons to monitor the extent of ablation in an effective and safe manner. Its principle relies on the temperature-dependent water proton resonance frequency (PRF). PRF image mapping is based on the fact that protons are displaced more efficiently within the magnetic field in the form of free water molecules (H 2 O) than in the form of hydrogen bonded water molecules. As thermal energy is delivered during LITT and temperature increases, the number of hydrogen bonds decreases, resulting in an increased number of free H 2 O molecules and a lower PRF, which can be visualized with MRI thermometry coupled with advanced computer software in real-time fashion.
Three zones of specific histologic changes around the laser probe are observed during LITT. The first zone is the area closest to the tip of the probe and represents the area of greatest tissue damage caused by the highest degree of thermal energy absorption. The second or intermediate zone also undergoes thermal injury. Tissue cells located in the third and most marginal zone, although damaged by thermal energy, are still viable. True coagulation necrosis is observed in the first 2 zones. The NeuroBlate System (Monteris Medical Corporation, Plymouth, MN) displays the 3 zones of thermal damage in the computer software as the thermal-damage-threshold lines (TDT lines), through real-time imaging data acquired by MRI thermography. This distinctive characteristic of the NeuroBlate System gives surgeons the capability of performing an effective and complete ablation of the tumor by including the target volume in the first 2 zones (TDT lines). Optimal laser ablation is achieved when a sharp border of thermal injury is observed at the brain-tumor interface characterizing a selective procedure with preservation of the normal brain tissue surrounding the tumor.
Historical context and evolution of technologies used in the minimally invasive laser treatment of glioblastomas
Despite recent advances, surgery of high-grade gliomas remains a challenge, especially in recurrent cases and deep, difficult-to-access regions, where access for aggressive surgical resection is often not possible. The median survival with optimal treatment is less than 15 months in glioblastoma and, in cases of recurrent glioblastoma, the overall survival is about 3 to 5 months and treatment options are limited, such as second-line chemotherapy, brachytherapy, and additional surgery. Additional surgery has increased the overall survival by 8 weeks and often is not an option because of difficult-to-access tumor location in deep or eloquent areas. Focal therapies such as stereotactic radiosurgery and brachytherapy have limited results for recurrent glioblastoma, so the use of these treatments remains controversial. Therefore, with the goal of treating patients with tumors deemed inoperable and/or located in eloquent areas, the use of LITT for recurrent glioblastoma has increased and studies have shown the safety of the procedure.
LITT is a minimally invasive treatment modality for brain tumors, a concept that has existed since the late 1970s, but was first described by Bown in 1983. The use of hyperthermia for the treatment of tumors is based on the selective tumor response during tissue heating. Hahn showed not only that heating has the benefits of thermal ablation but also that some drugs can be enhanced by increased temperatures. Further, after multiple initial attempts, such as ruby, argon, and CO 2 lasers, the most effective neuronal tissue damage was achieved in the near-infrared part of the electromagnetic spectrum. These initial studies have led to specific considerations of the interaction between laser energy and brain tissue and the resultant lesion obtained by the application of laser. Laser emission parameters (wavelength, frequency, and laser mode), specific tissue optical and thermal properties (water and hemoglobin content, thermal conductivity, and specific heat) associated with the intensity and time of exposure determine the desired lesion effect on the exposed tissue, which can be represented by coagulation, hemostasis, cutting, or vaporization. Bown conducted several studies in experimental photocoagulation of gastric ulcers, and, in 1983, the author first described an experiment using a neodymium-doped yttrium-aluminum-garnet (Nd:YAG) laser in a brain tumor model achieving coagulation necrosis without surrounding tissue vaporization. In 1990, Sugiyama and colleagues described the use of LITT for the treatment of brain tumors using a computed tomography stereotactic technique, but, because of the high risk of thermal damage to the surrounding tissue and low efficacy, acceptance was limited. At that time, LITT technologies did not gain popularity because of a lack of precision in the thermal energy source, limited ablative volume, and an inability to monitor laser-induced tissue damage in real time. During the past decades, with the advance of MRI technologies, thermal-induced lesions have been monitored by magnetic resonance (MR) thermal imaging, based on PRF. Nowadays, LITT is capable of delivering a controlled, customizable, and precise thermal ablation.
Recently, there have also been important technological and design advances in the development of new LITT probes. Initial probes used bare-tip fiberoptic probe designs and, consequently, technical issues related to overheating, tissue carbonization, and fiber damage were noted. Nowadays, enclosed fiberoptic probe design with special cooling systems, such as constant fluid (saline or water) or cooled gas (CO 2 ) mechanisms, allows better results. Classically, thermal energy at the probe tip resulted in ellipsoid lesions along the axis of the fiber. Now, with advances in cooling and probe designs, side-firing laser probes allow asymmetric tissue penetration and better control of laser ablation in cases of complex-shaped tumors.
