Fig. 15.1
Nicotinic receptors. Graphic presentation of homomeric and heteromeric receptors and their location. Most functional nAChRs contain more than one subunit, and are therefore heteromeric. The different subunit combinations determine the receptor properties in terms of affinities for different ligands electrophysiological properties, Ca2 + permeability and ability to be ‘unregulated’ by nicotine. Yellow circles refere to acetylcholine binding sites. (Reprinted in a modified format from Taly et al. (2009, pp. 733–750). Copyright (2009) with permission from Nature Publishing Group; and from Gotti et al. (2006). Copyright with permission from Elsevier
The composition of the nicotinic receptor is important, since distinct subunits not only play a role in different functional conformation characteristics of the receptor, but also regulate cholinergic neurotransmission in distinct brain areas. Thus, α7 containing receptors are associated with low affinity for nicotine and a rapid desensitization in presence of agonists, whereas α4β2 receptors have higher affinity for nicotine and slower rates of desensitization (reviewed in Metherte 2004). Incorporation of the alpha5 nicotinic acetylcholine receptor (nAChR) subunit into the α4β2 nAChRs increases the excitatory response and influences the maturation of layer VI prefrontal cortex neurons (Bailey et al. 2012) and is required for normal attentional behaviours in adult mice (Bailey et al. 2010). The α3β2 subunits are expressed in the visual pathway with α4α6β2β3 receptor subtypes being present in visual and mesostriatal pathways, and most likely having specific roles in the brain function (reviewed in Steinlein and Bertrand 2008), whereas α4β3 heteromeric receptors play a role in controlling glutamate release (Alkondon and Albuquerque 2004) .
The α7 nicotinic receptors are highly enriched in hippocampal neurons, co-localizing with GABA-ergic neurons (Kawai et al. 2002) and dendrites (Sylvester Vizi et al, 2004) and mediate GABA release, whereas nicotinic agonists against the α7 nicotinic receptors modulate dendritic growth (Torrao et al. 2003). Thus, the documented association of microdeletion of chromosome 15q13.3 that encompasses several genes, including the gene for α7 nicotinic acetylcholine receptor (CHRNA7) results in decrease in hippocampal α7 receptor density, abnormal hippocampal auditory sensory processing and increased hippocampal CA3 pyramidal neuronal activity in animal studies (Adams et al. 2012), further providing support that reduced CHRNA7 expression may contribute to the hippocampal abnormalities of inhibitory circuits, as observed in ASD. Interestingly, the allosteric potentiation of α7 nAChR may mediate the antipsychotic-like effect of the cholinesterase inhibitor galantamine as an adjunctive treatment (Wiker et al. 2008), providing further support for development of novel α7nAChR selective antipsychotic treatment that could be used in schizophrenia and other related psychiatric disorders, including ASD .
15.2.1.2 Muscarinic Acetylcholine Receptors
The muscarinic acetylcholine receptors (mAChR) mediate most of the action of acetylcholine not only in the central and peripheral nervous system, but also in the end organs of the parasympathetic nerves (e.g. cardiac and smooth muscles and secretory glands) .
mAChRs are implicated in learning and memory. Out of the five mAChR (M1-M5) identified in mammals, M1 receptors are important for cognitive processes, including working memory (Felder et al. 2000; Auld et al. 2002) and activation of M1 receptors has cognition-enhancing effects, including restoring impaired learning (Shirey et al. 2009). In contrast, neurodegenerative processes, e.g. amyloid deposits, can decrease the neurotransmission signals of these receptors, resulting in decreased cholinergic activity (Auld et al. 2002).
