Models of Psychopathology in Epilepsy: Lessons Learned from Animal Studies
Thomas J. Hoeppner
Michael C. Smith
Recent developments in animal studies on the interface between epilepsy and psychopathology provide a better understanding of each of these clinical disorders and an appreciation of the underlying factors that contribute to the varieties of comorbidities that have been observed. Further, they provide the basis for more specific therapies for each of the subtypes of epilepsy and psychopathology and may permit the development of therapies that simultaneously address a combined manifestation of epilepsy and psychopathology. New developments are identified in each area. They reflect the broad introduction of mouse models and new genetic information most strongly (1,2,3). This review will focus on the animal models of depression/anxiety and their relationship to models of epilepsy. There is an increased appreciation of the limitations of many of the animal models that represent a single symptom of a clinical syndrome and of models that have been developed around a pharmacogenetic hypothesis. Therefore, several models of depression have been developed on the basis of the presumed mechanism of action of clinically effective antidepressants, such as enhancement of serotonin neurotransmission. Yet, the delayed clinical efficacy of antidepressants is not consistently addressed in the animal models. One of the most interesting new observations is the demonstration in rats that kainate-induced seizures in early life lead to the development of several depression-like symptoms and these can be prevented by exposure to an enriched environment after kainate administration (4,5).
There have been major new publications on animal models of depression (1,2,3) and models of seizures and epilepsy (6). While these focus on the methodology of each of the individual animal models of psychiatric disorders, seizures, or epilepsy, there is explicit recognition that seizures may have behavioral or cognitive effects (7,8). There is an asymmetry in this interest. Animal models of psychopathology are rarely evaluated for epileptogenic tendencies.
Many patients with epilepsy show cognitive, memory, or affective abnormalities even when they are not having seizures and these abnormalities occur more frequently
than would be expected by chance (9,10,11,12). Several factors may contribute to the association between epilepsy and behavioral abnormalities. One condition may be the etiology of the other or they may both have a common source.
than would be expected by chance (9,10,11,12). Several factors may contribute to the association between epilepsy and behavioral abnormalities. One condition may be the etiology of the other or they may both have a common source.
The three possible relationships are that the epilepsy leads to the psychopathology, the psychopathology leads to the epilepsy or that another process is responsible for both.
The first possibility is that the presence of epilepsy might lead to the development of behavioral abnormalities. For instance, the behavioral abnormality might be (a) a reaction to the social stigma of having epilepsy (13); (b) due to the presence of any chronic, intractable disease (12); (c) a consequence of epileptic activity itself; or (d) a consequence of the treatment of epilepsy (medical or surgical).
The second possibility is that the presence of certain behavioral abnormalities might increase the likelihood of developing epilepsy. For instance, hyperactivity, impulsive behavior, and other socially maladaptive behaviors might increase the likelihood of head trauma or drug and alcohol addiction with a concomitant increase in posttraumatic epilepsy or other forms of epilepsy. In addition, the treatment of psychiatric disease, by modulation of neuronal circuits, may alter the excitability of critical neuronal circuits, increasing the likelihood of developing epilepsy.
The third possibility is that the presence of epilepsy and of a behavioral abnormality may be due to a common pathophysiological process. For instance, an insult to the brain that caused the epilepsy may also cause a behavioral abnormality, or there may be a common genetic predisposition for both epilepsy and certain behavioral abnormalities due to abnormal production of a type of γ-aminobutyric acid (GABA) receptor or other neurotransmission factor.
This chapter addresses the thesis that experimental animal studies provide novel information about psychopathology in epilepsy and that new therapies may emerge from a consideration of animal models.
