Traumatic brain injury (TBI) is a serious disorder that is all too common. TBI ranges in severity from mild concussion to a severe life-threatening state. Across this spectrum, rational therapeutic approaches exist. Early identification that TBI has occurred in a patient is paramount to optimal outcome. Proper clinical management should be instituted as soon as possible by appropriately trained medical providers. More seriously injured patients must be triaged to advanced care centers. It is only through this rational approach to TBI that patients may expect to achieve optimal clinical and functional outcome.
Key points
- •
Traumatic brain injury (TBI) is a treatable condition.
- •
Early diagnosis of TBI is critical.
- •
Clinical practice guidelines (CPGs) are available to help clinical management across the spectrum of TBI severity.
- •
Optimal outcome depends on proper care beginning prehospital and continuing in an advanced clinical care setting by appropriately trained medical providers.
Introduction
TBI is pervasive and is a leading cause of death and disability, particularly in young people. Despite widespread use of preventative devices, such as helmets, seatbelts, and airbags, moderate to severe TBI disables 80,000 persons per year. A significant percentage of these patients require intensive neurologic management, including neurosurgery. Several CPGs have been written to help guide physicians in managing these complex patients. The most widely used are the Brain Trauma Foundation CPGs. Despite lack of evidence from randomized clinical trials (RCTs) to support every recommendation, CPGs provide a rational approach for treating this complicated group of patients. Moreover, there is emerging evidence that suggests improved outcomes when these CPGs are followed and specialized neurocritical care teams are providing the primary clinical care.
Introduction
TBI is pervasive and is a leading cause of death and disability, particularly in young people. Despite widespread use of preventative devices, such as helmets, seatbelts, and airbags, moderate to severe TBI disables 80,000 persons per year. A significant percentage of these patients require intensive neurologic management, including neurosurgery. Several CPGs have been written to help guide physicians in managing these complex patients. The most widely used are the Brain Trauma Foundation CPGs. Despite lack of evidence from randomized clinical trials (RCTs) to support every recommendation, CPGs provide a rational approach for treating this complicated group of patients. Moreover, there is emerging evidence that suggests improved outcomes when these CPGs are followed and specialized neurocritical care teams are providing the primary clinical care.
Pathophysiology
TBI is understood to be the result of 2 phases: initial neuronal injury followed by secondary insults. Initial neuronal injury occurs immediately and is due to the inciting traumatic event, whereas the second or late phase occurs from multiple neuropathologic processes and evolves over a period of minutes to days.
Because the primary injury phase is immediate, it is not easily treatable. In the most severe cases, this phase of injury may be deadly. Direct impact of parenchymal tissue against the bony vault of the skull leads to both neuronal and vascular injuries. Lesions may be identified at both the side of collision as well as the opposite side, causing coup-contrecoup lesions. Moreover, rotational forces may lead to additional injuries, including diffuse axonal injury. Diffuse axonal injury is shearing of axons in cerebral white matter, causing neurologic deficits, such as encephalopathy. The consequences of this type of injury can be delayed by up to 12 hours after initial trauma. In cases of penetrating brain injury, skull fracture is present in addition to an injury tract. If the penetration is due to a high-velocity fragment, such as a bullet, there is superimposed tissue cavitation and possibly ricochet injury. Finally, diffuse microvascular damage occurs and is due to an early loss of cerebral vascular autoregulation and a loss of blood-brain barrier integrity.
Several types of bleeding complications are possible after TBI and often coexist, including subdural hematoma, epidural hematoma, intraparenchymal hemorrhage, contusion, and traumatic subarachnoid hemorrhage. Subdural hematoma is the most common reason for ICU admission for head injury and constitutes approximately 50% of all TBI admissions; epidural hematoma accounts for approximately 3%. Skull fracture, especially in the temporal or parietal region, is an important cause of epidural hematoma, usually in association with laceration of the middle meningeal artery.
Focal injuries occur at the site of impact, often identified by neuroimaging as contusion or hematoma. The orbitofrontal and anterior temporal lobes are most commonly affected. Neurologic deficits are typically (but not exclusively) referable to the functions of those areas. The development of delayed hematoma formation may take up to several days after the initial injury.
The delayed nature of secondary injuries allows for medical and surgical intervention and has become a major focus of TBI treatment. Injury at this level involves both neurons and glia. Many cellular processes are implicated in this phase, ultimately resulting in neuronal suicide. These mechanisms include hypoxia, ischemia, effects of free radicals, excitatory amino acids, certain ions (eg, calcium), ischemia, metabolic crisis, and inflammation.
