© Springer International Publishing Switzerland 2015
Javier Fandino, Serge Marbacher, Ali-Reza Fathi, Carl Muroi and Emanuela Keller (eds.)Neurovascular Events After Subarachnoid HemorrhageActa Neurochirurgica Supplement12010.1007/978-3-319-04981-6_26Pharmacological Modulation of Spreading Depolarizations
(1)
Department of Neurosurgery, Heidelberg University Hospital, Im Neuenheimer Feld 400, 69120 Heidelberg, Germany
Abstract
Spreading depolarization (SD) is a wave of almost complete depolarization of the neuronal and glial cells. Nowadays there is sufficient evidence demonstrating its pathophysiological effect in migraine with aura, transient global amnesia, stroke, subarachnoid hemorrhage, intracerebral hemorrhage, and traumatic brain injury. In these cases, occurrence of SD has been associated with functional neuronal damage, neuronal necrosis, neurological degeneration, and poor clinical outcome. Animal models show that SD can be modulated by drugs that interfere with its initiation and propagation. There are many pharmacological targets that may help to suppress SD occurrence, such as Na+, K+, Cl−, and Ca2+channels; Na+/K+-ATPase; gap junctions; and ligand-based receptors, for example, adrenergic, serotonin, sigma-1, calcitonin gene-related peptide, GABAA, and glutamate receptors. In this regard, N-methyl-d-aspartate (NMDA) receptor blockers, in particular, ketamine, have shown promising results. Therefore, theoretically pharmacologic modulation of SD could help diminish its pathological effects.
Keywords
Spreading depolarizationKetamineNMDA receptorTherapyIntroduction
Spreading depolarization (SD) is a wave of neuronal and glial depolarization that propagates across the cortex; it is characterized by neuronal swelling, distortion of dendritic spines, and depression of neuronal activity [9]. Underlying this phenomenon is a dramatic failure of brain ion homeostasis, acidification, efflux of excitatory amino acids, and increase of energy metabolism [29]. Experimentally, SD can be elicited through chemical, mechanical, or electrical stimulations. However, this depolarization also occurs spontaneously after mechanical damage, hypoxia, ischemia, or hypoglycemia [45]. SD is associated with important hemodynamic changes. The physiological hemodynamic response during SD development is arteriolar vasodilation. As a result, increments of more than 100 % of cerebral blood flow (spreading hyperemia) occur, followed by a mild but prolonged hypoperfusion (spreading oligemia). In tissue at risk, such as areas adjacent to subarachnoid hemorrhage (SAH), an inverse hemodynamic response with hypoperfusion (spreading ischemia) can be observed. It has been shown that, under pathologic circumstances, SD can produce oxidative stress, worsen hypoxia, and induce neuronal death [9, 10].
Since its original description by the Brazilian physiologist Aristides Leão in 1944, SD has been extensively characterized in animal models [31]. Not until the past decade has an electrophysiological confirmation of SD in human brain been found and its role in the pathophysiological basis of several neurological conditions addressed [20, 29]. Today there is sufficient evidence showing that SD plays an important role in migraine with aura (MA), transient global amnesia, stroke, SAH, intracerebral hemorrhage, and traumatic brain injury (TBI), where SD occurrence has been associated with functional neuronal damage, neuronal necrosis, neurological degeneration, and poor clinical outcome [20, 29]. Therefore, therapeutic modulation of SD has raised high expectations.
In the human brain, pharmaceutical targeting of SD is still in its infancy. Nonetheless, a large body of experimental study shows that SD can be presumably modulated by drugs that interfere with its initiation and propagation. The range of targets is wide and includes ion trafficking such as Ca2+, K+, and voltage-gated Na+ and Cl− channels, along with Na+/K+-ATPase and gap junctions, and many ligand-based receptors, for example, adrenergic, serotonin, sigma-1, and glutamate receptors [2, 16, 45]. In addition, many analgesics and sedatives seem to exert an impact on the susceptibility and occurrence of SD [27]. In particular, N-methyl-d-aspartate (NMDA) receptor antagonists have been shown to inhibit SD initiation and/or propagation and they seem to be, at the moment, the most effective drugs for SD suppression [48]. Based on these observations, pharmacological modulation of SD in humans as a neuroprotective therapy could be feasible. Here, we briefly review some important aspects of pharmacological modulation of SD.