Background to the Use of Animal Models in Studying Normal Function, Epilepsy, and Psychopathology
Animal studies have provided an extensive understanding of anatomical, physiological, pharmacological, and behavioral processes and, in particular, the fundamental mechanisms of normal neuronal excitability and the neuronal elements of emotional behavior. It is upon this substrate that pathological alterations and therapeutic interventions occur. The basic mechanisms involved in membrane potential and action potential generation and synaptic transmission form the foundation of neuronal physiology. Disturbance of these cellular mechanisms within particular anatomical circuits results in the diverse panoply of neurological and psychiatric diseases (14,15). Systematic exploration and controlled manipulation of these basic physiological and anatomical mechanisms can only be accomplished in animals. We will outline the normal physiological elements and identify the contribution they make in understanding the nature of epilepsy and behavioral disturbances and their treatment. Subsequently, we will examine the application of specific animal models of epilepsy and psychopathology.
The animal models may occasionally appear to be very poor representations of human disease and the promises offered by the animal models may often exceed their yield (16), but in several instances they have provided a basis for new therapies, an understanding of the mechanism of action of established therapies, an understanding of disease etiologies, or a description of diseases that have not yet been appreciated in humans.
Physiological Processes of Neuronal Excitability
Action Potential Mechanisms
The distinct function of voltage-gated sodium and potassium channels in a semipermeable membrane described in the squid giant
axon provide our understanding of the ionic and molecular basis of action potential generation (17). These macromolecular complexes have been well characterized, and are the therapeutic targets for some of the most widely used anticonvulsants, including phenytoin, carbamazepine, valproate (VPA), and lamotrigine. These antiseizure agents block sustained repetitive firing of isolated neurons, leaving low-frequency action potentials relatively unaffected (18). Such an action provides symptomatic treatment of seizures, which involve high-frequency discharges, while leaving the lower-frequency discharges involved in normal behaviors relatively unaffected. In contrast, the puffer fish poison, tetrodotoxin, blocks the voltage-gated sodium channel preventing the increase in sodium permeability associated with the rising phase of the action potential, altering both normal and pathological neuronal firing (19). Differentiating the action of an agent on repetitive firing from the action of a direct sodium channel blocker permits the development of less toxic anticonvulsants. Some of the anticonvulsants directed at action potential mechanisms have proved to be very effective in treating refractory pain (carbamazepine, phenytoin, topiramate, zonisamide), although it is not certain that this is the principal mechanism responsible for the benefit.
axon provide our understanding of the ionic and molecular basis of action potential generation (17). These macromolecular complexes have been well characterized, and are the therapeutic targets for some of the most widely used anticonvulsants, including phenytoin, carbamazepine, valproate (VPA), and lamotrigine. These antiseizure agents block sustained repetitive firing of isolated neurons, leaving low-frequency action potentials relatively unaffected (18). Such an action provides symptomatic treatment of seizures, which involve high-frequency discharges, while leaving the lower-frequency discharges involved in normal behaviors relatively unaffected. In contrast, the puffer fish poison, tetrodotoxin, blocks the voltage-gated sodium channel preventing the increase in sodium permeability associated with the rising phase of the action potential, altering both normal and pathological neuronal firing (19). Differentiating the action of an agent on repetitive firing from the action of a direct sodium channel blocker permits the development of less toxic anticonvulsants. Some of the anticonvulsants directed at action potential mechanisms have proved to be very effective in treating refractory pain (carbamazepine, phenytoin, topiramate, zonisamide), although it is not certain that this is the principal mechanism responsible for the benefit.
Synaptic Transmission
Presynaptic Mechanisms
Synaptic transmission depends on neurotransmitter synthesis, packaging in vesicles, calcium-regulated docking and release at presynaptic release sites, diffusion across the synaptic cleft, and subsequent binding at a postsynaptic receptor. Each of these processes provides a target site for disease and a target site for therapy.
Postsynaptic Mechanisms
The macromolecular postsynaptic receptors are neurotransmitter specific, but the receptors for a given neurotransmitter show remarkable diversity in affinity for the neurotransmitter and other ligands (20,21).
Each neurotransmitter has a family of receptors to which it will bind. The family is composed of various combinations of discrete subunits. The combination of subunits results in not only neurotransmitter specificity but also diversity in affinity for the neurotransmitter and for other ligands. The postsynaptic receptor may have an associated ionophore or a metabotropic effect through a second messenger.