Inflammation has proved a critical element of the secondary TBI process. Within the central nervous system, the inflammatory process is activated almost instantaneously and undergoes a series of events, including expression of adhesion molecules, cellular infiltration, and additional secretion of inflammatory molecules and growth factors, causing either regeneration or cell death. Most likely, this inflammatory cascade is both helpful and deleterious to an injured brain and thus remains a critical area for research.
Hypoxic and hypoperfusion injury is well recognized as a leading contributor to secondary brain injury. The injured brain is more susceptible to hypoxic-ischemic states, with changes in cerebral autoregulation exacerbating injury. Delayed neurologic deterioration is often caused by ischemic injury. Finally, posttraumatic vasospasm is thought a common occurrence and may worsen ischemic burden.
Despite the advances made in understanding mechanisms of TBI, no single specific treatment has been shown to halt or reverse the chain of events leading to neuronal death. Thus, the clinical emphasis in severe TBI treatment remains supportive based on an understanding of intracranial anatomy and pathophysiology.
Intracranial dynamics
The intracranial volume is relatively fixed and surrounded by an inelastic skull. The contents of the intracranial vault that contribute to intracranial pressure (ICP) are the brain (1300 mL), blood (110 mL), and cerebrospinal fluid (CSF) (65 mL). Normal ICP in healthy adults is approximately 10 mm Hg. The Monro-Kellie doctrine states that the intracranial contents are encased in a rigid skull and the components are relatively inelastic, so change in the volume of one component must be compensated for by reduction in the volume of another component of the system or ICP increases. Although this is a simplification of the complex pathophysiology involved, the Monro-Kellie doctrine provides a conceptual framework for understanding increased ICP causes and treatments in the context of TBI.
The brain parenchyma is made up largely of water, which is not compressible. CSF, which contributes 10% of the intracranial volume, is produced predominantly by the choroid plexus, with a small amount produced as interstitial fluid from brain capillaries. Approximately 10 mL/h to 20 mL/h of CSF is produced normally, and this amount is not significantly reduced by increased ICP. CSF is taken up into the cerebral venous sinuses and drained through the arachnoid villi. Intracranial blood volume, approximately 10% of the volume within the skull, is approximately two-thirds venous and one-third arterial. Flow of arterial blood is regulated largely by change in arteriolar caliber, which adjusts in response to systemic arterial pressure, P o 2 , and P co 2 . When brain injury occurs and space-occupying lesions develop, the body must adjust its volumes of blood, CSF, and brain to compensate. In states of poor compliance, however, a seemingly insignificant increase in intracranial volume can result in a dramatic increase in ICP. Ultimately, if ICP increases beyond mean arterial pressure (MAP), arterial blood is unable to enter the skull, and global ischemia and cerebral ensue.
Triage
When evaluating patients with known or suspected TBI, one must be able to effectively perform triage and assess quickly for proper initial management. Patients may be stratified as at low risk, moderate risk, or high risk for intracranial injury, using several criteria. Those with mild and nonprogressive headache and dizziness without loss of consciousness and normal neurologic examinations may be managed safely without neuroimaging. Close clinical monitoring with frequent neurologic examinations suffice. Under these conditions, it is imperative to withhold medications that may impair consciousness and responsiveness and thus interfere with clinical examinations. Examples of medications to avoid are central-acting analgesics and sedatives.
For patients with moderate risk to high risk for intracranial injury, CT head without contrast is recommended. This group of patients includes those with a history of a change in consciousness, alcohol or drug intoxication, posttraumatic seizure, vomiting, amnesia, or unreliable history. Moreover, these patients should be transferred to a hospital with neurocritical care and neurosurgical services.
Most commonly, TBI patients are classified according to severity scores, such as the Glasgow Coma Scale (GCS). Using this scale, a score of 13 to 15 is considered mild injury, 9 to 12 moderate, and 8 or less severe TBI. Although easy to use, the GCS is limited by its inability to take into account sedation, paralysis, intubation, or intoxication and may overestimate injury severity in these patients. A newer scoring system, the Full Outline of Unresponsiveness Score, was developed to improve on these problems. Unlike the GSC, the Full Outline of Unresponsiveness Score includes a brainstem examination and is better performed on intubated patients.