Clinical Implications of Spreading Depolarization
SD has been observed in patients suffering from SAH using subdural electrocorticographic strip electrodes. It has been shown that SD has a high incidence in this type of cerebrovascular disease, reported to be between 66 and 100 %; there is also a relationship between delayed cerebral ischemia (DCI) and SD occurrence in approximately 44–88 % of the cases [40]. Repeated SDs with prolonged depression periods have been found as an early indicator of DCI after SAH [15]. This finding called into question the role of angiographic cerebral vasospasm as the main cause of DCI, suggesting that SD has a potential pathophysiological mechanism in its development [52]. It is expected that pathological conditions that occur after SAH, such as hypoxia, low glucose, high extracellular concentrations of K+, and nitric oxide depletion by free hemoglobin products, are capable of triggering SD [11, 12]. Then, SD is initiated under conditions when (1) metabolic demands are not met per se and (2) the hemodynamic response is turned from hyperemia to spreading ischemia. This could lead into a self-perpetuating cycle with consecutive cortical necrosis and DCI formation [9]. Therefore, treatments targeting SD should decrease DCI and improve clinical outcome. In this regard, there is indirect evidence that the l-type Ca+ inhibitors, nimodipine [14, 49] and nicardipine [50], or the NMDA receptor blocker ketamine [25] could improve outcome by SD suppression in patients.
In other cerebrovascular diseases, spontaneous SDs have also been observed with high incidence. In stroke, for example, SDs are present in 86–100 % of patients and their presence is associated with infarct growth. It is hypothesized that prevention and treatment of SD may also help to avoid secondary ischemia and fatal brain edema [8]. SDs have been found in 50–60 % of TBI patients and their presence is related to unfavorable outcome [22, 23].
SD is now widely recognized to be the neurophysiological substrate of MA and a potential trigger of the headache [29]. Genetic factors that favor hyperexcitability of the visual cortex may enhance susceptibility to SD, such is the case for familial hemiplegic migraine (FHM), a genetic form of migraine that involves mutations in genes that encode for voltage-gated P/Q-type Ca2+ channel subunits (FHM1), Na+/K+-ATPase subunits (FHM2) [19], or Na+ channel subunits (FHM3) [38]. Transgenic mice carrying FHM mutations show increased susceptibility to SD, with a vastly reduced triggering threshold, an increased propagation velocity, and frequently multiple SDs after a single stimulus [33]. Another example is the K+ channel, TRESK, which is regulated by Ca2+ concentrations, where it has been shown that a dominant-negative mutation is linked to MA and SD [17]. Over the past years, several antimigraine drugs have been tested on SD. Tonabersat, a benzoylaminobenzopyran anticonvulsant, suppresses SDs in animal models after intraperitoneal administration; however, the clinical trials show conflicting results [2, 16]. Many other antimigraine drugs have been tested or are under investigation, e.g., valproate, propranolol, methysergide, amitriptyline, topiramate, gabapentin, lamotrigine, flunarizine, and olcegepant, although the results are contradictory. These drugs have an inhibitory effect on ion channels, receptors, and neurotransmitters such as Na+, l-type Ca2+, P/Q- and N-type Ca2+ channels, gap junctions, GABAA, and calcitonin gene-related peptide receptors, thus, supporting the role of multiple targets [6]. Research into SD inhibitory antimigraine drugs could, therefore, have a collateral impact on cerebrovascular diseases and brain trauma.
Pharmacological Targets and SD Modulation
When SD develops, a surge of [K+]e occurs, which leads to depolarization of adjacent neurons and glial cells. At the same time, [Na+]e decreases as it enters the cells accompanied by water. This process is followed by neuronal swelling and shrinkage of the extracellular space. At the same time, influx of Ca2+ also occurs, leading to release of glutamate and other neurotransmitters. Glutamate induces NMDA receptor activation, which may contribute to SD initiation, propagation, and, if released in excess, can generate excitotoxicity. Moreover, gap junctions are thought to play an important role in SD expansion by promoting intercellular Ca2+ and K+ waves. After SD, [K+]e is restored by Na+/K+-ATPase and astrocytes [44]. This suggests Na+, K+, Ca2+ channels, Na+/K+-ATPase, gap junctions, and NMDA receptors as the main targets for SD modulation.