The neurotransmitter receptors commonly show developmental changes in their expression. The developmental changes in particular neurotransmitter systems may underlie the high incidence of seizures in early life (22).
Neurotransmitter receptor subtypes also show distinct cellular and regional distributions. The diversity, regulation of number, and cellular and regional location of a given neurotransmitter receptor subtype determine its role in neuronal excitability.
Reuptake and/or degradation of neurotransmitters are dependent on specific reuptake pumps, autoreceptors, and enzymes.
The discussion of neurotransmission as in the preceding text applies particularly to the small molecule “traditional” neurotransmitters and neuropeptides. The participation of gas neurotransmitters in these processes expands the set of regulatory mechanisms that may be involved in neuronal excitability and emotional behavior.
Pharmacological Treatment of Epilepsy and Psychiatric Disorders Directed Extensively at Synaptic Mechanisms Based on In vitro Animal Models
Antiepileptic Drugs Affecting Synaptic Transmission
Many anticonvulsants are characterized according to the type of synaptic mechanism they are directed toward (23,24).
Utilizing animal models, it has been demonstrated that many currently available
anticonvulsants have a mechanism of action that enhances neurotransmission that utilizes GABA, the primary inhibitory neurotransmitter in the central nervous system. These include phenobarbital, diazepam, vigabatrin, and tiagabine. Gabapentin was developed as an anticonvulsant on the basis of its structural similarity to GABA; however, it does not appear to act at the GABA receptor or at any site related to GABA, but rather acts at a subunit of presynaptic P/Q-type voltage-sensitive calcium channels, where it inhibits calcium influx in noradrenergic terminals. Gabapentin and the structurally related pregabalin are both anticonvulsant and effective in blocking neuropathic pain (25). Blocking neurotransmission that utilizes glutamate, the primary excitatory neurotransmitter in the central nervous system, appears to be an important function of felbamate, remacemide, and topiramate (23).
anticonvulsants have a mechanism of action that enhances neurotransmission that utilizes GABA, the primary inhibitory neurotransmitter in the central nervous system. These include phenobarbital, diazepam, vigabatrin, and tiagabine. Gabapentin was developed as an anticonvulsant on the basis of its structural similarity to GABA; however, it does not appear to act at the GABA receptor or at any site related to GABA, but rather acts at a subunit of presynaptic P/Q-type voltage-sensitive calcium channels, where it inhibits calcium influx in noradrenergic terminals. Gabapentin and the structurally related pregabalin are both anticonvulsant and effective in blocking neuropathic pain (25). Blocking neurotransmission that utilizes glutamate, the primary excitatory neurotransmitter in the central nervous system, appears to be an important function of felbamate, remacemide, and topiramate (23).
On the basis of this experience, one can design an anticonvulsant based on its ability to enhance GABA neurotransmission or to block glutamate neurotransmission. Although this approach does not guarantee a useful antiepileptogenic drug for clinical purposes, it provides a rational approach to the development of antiepileptic drugs (AEDs). It is generally predictable that agents that act on ubiquitous neurotransmitter systems such as GABA and glutamate will alter the occurrence of seizures, but they are also likely to influence other behaviors (including emotional expression) that utilize these neurotransmitter systems. Therefore, barbiturates, which enhance GABA neurotransmission, are also potent sedatives. It seems likely that the use of ligands that are more selective in their action, such as those that act at a specific subtype of a neurotransmitter receptor, which has a restricted distribution, will in general have reduced side effects. Ketter et al. (26) propose that better psychiatric outcomes in patients with epilepsy may be obtained by the judicious use of AEDs that have mechanisms of action that are “sedating” or “activating.” Therefore, they propose utilization of the psychotropic action of AEDs for positive benefit. They suggest that the sedating (primarily GABA agonists) AEDs, such as barbiturates, benzodiazepines, VPA, gabapentin, tiagabine, and vigabatrin might be beneficial in patients with activated baselines involving insomnia, agitation, anxiety, racing thoughts, and weight loss, while activating (primarily glutamate antagonists) AEDs such as felbamate and lamotrigine might be helpful in patients with sedated baselines involving hypersomnia, fatigue, apathy, depression, sluggish cognition, and weight gain. Of further relevance to psychiatry is the observation that barbiturates may alter the metabolism of tricyclic antidepressants, resulting in the loss of the antidepressant effect (27).