All TBI patients with persistent impairment of consciousness should be admitted to an ICU. A brief but thorough neurologic examination should be performed, with emphasis on level of arousal and orientation, GCS score, pupils and other cranial nerves, and basic language and motor function. Afterwards, these patients should promptly receive a noncontrast CT scan of the head. While reading the CT, special attention should be given for the presence of parenchymal abnormalities, such as contusion or edema, as well as skull or facial fractures. Contusions are often detected in areas of the brain that make contact with bony prominences, such as the frontal, temporal, and occipital poles. Additionally, small contusions may evolve into hemorrhages, which should be considered if clinical deterioration occurs because it might lead to herniation. A normal CT scan does not preclude a severe neurologic injury; if a patient’s neurologic examination is abnormal, it must be assumed that a severe brain injury has occurred until proved otherwise. Repeat imaging should be strongly considered with any neurologic changes.
Emergency department and neurocritical care unit management
Both neurologic and functional outcomes may be improved by first avoiding hypoxemia, hypercapnia, and hypovolemia. In severe TBI, advanced trauma life support and brain resuscitation for TBI principles merge. Hypoxia, defined as Pa o 2 less than 60 mm Hg, and hypotension, defined as systolic blood pressure less than 90 mm Hg, are common in general trauma and in TBI. Hypoxia and hypotension are present in 50% and 30% of severe TBI patients, respectively. Management must focus on correcting these problems immediately to optimize outcome.
All trauma patients with severe TBI should be placed in a rigid cervical collar. There are 2 reasons for this. The first is to protect the cervical spinal cord and column until it is ascertained that neither is injured. Between 2% and 6% of TBI patients who reach the emergency department have cervical spine fracture. Surprisingly, iatrogenic damage to the spinal cord is estimated as high as 25% of those suffering from combined TBI and cervical cord injury. The other reason for the collar is to optimize cerebral venous drainage by maintaining the head in a midline position to minimize exacerbation of any intracranial hypertension. Caution should be used to not overtighten the collar because this impairs venous drainage. Finally, the head of the bed should always be maintained at 30°, again to optimize venous drainage.
Endotracheal intubation by well-trained personnel in the emergency department or ICU setting is recommended for patients with a GCS score of 8 or less. The potential benefit of prehospital intubation for such patients remains controversial after conflicting results of 2 major studies. The presence of an intact gag reflex should not deter from intubation. Intubation may have a deleterious effect on a TBI patient’s ICP. Even simply laryngoscopy has been shown to cause a reflex sympathetic response, releasing catecholamines and thus increasing systemic blood pressure, which may worsen ICP.
Many experts prefer rapid sequence intubation as a technique to secure the airway in TBI patients, but no RCT has been done to support this practice. Potential advantages of rapid sequence intubation are blunting of the sympathetic response, preoxygenation, and possibly a lower risk of aspiration. Because most comatose patients have intact gag reflexes, sympathetic responses occur and may have harmful effects. A common practice is to use lidocaine to blunt the gag response to intubation but it has not been conclusively shown either good or bad. A single dose of a very short-acting opioid, such as fentanil or sufentanil, in select cases may prove helpful minimize the response but this benefit must be weighed against the potential for worsened hypotension or premature respiratory depression.
Once definitive airway control is obtained, hypercarbia can be managed. A clear goal is to treat hypercarbia immediately, which is achieved by properly controlling minute ventilation. Continuous end-tidal carbon dioxide monitoring can be helpful in guiding mechanical ventilator adjustments. If there is increased ICP, then hyperventilation may be tried. Although widely used, this treatment option has potential for worsening the clinical state. Hyperventilation leads to cerebral vasoconstriction, which potentially exacerbates ischemia. If used, the goal should be hyperventilation to P co 2 34 mm Hg to 36 mm Hg as a temporary measure while arranging for more definitive therapies, such as hyperosmotic therapy or neurosurgical intervention. Hyperventilation without evidence of increased ICP or impending herniation should not be done. It has been shown that prophylactic hyperventilation worsens outcomes, possibly by lowering cerebral blood flow (CBF) and worsening ischemia.
After intubation, a portable chest radiograph should be obtained to confirm appropriate endotracheal tube placement and evaluate for signs of aspiration. Aspiration is a common problem in TBI patients and may lead to poor oxygenation or acute respiratory distress syndrome.