Although survival of patients with newly diagnosed glioblastoma benefits from gross total resection (GTR) compared with chemotherapy and radiation alone, in specific situations GTR is not possible and LITT can be considered as a primary surgical option. In particular, patients with tumors in deep, difficult-to-access locations, low functional status scores, multiple comorbidities, and nonsurgical recurrent glioblastoma can benefit from this minimally invasive therapy.
In addition, several other LITT indications have been reported, such as radiosurgery-resistant brain metastatic disease, epilepsy, and radiation necrosis. Initial technical difficulties have now been overcome and the safety of this technique has been established for future studies and clinical application.
Current evidence and techniques for the treatment of glioblastoma with laser interstitial thermal therapy (NeuroBlate System)
Primary glial neoplasms account for approximately 28% of all primary brain tumors and 80% of all malignant brain tumors. Of primary glial neoplasms, glioblastoma multiforme (WHO grade IV) accounts for approximately 45% of these tumors. Microneurosurgical resection with the aim of achieving GTR followed by concurrent chemotherapy (temozolomide) and radiation therapy remains the mainstay treatment of newly diagnosed glioblastoma. Despite the best available treatment, the overall prognosis remains poor, with 3-year and 5-year overall survival rates of 16.0% and 9.8%, respectively. In addition, median survival and progression-free survival have been reported to be 14.6 months and 6.9 months respectively. Patients with progressive or recurrent glioblastoma have been shown to have worse outcomes, with a median survival ranging between 6.2 and 9.2 months. Various chemotherapeutic options, such as salvage temozolomide, irinotecan, carmustine wafers, cetuximab, or carboplatin alone or in combination with antiangiogenic agents (bevacizumab), have been used in patients with recurrent disease with varied results. A recent meta-analysis reported an improved median and progression-free survival with a combination of bevacizumab and irinotecan (compared with bevacizumab alone) in patients with recurrent glioblastoma, but the toxicity and ill effects of this combination on quality of life led to a high discontinuation rate.
Surgical options for patients with recurrent or progressive disease are limited, with controversial data in the literature. Young age at diagnosis, good performance status (Karnofsky performance scale [KPS] score at recurrence), and extent of resection at recurrence have been shown to prolong survival in patients with recurrent glioblastoma, irrespective of the extent of resection at initial surgery. Note that, after adjusting for age, multiple resections have not been shown to be a predictor of overall survival in patients with recurrent glioblastoma. Multiple surgical resections in combination with chemoradiotherapy have been shown to prolong survival in patients with recurrent disease (median survival, 26 months for multiple resections vs 16 months for 2 resections followed by nonsurgical management). Repeat surgical resections for recurrent tumors are usually performed in patients with good performance status and may reflect a selection bias in these studies. Moreover, repeat surgical resections are associated with 18% to 22% incidence of postoperative neurologic deficits. Incidence of neurologic deficits has been shown to increase from 4.8% during the first surgical resection, to 12.1% in the second surgery, to 8.2% in the third, and to 11.1% during 4 or more resections for recurrent glioblastoma. Radiosurgery, fractionated radiation therapy, and interstitial brachytherapy have limited roles in these patients. Given the risks associated with repeat surgical resections in combination with a paucity of adjunct treatment modalities, LITT is an appealing alternative treatment option in selected patients with recurrent glioblastoma. Also, for patients with recurrent glioblastoma with significant medical comorbidities or those who do not wish to undergo traditional open surgical approaches, LITT can be a reasonable treatment option.
Maximal tumor cytoreduction or GTR is thought to improve outcomes in patients with either newly diagnosed or recurrent glioblastoma. LITT can achieve maximal tumor cytoreduction via photocoagulation of pathologic tissue, similar to the cytoreduction that can be achieved by open surgical techniques. Moreover, LITT is associated with less neurologic morbidity and mortality compared with open surgical techniques, especially for deep-seated or difficult-to-access tumors. Laser interstitial thermotherapy has also been shown to disrupt the blood-brain barrier (BBB) with neovascularization in animal models, which may have favorable implications for the effectiveness of early chemotherapy. Given these advantages, LITT can also be a reasonable alternative upfront therapeutic option in carefully selected patients.