M2 mAChR is located presynaptically, and similar to M1 mChAR, is predominantly found in the cerebral cortex and hippocampus, but in contrast to M1, is present both on cholinergic and noncholinergic terminals (reviewed in Nathanson 2008). In contrast to the M1 and M2 mAChRs that are found within the brain tissue, M3 receptors are localised to the peripheral nervous system, and contribute to the cholinergic stimulation of gastrointestinal motility (Matsui et al. 2000), mediate cholinergic-induced dilatation of coronary arteries (Lamping et al. 2004), and detrusor bladder contractions (reviewed in Abrams et al. 2006), stimulate parathyroid gland secretion (Culp et al. 1996) and mediate salivation (Matsui et al. 2000). Interestingly, a recent case report (Petersen et al. 2013) identified an individual with autism who had a deletion in 1q43 comprising only one coding gene, CHRM3, for mAChR M3. This finding expands upon previous animal knock-out M3 receptor studies, on the link between the M3 muscarinic receptor pathophysiology and the neurocognitive phenotype of ASD .
The M4 mAChRs in contrast are inhibitory autoreceptors for acetylcholine. Their activation inhibits acetylcholine release in the striatum. The depression in M4 activity (as demonstrated in M4 knock-out mice) results in abnormal social behaviour, including locomotor hyperactivity, reduction in social contacts, sensory motor gating deficits (Koshimizu et al. 2012), similar to those found in ASD subjects and schizophrenia. The M5 mAChR receptors are also found in the hippocampus and various subcortical areas, including substantia nigra, ventromedial hypothalamic nucleus, mammillary bodies, and ventral tegmental area (Vilaró et al. 1990) and may play a role in the regulation of the dopaminergic nigrostriatal pathway, and also up-regulate the expression of immunoglobulin G and proinflammatory cytokines (Kawashima et al. 2012) .
15.3 Post-Mortem Studies
15.3.1 Muscarinic Receptors in Autism
Muscrininic receptor changes have not been extensively investigated in autism . Our own post-mortem study has found 30 % decrease in M1 receptor binding in the cortical regions of autistic subjects (Perry et al. 2001; Table 15.1). This could be attributed to epilepsy, as 40 % of autistic children are estimated to suffer from epilepsy (Minshew et al. 1997). Furthermore, low numbers of M1 receptors have been reported in hippocampal sclerosis, linked with temporal lobe epilepsy (Pennell et al. 1999) and in the hippocampal CA1 region in aged epileptic animals (Cavarsan et al. 2011). However, the findings of Perry et al. (2001) were not due to presence of epilepsy within the series of autism individuals examined. The findings from the latter study are similar to those reported for schizophrenia, where one of the more consistent neurotransmitter abnormalities is loss of the various muscarinic receptors (e.g. M1 and M4) in different brain areas, including the superior frontal and temporal gyri and hippocampus (Mancama et al. 2003; Deng and Huang 2005; Scarr et al. 2007; Kang et al. 2009) .
Table 15.1
Summary of post-mortem studies focusing on the cholinergic system in adults with ASD
Study | Subjects | Main findings in adult subjects with autism compared to control |
---|---|---|
Perry et al. 2001 | 7 autistic adults (diagnosed by DSM-IV criteria), 6 MR adults, 3 adults with Down’s syndrome, 6 controls | Lower cortical M1 receptor binding (up to 30 %) and decrease in nicotinic receptors by 65–73 % present in the parietal and frontal cortices |
Lower levels of Α4 and β2 nicotinic receptor subunits were found in the parietal cortex | ||
BDNF expression was three-fold higher in the basal forebrain | ||
Lee et al. 2002 | 8 autistic MR adults (diagnosed by DSM-IV criteria), 11 non-autistic MR adults, 10 controls | A reduction of 40–50 % nicotinic receptor binding to the agonist epibatidine (subunits Α3, Α4, β2) in the granular, Purkinje and molecular layers |
A three-fold increase in the nicotinic receptor binding of Α-bungarotoxin (Α7 subunit) in the granule cell layer | ||
A decrease in Α4 receptor subunits in Purkinje and other cell layers | ||
A non significant increase in Α7 subunit in the granule cell layer | ||
Martin-Ruiz et al. 2004 | 6 autistic adults (diagnosed with DSM-IV criteria), 8 controls | Lower expression of Α4 and β2 subunit mRNA levels, protein expression and receptor binding density in the parietal cortex |