Positive end-expiratory pressure (PEEP) should be used judiciously in TBI patients. If needed to improve oxygenation, then the lowest level should be used that achieves clinical goals. High PEEP, however, can impair cardiac venous return, which may decrease cerebral venous drainage and thus worsen ICP. Studies on PEEP have shown that the impact of PEEP on ICP and cerebral perfusion pressure (CPP) varies greatly among TBI patients, necessitating close monitoring and individual tailoring of this therapy.
In cases of suspected elevated ICP, central venous access is needed. This catheter can be used to deliver hypersomolar saline solutions that are 3% or higher because these solutions can cause venous phlebitis. Also, vasopressors may be needed to meet CPP goals. Finally, a central venous catheter allows measurement of central venous pressure. Central venous pressure is important for providing a measurement of intravascular volume state. Volume management is an important aspect of trauma care.
Many experts recommend avoiding internal jugular catheters because compression or clot of this vessel may increase ICP. Also, placing this line requires putting patients in the Trendelenburg position. In this head-down position, ICP increases due to cerebral venous congestion. The femoral vein approach is safer than the subclavian but has a higher likelihood of infection. Thus, the subclavian approach is preferable.
Other accesses recommended are an arterial catheter and gastric tube. A radial arterial catheter should be inserted to allow optimal cerebral perfusion pressure management and frequent blood sampling. A feeding tube is needed. For this purpose, an orogastric tube should be used rather than a nasogastric tube until skull base and facial fractures are safely ruled out.
As discussed previously, hypotension must be prevented and treated aggressively. Studies reveal a direct correlation between negative fluid balance and worse neurologic outcome in TBI patients. On admission, unless there is clinical evidence to the contrary, patients should be treated as though they are intravascularly depleted. Hypotension should be considered the result of hypovolemia or acute blood loss rather than a manifestation of TBI. In spinal cord injury, however, hypotension is frequently the result of compromised sympathetic tone. Fluid resuscitation using isotonic crystalloids is generally recommended to meet intravascular volume goals. Prehospital hypertonic saline resuscitation has been effective in some settings, such as the battlefield, but studies do not support its use in the civilian setting. A randomized study of albumin for volume resuscitation suggests increased mortality and thus is not recommended. In cases of elevated ICP, hypertonic saline solutions are considered. Hypotension in these cases is defined as a systolic blood pressure less than 100 mm Hg or, in cases of intracranial hypertension, a cerebral perfusion pressure less than 70 mm Hg.
At the other extreme, severe hypertension is dangerous in this patient population. It has been shown to be predictive of mortality and pneumonia. Under these circumstances, blood pressure should be quickly lowered with agents, such as intravenous (IV) labetalol, nicardipine, or enalaprilat, because these agents have not been shown to cause elevations in ICP.
A thorough laboratory work-up, including complete blood cell count, electrolytes, glucose, coagulation parameters, blood alcohol level, pregnancy test (when appropriate), and urine toxicology, should be sent as soon as possible because these factors may dramatically influence a patient’s treatment plan. Electroencephalography (EEG), either intermittent or continuous, is highly recommended because nonconvulsive seizures have been detected in 23% of moderate to severe TBI patients and are associated with the development of hippocampal atrophy.
Additional imaging
Initial neuroimaging that is normal does not preclude development of delayed lesion, such as edema or hemorrhage. Frontal or temporal contusions often worsen a day or 2 after the initial event. This is commonly caused by edema formation or hemorrhage, both of which can lead to fatal brainstem compression. Temporal contusions classically enlarge and cause uncal herniation.
It is recommended to repeat neuroimaging after several hours if there is any clinical deterioration or if a coagulopathy is present. Close attention should be paid for subarachnoid blood, which may be traumatic or due to aneurysmal rupture. A patient found down and thought to have suffered a TBI may have had a spontaneous subarachnoid hemorrhage from a ruptured cerebral aneurysm. Thus, if clinically suspicious, angiography should be considered in select cases to evaluate for underlying vascular malformation, such as aneurysm.
Although MRI provides much higher fidelity brain and spinal cord images than CT, these additional data are rarely helpful in guiding initial resuscitation. For this reason, CT is adequate. Once stable, however, the patients should undergo MRI. Typically, this is a day or 2 after admission. MRI may clarify and highlight regions of edema, microhemorrhages, or infarction. Newer sequences, such as gradient-recalled echo, susceptibility-weighted imaging, diffusion-weighted imaging, and diffusion tensor imaging, provide greater sensitivity for specific types of injury and hold potential for better prognostic tools in the future. Either CT or MRI of the cervical spine should be considered in all severe TBI patients based on clinical suspicion.