Ethosuximide (ESM) reduces the low-threshold calcium current (through type T channels) in thalamic neurons and this seems to be important in the control of absence seizures (28,29).
The low neurotoxicity of ESM may be attributable in part to a more selective mechanism and anatomical distribution of its site of action in the nervous system compared with most other AEDs.
Antipsychotic Drugs Affecting Synaptic Transmission
Many drugs used in the treatment of psychiatric conditions are defined on the basis of the neurotransmitter action they alter in animal models (30).
Neuroleptics generally antagonize dopamine-mediated neurotransmission, particularly at D2 receptors, and this is thought to be important to their efficacy (31). The D2 receptors are particularly dense in the basal ganglia and in mesocortical and mesolimbic structures. The dopamine hypothesis of schizophrenia is based on the observation that the potency of antipsychotic drugs directly correlates with their affinity for the dopamine receptor (32). In this view, schizophrenia is attributable to an aberration in dopamine neurotransmission. Combined studies using patients with schizophrenia and animal models suggest that a balance between transmission at D1 and D2 receptors and a balance between
transmission at D2 and glutamate receptors may be important in the pathophysiology of schizophrenia (32,33).
transmission at D2 and glutamate receptors may be important in the pathophysiology of schizophrenia (32,33).
Antidepressants generally increase the availability of monoamines by blocking their reuptake or inhibiting their metabolism (30,34).
Tricyclic antidepressants inhibit reuptake of norepinephrine and serotonin into nerve endings. They reduce the synthesis and release of norepinephrine and decrease the firing of neurons in the locus coeruleus of the rat. They tend to increase the likelihood of seizures.
Monoamine oxidase inhibitors prevent the enzymatic deamination of norepinephrine, serotonin, and dopamine to varying extents. They also interfere with the metabolism of barbiturates and many other drugs that might alter seizure control (35).
Selective serotonin reuptake inhibitors (SSRIs) act at presynaptic autoreceptors of serotonin releasing neurons and are effective in the treatment of depression (34). Some SSRIs, but not all, can lower the seizure threshold (36).
Anxiety was often treated in the past with barbiturates, but it is now more commonly treated with benzodiazepines (30). The efficacy of benzodiazepines in relief of anxiety has been demonstrated in a conflict punishment model in animals (37). Both benzodiazepines and barbiturates enhance GABA neurotransmission at the postsynaptic macromolecular complex. There are specific binding sites for benzodiazepines associated with GABA receptors and a separate binding site for barbiturates on the GABA macromolecular complex (21).
Note that both benzodiazepines and barbiturates are potent anticonvulsants in both animal models and patients with epilepsy.
The fact that some AEDs and antipsychotic drugs act on the same neurotransmitter systems and that some of the manifestations of the diseases are induced by these treatments (see subsequent text) in animal models provides a clear basis for interaction between the treatment of one condition and induction of the other.
Animal Models: General Considerations
Animal models of human behavior and disease serve different purposes. They may be used to provide insight and understanding of normal function or a disease process. The use of animal models for testing the safety and efficacy of therapeutic approaches is standard practice. The animal models minimize or eliminate placebo effects, allow the rapid screening of large numbers of compounds in a short period of time, and minimize cost. The models may also give insight in to the mechanisms involved in pathophysiology (producing epilepsy or behavioral abnormalities). Note that the models that have been developed reflect a collective understanding of the disease and the attempt to control some of the abnormal features. The models are generally considered to be useful if they are predictive of a response to therapy in the clinical condition (predictive validity), even if they lack many of the features of the clinical disorder. This frequently leads to animal models of a symptom and consequently to symptomatic therapies. Such approaches may not influence the underlying etiologic processes. Therefore, sodium channel blockers are effective in preventing seizures induced by maximal electroshock (MES) and provide symptomatic therapy in epilepsy. Epilepsy is a chronic disease in which recurrent seizures occur. A seizure is defined as a stereotyped, involuntary paroxysmal alteration of behavior (based on an abnormal synchrony of neuronal discharge). While epilepsy is a chronic disease and may be manifest in a variety of syndromes, many of the animal models are acute seizure models. This may be beneficial for certain therapeutic purposes, but will tend to mask or ignore interictal features. Chronic models may be necessary to study the process of epileptogenesis, the interictal state, and the long-term consequences of repeated seizures (38,39).