A4 subunit mRNA levels were higher and the protein expression and receptor density decreased in the cerebellum | ||
Non significant increases in Α7 subunit mRNA and protein expression levels and significant increases in receptor binding density in the cerebellum | ||
Ray et al. 2005 | 3 autistic adults (diagnosed by DSM-IV criteria), 3 controls | Decrease in Α7 and β2 immunoreactive neurons in the paraventricular nucleus (PV) and nucleus reuniens |
No difference between autistic and control adults in co-expression of Α7 and glutamic acid decarboxylase in the PV | ||
The above suggests that the loss of Α7 subunits in autism is not due to a loss of GABA-ergic neurons |
15.3.2 Nicotinic Receptor Changes in Autism
We have demonstrated significant loss of nicotinic receptors (40–50 and 65–73 % for the cerebellar cortex, and the parietal and frontal cortices, respectively) in autistic adult subjects compared to control counterparts (Perry et al. 2001; Table 15.1). In the parietal cortex specifically, there were 30 % lower levels of α4 and β2 nicotinic receptor subunits (p < 0.05). Similar changes were also reported for the thalamus, with a significant decrease in numbers of α7 and β2 immunoreactive neurons in the paraventricular nucleus and nucleus reuniens in autistic subjects (Ray et al. 2005).
The profound involvement of the nicotinic cholinergic system in autism was further supported by a study in the cerebellar cortex, that reported lower nicotinic receptor binding for the agonist epibatidine (α3, α4, β2) restricted to the granule cell, Purkinje and molecular layers in the analysed autistic subjects (Fig. 15.2). In contrast, in the granule cell layer, there was a three-fold increase in the nicotinic receptor binding for the agonist α-bungarotoxin (α7 subunit), despite choline acetyltransferase (ChAT) levels being similar in autistic and control subjects (Lee et al. 2002). This suggests that the presynaptic cholinergic system is not altered in autistic subjects.


Fig. 15.2
nAChR subunit immunochemistry in the cerebellar cortex. nAChR α4 (a and c) and α7 (b and d) subunits in autistic (c and d) compared to control (a and b). M molecular layer, p Purkinje cell layer, g granule cell layer. (Reprinted from Mukaetova-Ladinska et al. (2010, pp. 129–161). Copyright (2010) with permission of Springer Science + Business Media
Our other studies concentrated on investigating the levels of mRNA, protein expression and receptor binding densities of the various nicotinic receptor subunits in both autistic and control subjects (Figs. 15.2 and 15.3). The α4 mRNA levels, protein expression and receptor binding densities were all lower in the parietal cortices of autistic subjects than in controls (p < 0.05; Martin-Ruiz et al. 2004; Table 15.1). β2 protein expression was similarly found to be 5-fold lower in this brain area in the autistic subjects, but did not reach statistical significance. Although the cerebellar α4 mRNA levels increased, the protein expression and receptor binding densities decreased in the autistic children (p = 0.02). Levels of α7 receptor subunit binding density were also increased in the cerebellum (p < 0.05), as well as non-significant increases in mRNA levels and protein expression (Martin-Ruiz et al. 2004).


Fig. 15.3
Location of cholinergic deficits in the autistic brain. Summary of findings listed in the text. (Reprinted from Mukaetova-Ladinska et al. (2010, pp. 129–161). Copyright (2010) with permission of Springer Science + Business Media
The significance of loss of nicotinic receptors in neocortical, cerebellar, thalamic and striatal regions in adult autistic individuals (Perry et al. 2001; Lee et al. 2002; Martin-Ruiz et al. 2004; Figs. 15.2 and 15.3) is unknown. Since these receptors are widely localised pre- and post-synaptically, reduced expression of nAChR (especially α4β2 nAChR, Martin-Ruiz et al. 2004) indicate widespread nicotinic receptor dysfunction and disconnectivity. These cholinergic system abnormalities could be related to cortical cell loss or dysfunction, including changes caused by abnormal cortical neuronal morphology (for example synaptic and dendritic abnormalities, Mukaetova-Ladinska et al. 2004), similar to other developmental disorders (such as schizophrenia in which nicotinic receptors have also been implicated).