Intracranial hypertension after TBI
Most severe TBI patients develop intracranial hypertension as defined as ICP greater than 20 mm Hg. Consequently, TBI patients with GCS score of 8 or less after resuscitation should be considered as having this condition until direct ICP measurement proves otherwise. Because these patients typically have severely compromised neurologic examinations, thus limiting an examiner’s ability to detect deterioration, ICP monitoring becomes a tool for assessing clinical worsening. Sustained elevations in ICP generally herald ongoing secondary injury, such as cerebral edema, hemorrhage, hydrocephalus, or ischemia, and, as a consequence, the management of ICP has become a foundation of neurocritical care. Several studies provide evidence that there is a correlation between the extent and duration of ICP elevation and poor outcomes after TBI. Despite this association, evidence is lacking that supports the notion that ICP management improves clinical outcome. Because the brain is anatomically compartmentalized, herniation syndromes may occur without associated changes in global ICP. An example is after posterior fossa hemorrhage, there may be brainstem herniation without changes in ICP above the tentorium.
CPP, which is the difference of ICP and mean arterial pressure, is generally used to understand the connection between systemic circulatory function and CBF. CPP has become a surrogate for determining adequacy of brain perfusion. CPP is a global measure and does not provide any insight into regional differences in perfusion. Normal subjects autoregulate CBF. In an uninjured state, CBF is constant at 50 mL/100 g tissue/min across a wide range of MAP. At least one-third of TBI patients, however, have abnormal cerebral autoregulation. When this occurs, such patients have pressure passive perfusion. CBF varies directly with changes in MAP. With increased MAP, CBF increases leading to increased cerebral blood volume and, thus, increased ICP. Conversely lower MAPs may cause hypoperfusion and ischemia. For this reason, a goal CPP between 50 mm Hg and 70 mm Hg is usually targeted. This value has been chosen because it allows sufficient pressure to overcome the elevated ICP while avoiding problems with hypertension.
CPGs recommend ICP monitoring in patients with severe TBI who are comatose (GCS score <9) after resuscitation and who either have abnormalities on CT scan or meet at least 2 of the following 3 criteria: age greater than 40 years, systolic blood pressure less than 90 mm Hg, or motor posturing. Optimally, ICP is measured by devices placed inside the brain—intraventricular or intraparenchymal monitors. Intraventricular catheters or extraventricular drains are favored by most neurocritical care specialists because these devices allow for therapeutic drainage and sampling of CSF. Such ICP monitoring devices are resource intensive. They also carry a small but significant risk of infection and hemorrhage and, in cases of intraparenchymal monitors, are prone to drift over time. Other options that require less maintenance and have lower infection risks but that do not allow for therapeutic drainage of CSF are the subdural bolt, intraparenchymal fiberoptic ICP monitor, and the epidural ICP monitor.
ICP may not be the only important intracranial variable that should be measured. Studies of TBI patients with parenchymal oxygen or microdialysis probes have shown that brain tissue hypoxia and metabolic distress occur independently of ICP or CPP. Ischemia occurs in some patients at apparently adequate CPP levels. Critics of ICP monitors are quick to point out that clinical outcome-based studies are mixed regarding the impact of ICP monitoring. Some studies evaluating protocol-driven care of severe TBI, including ICP monitoring, have shown benefit; others suggest that ICP monitoring may be associated with increased mortality, greater therapeutic intensity, and longer length of stay in the ICU. In spite of the shortcomings of ICP-directed therapy, none of the other variables has been shown similar. To date, there are no RCTs that provide convincing evidence that using tissue oxygen, microdialysis, or any other modality improves outcome. All of this highlights the need for more research focused on individualized treatment protocols that may be based on multiple modality monitoring.
Because the current CPGs recommend using ICP monitoring principally, then an important treatment goal is to maintain the ICP less than 20 mm Hg and the CPP between 50 mm Hg and 70 mm Hg. If ICP remains poorly controlled after intubation with controlled ventilation and head-of-bed elevation, then mannitol should be given in a dose of 0.5 g/kg to 1 g/kg through either a peripheral or central vein. This therapy usually takes approximately 15 to 30 minutes to have an effect on ICP. Repeat boluses every 6 hours can be given. Some experts recommend using a serum osmolarity goal of 310 mOsm/L to 320 mOsm/L to achieve optimal effect. Renal function and fluid balance should be monitored while using mannitol because this is a diuretic. Osmotic diuresis occurs as does a lowering of the serum sodium level. Fluid balance can be restored using saline solutions. Studies suggest that mannitol not only lowers ICP but also improves CBF to hypoperfused areas of the brain in patients with high ICP.