Epilepsy Models
There are almost a hundred different animal models of seizures and epilepsy (6,40,41,42,20).
The models utilize chemical/pharmacological convulsants (alumina cream, cobalt, tungstic acid, iron, pentylenetetrazol [PTZ], penicillin, picrotoxin, strychnine, potassium, ouabain, kainate, pilocarpine, insulin, alcohol withdrawal), electrical stimulation (MES, electrically induced status epilepticus, kindling), sensory stimulation (audiogenic, photogenic seizures), genetic models (genetically epilepsy prone rat, tottering mouse, Papio papio, Genetic Absence Epilepsy Rats from Strasbourg [GAERS]), freezing, and physical trauma. A comprehensive volume Models of Seizures and Epilepsy (6) has been recently published. This volume provides a technical manual for laboratory workers, identifying how each model can be generated and its characteristics (electrical, behavioral, histopathology, ontogenic, pharmacological reactivity), and it also provides insights into human epileptic disorders developed through the use of each model.
The models utilize chemical/pharmacological convulsants (alumina cream, cobalt, tungstic acid, iron, pentylenetetrazol [PTZ], penicillin, picrotoxin, strychnine, potassium, ouabain, kainate, pilocarpine, insulin, alcohol withdrawal), electrical stimulation (MES, electrically induced status epilepticus, kindling), sensory stimulation (audiogenic, photogenic seizures), genetic models (genetically epilepsy prone rat, tottering mouse, Papio papio, Genetic Absence Epilepsy Rats from Strasbourg [GAERS]), freezing, and physical trauma. A comprehensive volume Models of Seizures and Epilepsy (6) has been recently published. This volume provides a technical manual for laboratory workers, identifying how each model can be generated and its characteristics (electrical, behavioral, histopathology, ontogenic, pharmacological reactivity), and it also provides insights into human epileptic disorders developed through the use of each model.
Several animal models incorporate the growing appreciation of the genetic factors influencing neuronal activity and the role of developmental events in the emergence of seizure disorders.
Selective models will be discussed for their impact on our understanding of epilepsy, their role in therapeutics, or their relationship with psychological processes. These are among the most widely utilized. The models of epilepsy share a common marker for the occurrence of seizures: the electrical manifestations of neuronal hyperexcitability and hypersynchrony—the paroxysmal depolarization shift at the cellular level, epileptic spikes, and/or electrographic seizures in the electroencephalogram (41)—and some of them show behavioral seizures. There is no single marker for psychopathology that is as readily available and accepted as is electrical activity for a seizure. Animal models of psychopathology rely primarily on behavioral measures of function. Animal models of epilepsy reflect the major categories of seizures: generalized seizures (absence, myoclonic, and tonic-clonic) and partial seizures (simple, complex, and partial seizures with secondary generalization). The availability of electrographic discharges, as a marker for epileptic activity, enables the use of in vitro preparations for the study of seizures. The use of tissue slices or dissociated neurons derived from several different brain regions in diverse species has contributed enormously to our understanding of the mechanisms of action of anticonvulsants and convulsants (18,28,29,41). While there are several in vitro animal models, this discussion will focus on in vivo models to facilitate consideration of focal neuroanatomical features and behavioral manifestations.