Laser Interstitial Thermal Therapy for Recurrent Glioblastoma
Several studies have reported the utility of LITT in patients with recurrent glioblastoma. Literature documenting the efficacy of LITT in a patient with recurrent glioblastoma dates back to the early 1990s. In 1998, Reimer and colleagues reported the use of LITT in a patient with recurrent gliosarcoma (20 × 35 mm) involving the left precentral gyrus. Tumor progression was noted at 8 months within the ablated zone. Later, Leonardi and colleagues performed 9 LITT procedures in 6 patients with recurrent/residual disease (1 newly diagnosed). The procedure was performed under local anesthesia using 1064-nm Nd:YAG laser and 0.2-T MRI. Clinical outcome and tumor response rates were not reported in this study, but the investigators noted no correlation between tumor grade and tissue response to thermal therapy. A year later, the investigators reported overall survival and progression-free survival of 9 months and 4 months respectively in 6 patients with recurrent glioblastoma. Good functional status (KPS >70) was maintained for 7.5 months in these patients following LITT. Another report of 2 cases with recurrent glioblastoma (case 1: multifocal disease diagnosed during follow-up and the lesion was treated primarily with LITT. Case 2: true recurrence) reported an overall survival of 13 months (16 months since recurrence) and 15 months (20 months since recurrence) following LITT. A year later, the same investigators reported the utility of LITT in 16 patients (26 LITT procedures) with recurrent glioblastoma and a mean follow-up of 9.1 ± 6.3 months. This study reported a difference in median survival between the first 10 patients and later 6 patients of 5.2 months and 11.2 months, respectively, which the investigators attributed to the time delay between diagnosis of tumor recurrence and LITT (ie, learning curve). There was an overall median survival of 6.9 months following LITT and 9.4 months after first relapse.
Recently, Carpentier and colleagues reported the utility of salvage MR-guided LITT therapy in 4 patients (5 lesions) with recurrent glioblastoma. This study reported a progression-free survival and mean overall survival of 37 days and 10.5 months, respectively. Of note, 2 patients had local recurrence and another 2 had distant recurrence following LITT.
The first human phase I multicenter study evaluating the safety and efficacy of escalating doses of thermal energy using LITT in patients with recurrent glioblastoma was published in 2013. Of 11 patients enrolled in this study at 2 centers (Cleveland Clinic and University Hospital Case Western Reserve Medical Center), 10 underwent LITT and were followed up for a minimum of 6 months or until death. Median overall survival and progression-free survival of 316 days and greater than or equal to 30% at 6 months, respectively, were reported. Median overall survival of 225 days, 198 days, and 434 days following LITT was reported in patients treated using yellow TDT lines, blue TDT lines, and white TDT lines, respectively.
Hawasli and colleagues reported the clinical outcomes in 4 patients who underwent LITT for recurrent glioblastoma. Three of these 4 patients underwent standard treatment (surgery followed by concurrent chemoradiotherapy) and 1 patient had radiation with chemotherapy only. Progression-free survival was reported to be 9.2 months in 1 patient, who underwent a second LITT procedure for recurrence; 8.4 months in the second patient, who had distant recurrence; 7.6 months in the third patient, who was reported to have satellite lesion at recurrence; whereas no recurrence was noted in the fourth patient at 3.2 months of follow-up.
Recently Mohammadi and colleagues investigated the utility of LITT in 24 patients with glioblastoma (out of 34 patients with high-grade gliomas) in difficult-to-access areas in a multicenter retrospective study. Eighteen patients underwent LITT procedures for recurrent high-grade gliomas, whereas 16 patients underwent LITT as an upfront procedure for these tumors; however, the number of patients who underwent an upfront or salvage LITT procedure was not specified in this study. Ninety-one percent of the median tumor volume was covered by blue line and 3 cm was the maximum diameter of tumor treated. Median progressive-free survival ranged between 4.6 months and 9.7 months, based on tumor coverage by TDT lines. Clinical outcome based on upfront or salvage therapy for glioblastoma specifically was not mentioned in this study.
Based on these studies, median overall survival following LITT ranges between 6.9 months and 14.5 months in patients with recurrent glioblastoma compared with between 4 and 6 months in the literature. This difference in overall survival may not be attributable to LITT alone and the role of adjunctive therapies cannot be underestimated. However, LITT offers a minimally invasive approach to achieve tumor cytoreduction coupled with possible early opening of the BBB (based on animal studies ) for adjunct chemotherapies to be effective.