An increasingly popular therapy is hypertonic saline. This therapy has the advantage of increasing serum sodium and, thus, serum osmolarity without causing diuresis. It is especially useful when faced with a TBI patient who has compromised intravascular volume, such as from hemorrhagic shock. For hyperacute ICP and herniation treatment, 23% hypertonic saline is administered through a central venous line. Up to 50% reduction in ICP can occur. The onset of effect is within minutes and the effect lasts for a few hours. For prolonged therapy, a continuous IV infusion of 2% or 3% hypertonic saline is used starting at 75 mL/h and titrating as needed. The objective should be achieving an ICP goal or a desired serum osmolarity. If concentrated sodium solutions are used, sodium chloride and sodium acetate should be used as a 50/50 mix to minimize hyperchloremic metabolic acidosis. When compared with mannitol, no significant differences have been found with respect to the extent of reduction of ICP or duration of action.
If initial ICP-lowering interventions are unsuccessful, pharmacologic coma or surgical decompression should be considered. The proposed effect of pharmacologic coma on ICP is via reduction of cerebral metabolic rate of oxygen, often by induction of pentobarbital coma. A few studies suggest that barbiturates are effective at reducing ICP but their effect on long-term outcomes is not clear. A retrospective study of severe TBI patients with refractory intracranial hypertension treated with pentobarbital coma showed 40% survival rates with 68% of those patients achieving good functional outcomes.
If the decision to use pharmacologic coma is made, pentobarbital can be administered at a loading dose of 5 mg/kg IV, followed by an infusion of 1 mg/kg/h to 3 mg/kg/h. The loading dose is 2 mg/kg IV followed by an infusion up to 200 μg/min. Continuous EEG monitoring is recommended to attain a goal of burst suppression. Decisions to initiate pharmacologic coma should not be taken lightly or in patients lacking hemodynamic stability. Pentobarbital coma carries well-known side effects, including hypotension, hypocalcemia, hepatic and renal dysfunction, and increased susceptibility for sepsis.
Propofol is another option to produce coma and has the advantage of a much shorter half-life. Propofol use often results in hypotension requiring vasopressor support and, if used for a prolonged period, may cause the rare but deadly propofol infusion syndrome. An advantage of propofol over pentobarbital is its short half-life, allowing for wake-ups to perform neurologic examinations. Despite its widespread use, there has been some concern after a study suggested that propofol was unable to reduce ischemic burden from TBI in spite of achieving EEG burst suppression.
For the past few decades, decompressive craniectomy has been used for control of refractory ICP. A recent landmark RCT—Decompressive Craniectomy (DECRA)—compared bilateral decompressive craniectomy with standard medical therapy and failed, however, to show superior 6-month neurologic outcomes after surgery. Critics of this study state that the results are not generalizable because the ICP in the standard-care group was not severely elevated enough and many patients who underwent surgery did not have refractory high ICP. Consequently, this study does not invalidate the practice of resorting to decompressive craniectomy after a longer period of refractory ICP. Moreover, the study did not evaluate patients with unilateral craniectomy or those with focal space-occupying lesions, such as hematomas.
TBI patients are seriously ill; thus, meeting CPP goals may be difficult using IV fluids alone. If so, vasoactive pharmacologic agents, such as norepinephrine and phenylephrine, may be required. These 2 agents are preferred because they are considered to have the least effect on cerebral vasomotor tone. Invasive hemodynamic monitoring with a central venous pressure catheter to ascertain intravascular fluid state should be done concomitantly. It should be remembered that barbiturates and propofol are myocardial depressants and, thus, aggressive cardiovascular management probably is necessary in the event of pharmacologic coma. High CPP goals (>70 mm Hg) should be avoided due to an increased risk of developing acute respiratory distress syndrome.