Voltage-Gated Na+ Channels
The role of Na+ channels in SD generation or propagation is under debate. Tetrodotoxin (TTX), one inhibitor of voltage-gated Na+ channels, is sufficient to diminish the SD phenomenon or reduce the propagation velocity, but it does not prevent SD. Actually, TTX has a stronger effect on hypoxic spreading depolarization than on SD [45]. Conversely, other studies demonstrated there is no sign of any attenuation of SD in the presence of TTX [46]. However, dysfunction of Na+ channels has been confirmed in FHM3, which provides further support for the potential role of these channels in generation of SD [7].
K+ Channels
Voltage-gated K+ channels control neuronal excitability and inhibit hyperexcitability. Generally, the increase of membrane K+ conductance will lower neuronal excitability by hyperpolarization. Concurrent with this, several studies have shown that blockages of K+ channels (e.g., Ba2+, Rb+, Cs+, tetraethylammonium chloride (TEA), and 4-aminopyridine) lower the threshold of SD, and some of these substances can even induce spontaneous SDs [18]. K+ channel opening can reduce SD incidence and propagation velocity [51]. However, it is unclear whether these effects are specific for special channel types or a nonspecific outcome from hyperpolarization.
Ca2+ Channels
Ca2+ channels have a potential role in SD generation or propagation [33]. Important Ca2+ channels subtypes seem to be P/Q-type (Cav2.1) and N-type (Cav2.2), which have been related to special types of migraine [28]. After the blockage of P/Q-type Ca2+ channels by ωAgaIVA, SD cannot be induced in mouse cortical slices, whereas blockage of N- or R-type Ca2+ channels by ωCgtxVIA and SNX-482 has only a small inhibitory effect on SD threshold and propagation velocity [47]. Many of the medications blocking this channel currently tested on animal models are primarily antimigraine drugs such as gabapentin, flunarizine, topiramate, and valproate. They seem to elevate the electrical threshold, and decrease the frequency, duration, and propagation of SD. However, the results remain inconclusive [2, 3]. In general, l-type Ca2+ channels have no direct effect on SD generation and propagation. Nonetheless, blockers of this subtype channel, such as nimodipine and nicardipine, can suppress the vasoconstriction effect of high [K+]e. Thus, they can correct the inverse hemodynamic response induced by SD under pathological conditions [30, 50].
Na+/K+-ATPase
Na+/K+-ATPase is important for restoring [K+]e after SD, so it is critical for SD occurrence. Consistent with this, inhibitors (e.g., ouabain) of Na+/K+-ATPase are capable to enhance SD susceptibility [21]. Additionally, a ketogenic diet related to augmentation of Na+/K+-ATPase activity can suppress SD [4].
Gap Junctions
Gap junctions are the basis of a functional synchronization between neighboring cells. Some studies have shown that gap junction blockers (e.g., heptane, octane, quinine, quinidine, mefloquine, halothane, isoflurane, and tonabersat) inhibit SD propagation or duration [34, 35]. However, it is difficult to ascertain the role of neural or glial gap junctions in SD because of the lack of specificity of gap junction blockers. Moreover, the specific gap junction blocker, carbenoxolone, seems to have no effect on SD [37]. More evidence is needed to support the role of gap junctions in SD.
NMDA Receptors
N-methyl-d-aspartate (NMDA) receptors have a critical role in excitatory synaptic transmission, excitotoxicity, and plasticity in the central neuronal system. Both competitive and noncompetitive NMDA receptors blockers are able to modulate SDs. Some of the drugs already tested are AP5, AP7, CPP, CP-101,606, PCP, MK-801, ketamine, Mg2+, Zn2+, and N2O, which have shown the capacity to elevate SD electrical threshold, block or slow SD propagation, and reduce SD duration and speed [2, 24, 25]. However, the use of most of them is limited because of their harmful side effects.