Models of Generalized Tonic-Clonic Seizures
The MES model involves electrical activation of generalized seizures in normal animals (44). Stimulation through the intact skull (electrodes may be placed at various sites, but most commonly they are applied to the corneas) results in a high-frequency generalized epileptic discharge. In the rat and the mouse, MES produces tonic bilateral limb flexion and extension followed by clonus. Merritt and Putnam (45,46) successfully applied a variant of this model, in 1937, in the search for a nonsedating structural relative of phenobarbital with anticonvulsant properties. Their demonstration of the suppression of electroshock-induced convulsions in cats by diphenylhydantoin (phenytoin) was a landmark in the application of experimental animal techniques to clinical neuroscience questions. It resulted from a systematic screening of compounds in an animal model of epilepsy. Within a year of the discovery of its efficacy in suppressing electroshock-induced seizures in animals, phenytoin was introduced on a large scale for use in humans for the symptomatic treatment of epilepsy (47). The studies of Merritt and Putnam firmly established the validity of an animal model of epilepsy as a test for drug efficacy with important clinical significance. They combined behavioral (nonsedating), pharmacological (structural analog of phenobarbital), and therapeutic (anticonvulsant) criteria simultaneously. The discovery of phenytoin fulfilled
the need for minimizing an undesirable behavioral effect of phenobarbital (sedation). A comparable approach aimed at reducing the psychiatric manifestations associated with epilepsy might be profitable.
the need for minimizing an undesirable behavioral effect of phenobarbital (sedation). A comparable approach aimed at reducing the psychiatric manifestations associated with epilepsy might be profitable.
The MES model is recognized as a good predictor of efficacy against generalized tonic-clonic seizures. Drugs that are effective in blocking tonic hindlimb extension in this model are generally effective against partial and generalized tonic-clonic seizures in humans (48). However, levetiracetam, a new anticonvulsant, does not block MES seizures, but is an effective treatment for complex partial seizures in humans (49) The seizures produced by MES involve widespread, bilateral neural structures, but the tonic hindleg extension appears to be dependent on activation of brainstem structures (50). MES is an acute seizure model, and while it has been widely used for testing potential AEDs, it is not promoted as a model to study behavioral alterations or other interictal effects to any extent. Note, however, that electroconvulsive shock is widely utilized as the treatment of choice for severe clinical depression (51).
Models of Generalized Absence Seizures
Systemic administration of PTZ provides an animal model of absence seizures. The model mimics the bilaterally synchronous spike and wave discharge characteristic of absence seizures. There is an associated arrest of behavior. At high doses, this may be followed by myoclonic jerks and may lead to generalized tonic-clonic seizures. PTZ-induced seizures are blocked by ESM and VPA. PTZ blocks GABA-mediated inhibitory postsynaptic transmission (52). PTZ-induced seizures have been shown to be a good predictor of efficacy against absence seizures (48). High-dose systemic penicillin (another GABA inhibitor) also provides an animal model of absence seizures. Drugs that are effective against PTZ seizures, such as ESM and trimethadione, are not very effective against MES seizures. Some drugs that are effective against MES-induced seizures, such as carbamazepine and phenytoin, are not very effective against PTZ-induced seizures and may actually worsen them (48,53). This double dissociation suggests different mechanisms for the seizure models and different mechanisms of action for the anticonvulsants. As indicated above, ESM preferentially blocks the low-threshold calcium current in thalamic neurons and is very effective against the PTZ animal model and human absence seizures (28). In vitro animal models of absence seizures suggest a critical involvement of thalamocortical circuitry, with a low-threshold calcium current of neurons in the thalamic reticular nucleus providing a pacemaker function for the typical spike and wave discharges (54,55,56).
The GAERS are an inbred strain that display many of the characteristics of human absence epilepsy (57,58). They provide an excellent predictive model of the effect of AEDs on spike and wave discharges in humans with typical absence epilepsy, with the exception of lamotrigine, which suppresses spike and wave discharges in humans, but not in the rat absence model (59). It is of interest that haloperidol, a dopamine antagonist effective in treating psychosis, increases the spike and wave discharges in this animal model. The occurrence of spontaneous spike and wave discharges and behavioral arrest in the GAERS offers the opportunity to evaluate interictal behavioral alteration in a chronic model of human epilepsy in which there is generally considered to be little or no cognitive or emotional disturbance.