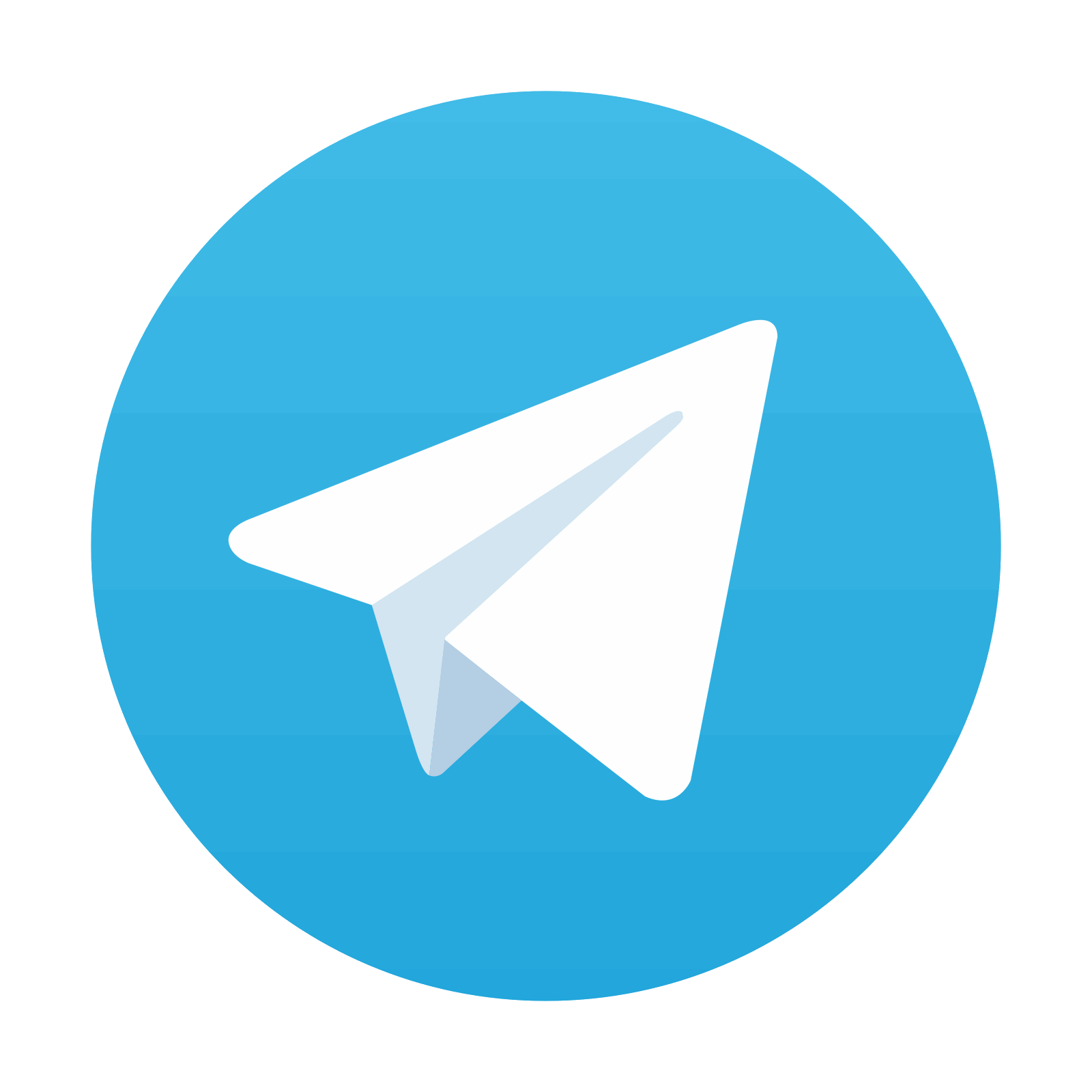
Stay updated, free articles. Join our Telegram channel

Full access? Get Clinical Tree
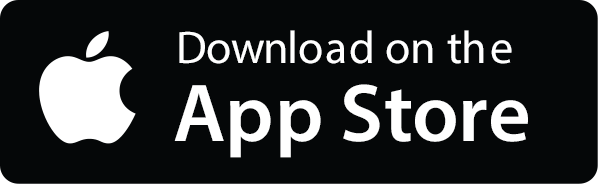
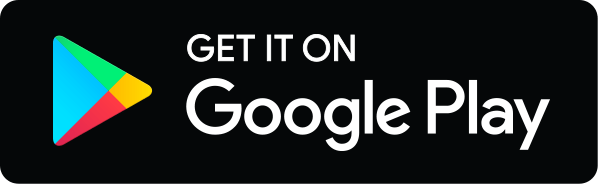