Laser Interstitial Thermal Therapy for Newly Diagnosed or De-novo Glioblastoma
Extent of tumor resection is a strong predictor of overall survival and also has an impact on quality of life in patients with newly diagnosed glioblastoma. There is a subset of patients with newly diagnosed glioblastoma who are either not suitable to undergo traditional open surgical resections because of their associated medical comorbidities, or deeper or eloquent location of the tumor (thalamus basal ganglia, insula), or who choose not to undergo open surgery. In such circumstances, LITT can be a useful alternative treatment modality to achieve adequate cytoreduction and facilitate adjunct treatment. However, in contrast with LITT for recurrent glioblastoma, efficacy of LITT is less clearly documented in the literature because it is a much newer procedure.
A report documenting the efficacy of LITT in a patient with newly diagnosed high-grade tumor was published in 1992. In 2002, Leonardi and colleagues reported on a 75-year-old patient with deep-seated bifocal glioblastoma, who was treated upfront with LITT, in their series of 6 patients with glioblastoma. However, clinical outcome in patients with newly diagnosed or recurrent disease following LITT was not stratified. Similarly, Mohammadi and colleagues, in their series of 34 patients with deep-seated high-grade gliomas (24 patients with glioblastoma) who underwent either upfront or salvage LITT, did not report the outcome data pertaining specifically to patients with glioblastoma. Jethwa and colleagues reported the utility of LITT in 3 patients with newly diagnosed glioblastoma. Two of these patients were considered high risk by a multidisciplinary oncology team in terms of an open surgical procedure and a third patient had a deep-seated tumor in the left temporal region. One patient with a tumor in the right frontal region required an additional treatment after initial LITT and another patient with tumor in the right frontal region required 2 trajectories (the other 2 had 1 trajectory each). Clinical outcome data were not reported.
Hawasli and colleagues reported the efficacy of LITT in 6 patients (thalamus, n = 4; basal ganglia, n = 1; corpus callosum, n = 1). No recurrence was noted in 4 patients at a mean follow-up of 0.2 months, 10.7 months, 0.1 months, and 0.9 months following LITT with maintenance of good functional status in surviving patients. Progression-free survival was reported to be 2.6 months and 3.2 months following LITT in 2 patients with recurrence. One patient died of fatal CNS infection 0.1 month following LITT and another patient died because of progressive CNS disease at 4.1 months following LITT. Three patients had no disease progression at follow-up of 10.7 months, 0.1 months, and 0.9 months following LITT. Further clinical studies focusing on the efficacy of LITT in patients with newly diagnosed glioblastoma are needed to validate this treatment modality.
Technical Nuances
At present, NeuroBlate System (Monteris Medical Inc, Winnipeg, Canada) and Visualase (Medtronic Inc, Minnesota, MN) are the two commercially available systems for LITT in patients with brain tumors in the United States. At our center we use the NeuroBlate System for laser ablation. The procedure is typically performed under general anesthesia in an intraoperative MRI suite (IMRIS, Winnipeg, Canada). Following anesthesia induction, the patient’s head is placed and secured in an operative position using a DORO head clamp (pro med instruments GmbH, Freiburg, Germany) and MRI scans (magnetization-prepared rapid acquisition gradient-echo with contrast and/or T2 volume) are obtained using scalp-applied fiducials. These images are then fused with preoperative MRI planning sequences for registration purposes. The patient’s head location is then registered with image space using the iPlan Curve navigation system (Brainlab AG, Munich, Germany). The site of skin incision is determined using the Varioguide system (Brainlab AG, Munich, Germany) and the bone is drilled with a twist drill. Stereotactic placement of an anchoring head bolt (4.5-mm outer diameter) is then performed using image guidance. A laser probe (either side firing or diffuse tip) is then inserted through the head bolt and connected to the robotic probe driver. M•Vision software is then used for planning and treatment at a workstation. This software integrates with the intraoperative MRI suite and uses a pulsed diode laser (Nd:YAG; range, 1046 nm; output, 12 W) to ablate the pathologic tissue. Gaseous CO 2 is used to cool the tip of the fiberoptic probe to avoid tissue vaporization as well as to enhance conformal treatment contours. Laser ablation is monitored in real time using MR thermometry and TDT lines are generated on the M•Vision workstation, which can be viewed throughout the procedure. Once satisfactory tumor ablation is achieved (tumor covered by blue TDT line [43°C for at least 10 minutes]), the procedure is concluded by explanting the bolts and suturing the skin incision. Multiple and larger lesions (up to 40–50 cm 3 in volume) can be treated in a single setting. However, for larger tumors, multiple trajectories are required and consideration must be given to the ability to safely accommodate postablation edema.