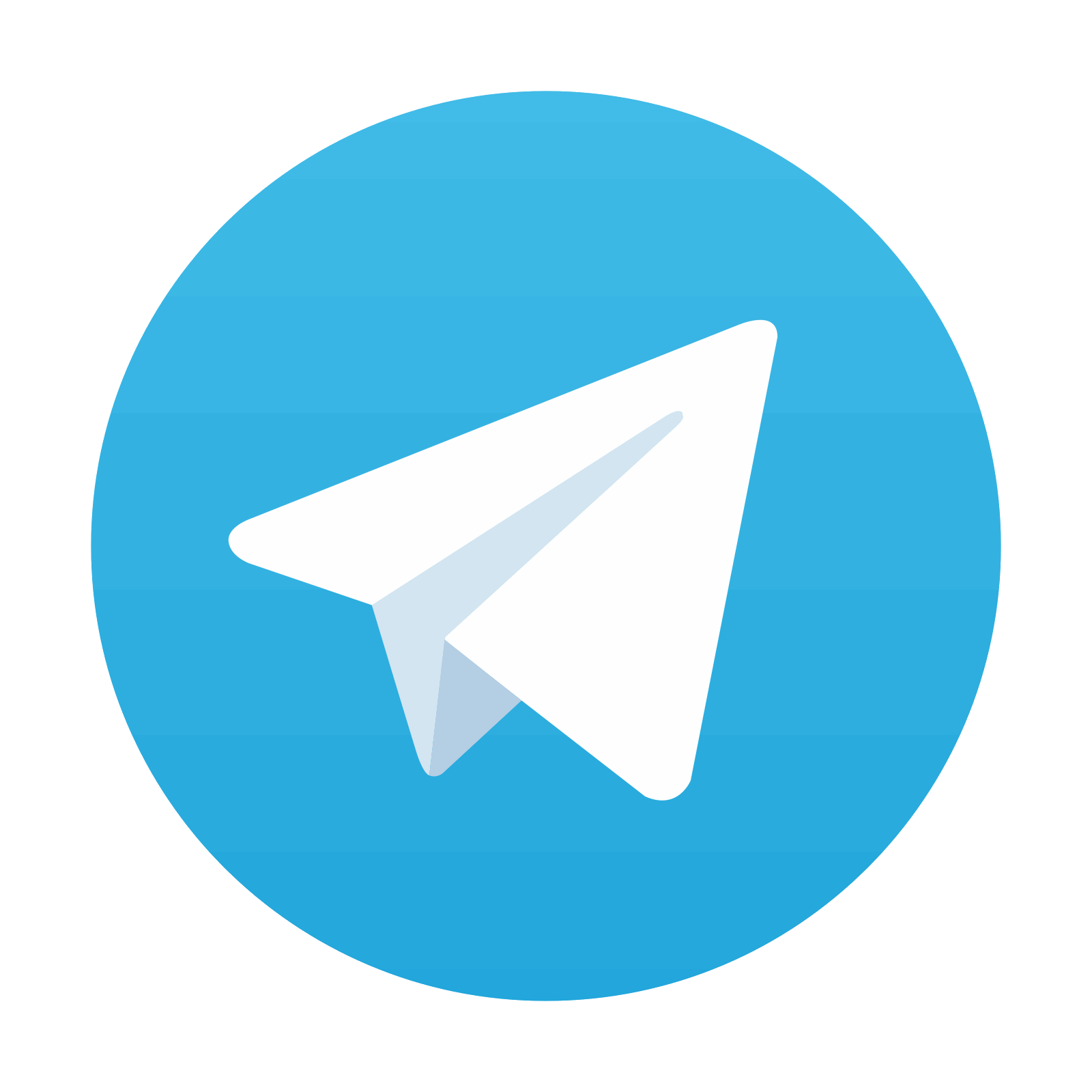
Stay updated, free articles. Join our Telegram channel

Full access? Get Clinical Tree
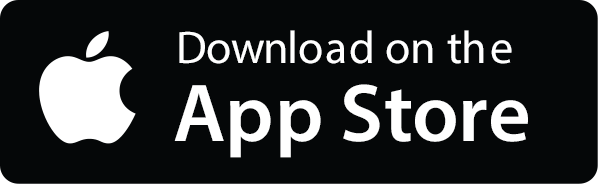
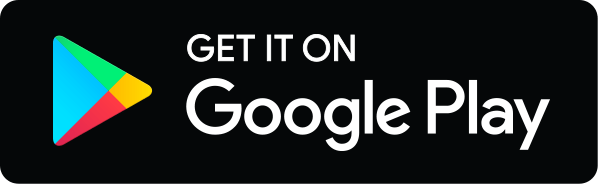