Models of Simple Partial Seizures
Focal application of aluminum hydroxide to the cortex results in a delayed appearance of epileptic spikes, and electrographic and behavioral seizures (60,61). The behavioral and electrical characteristics of the seizures reflect the site of application of the alumina. Intracortical alumina provides a chronic model of epilepsy that manifests spontaneous recurring seizures and is considered by some as the best validated model of epilepsy in regard to the behavioral and electrographic manifestations of seizures, the temporal and spatial distribution of the seizures, and the response to anticonvulsants
that parallels the response of patients with focal epilepsy (41). Decreases in GABA concentrations have been observed around the focus in the alumina model (62). This model would allow for examination of the interictal behavioral concomitants of recurrent seizures, but it appears that its use has been limited owing to the variability of seizure occurrence (a feature in common with human epilepsy), and the cost of the primate model. Lockard (63) showed that social stresses precipitate seizures in the alumina cream monkey model. The model is not as readily developed for rodents (personal observation) as for monkeys.
that parallels the response of patients with focal epilepsy (41). Decreases in GABA concentrations have been observed around the focus in the alumina model (62). This model would allow for examination of the interictal behavioral concomitants of recurrent seizures, but it appears that its use has been limited owing to the variability of seizure occurrence (a feature in common with human epilepsy), and the cost of the primate model. Lockard (63) showed that social stresses precipitate seizures in the alumina cream monkey model. The model is not as readily developed for rodents (personal observation) as for monkeys.
Recurrent focal partial seizures are also modeled by intracortical application of the metals cobalt, tungsten, zinc or iron (6,41). These models are effective in rodents and could be applied to the investigation of interictal behavioral alterations. In contrast to the other models, the use of iron as an epileptogenic agent mimics the availability of a naturally occurring blood component (from hemoglobin) associated with head trauma (64).
Electrical kindling can also produce a model of simple partial seizures, but it is discussed in the following text as a model of complex partial seizures. The difference reflects the site of stimulus application. Stimulation in the motor cortex produces a model of simple partial seizures, whereas stimulation in the amygdala or hippocampus produces a model of complex partial seizures.
Models of Complex Partial Seizures
Kainate
Kainate (administered systemically or intrahippocampal) produces epileptic seizures originating in the hippocampus. The kainic acid binds to glutamate receptors and produces widespread death of neurons, most prominent in the hippocampus (65). This model is particularly useful for evaluating excitotoxic processes and the consequences of hippocampal cell loss and the resultant neuronal circuitry reorganization. In some regard, this parallels the pathological changes of hippocampal sclerosis seen in patients with the syndrome of mesial temporal lobe epilepsy (66). The model has three major stages: (a) an initial episode of status epilepticus lasting hours, (b) a seizure-free latent period lasting weeks, and (c) a chronic period of spontaneously recurring seizures. There are important strain and age differences affecting the susceptibility to the model (67). In some mouse strains, kainate induces status epilepticus, but neither cell loss nor delayed spontaneous seizures occur (68). Immature and aged rats differ from young adults treated with kainate. Immature rats treated with kainate may have severe status epilepticus, but minimal cell loss, while aged rats rarely survive the status epilepticus. Variability of the kainate model can be problematic, with substantial mortality in some animals, inadequate epileptiform activity in others, and variable timing of the occurrence of spontaneous seizures. Note, however, that this variability mirrors some of the variability seen in patients with complex partial seizures (69).