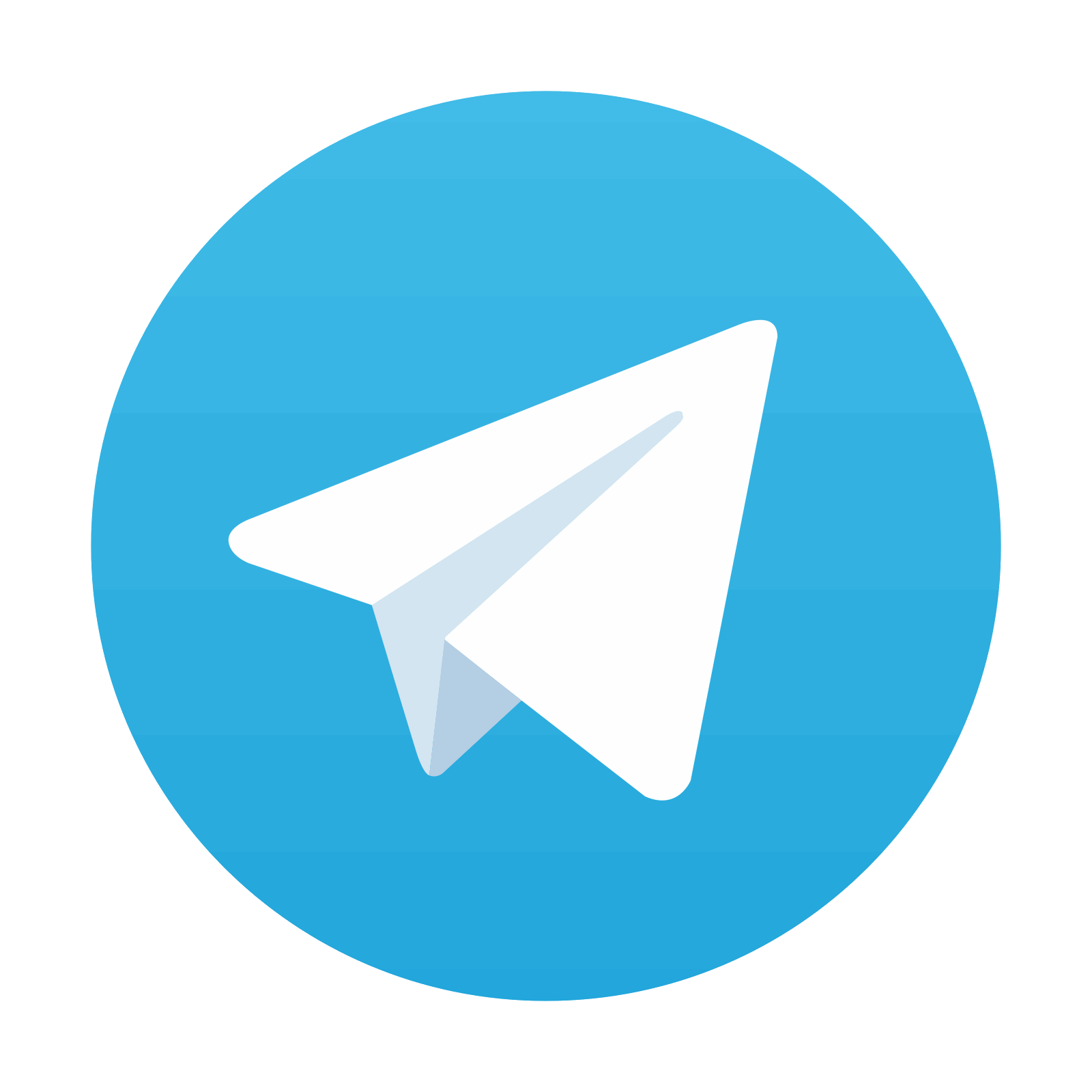
Stay updated, free articles. Join our Telegram channel

Full access? Get Clinical Tree
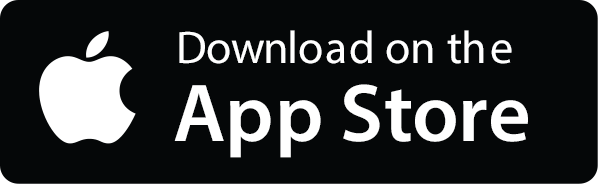
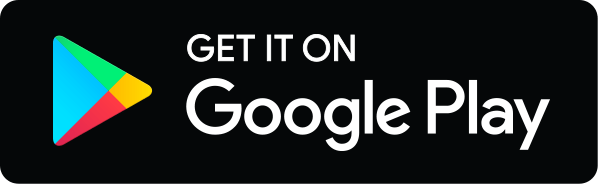