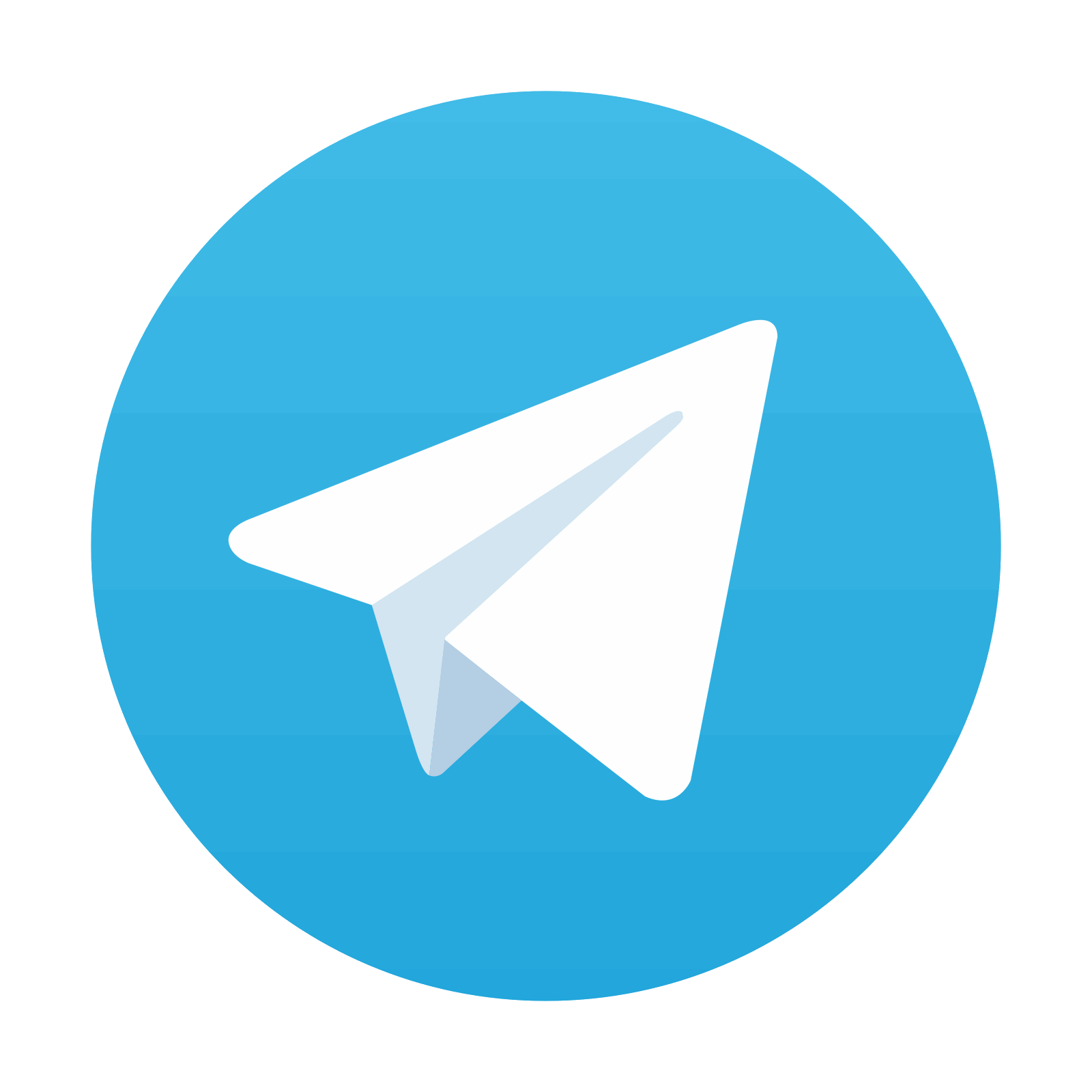
Stay updated, free articles. Join our Telegram channel

Full access? Get Clinical Tree
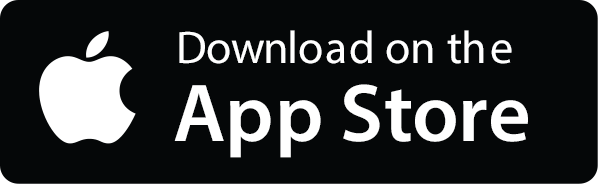
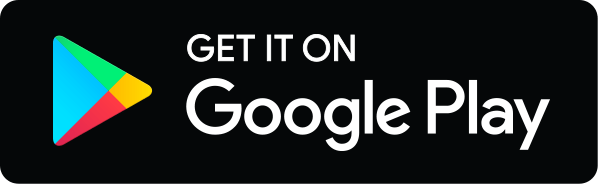