Neurotrophins mediate acetylcholine release from cholinergic neurons (Huh et al. 2008). In particular, the brain-derived nerve growth factor (BDNF) contributes to both pre- and postnatal brain development, and influences nAChR activity (Fernandes et al. 2008). This strongly argues for its association with a number of diseases associated with cognitive changes, including autism. A recent report studying autistic babies found 62 out of 64 to have increased blood levels of BDNF (Nelson et al. 2000). Furthermore, animal studies have confirmed that impairment in BDNF release at axonal level results in ‘autism-like’ behavior, with profound deficits in social and anxiety-related behaviors (Sadakata et al. 2012). However, the BDNF measurements in the human autistic brain tissue are not conclusive. Thus, both increase in BDNF-immunoreactivity in the fusiform gyrus (Garcia et al. 2012), and decrease in BDNF cortical concentration (Sheikh et al. 2010) in autistic children have been reported. These neurobiochemical measures closely follow the inconclusive pattern of peripheral blood BDNF measures in ASD subjects (Hashimoto et al. 2006; Nelson et al. 2006; Katoh-Semba et al. 2007; Croen et al. 2008; Abdallah et al. 2012). Interestingly, no changes in the nerve growth factor (NGF) were noted in the postmortem study (Perry et al. 2001). Since neurotrophic factors regulate the expression of nicotinic receptors (e.g α7 nAChR; Massey et al. 2006), more detailed studies are warranted to address the brain and the peripheral BDNF content in ASD.
A recent study also implicated the neurexin-1β dysfunction in relation to the α4β2 synaptic targeting to the presynaptic neuronal terminals (Cheng et al. 2009), thus further providing support for the role of altered synaptic changes in ASD. Interestingly, the β2-containing nAChRs have been shown to regulate executive and social behaviours (as demonstrated in a β2 nAChR subunit knock-out mice; Granon et al. 2003), which is very similar to the significant reduction of the α4β2 nAChR expression that we have described in the parietal and the cerebellar cortex.
Since nAChRs are known to modulate the release of other neurotransmitters, e.g. GABA and glutamate (Lavine et al. 1997; Baulac et al. 2001), the deficit of nicotinic receptors may denote the early imbalance between the excitatory (glutamatergic) and inhibitory (GABAergic) interneurons in the autistic brain, and thus would increase the excitatory/inhibitory impulse ratio further, and consequently result in worsening of the clinical presentation of autism. To explore these possibilities immunohistochemical double labelling studies using antibodies to specific nicotinic receptor subunits together with GABA or glutamate markers are needed.
15.4 Cholinergic Therapies for Autism
15.4.1 Acetylcholinesterase Inhibitors (ChEI)
These cholinergic abnormalities in the adult autistic brain tissue may be relevant to the clinical phenotype of ASD, especially cognitive deficits. Augmentation of acetylcholine , therefore, may help regulate some of these deficits; acetylcholinesterase inhibitors (ChEIs) decrease the rate at which acetylcholine is broken down. Donepezil, galantamine and rivastigmine are all ChEIs, licensed for the treatment of Alzheimer’s and Parkinson’s disease dementia, and also relevant to treatment of other neuropsychiatric disorders such as Lewy Body Dementia, Down syndrome, delirium, schizophrenia, depression, mania, traumatic brain injury, attention deficit hyperactivity disorder etc. (reviewed in Yoo et al. 2007). In the case of dementia, ChEIs have been shown to improve both cognitive and behavioural changes, global function and activities of daily living (reviewed in Farlow et al. 2008).
ChEIs have been predominantly used in adults and older people. Their safety for younger people has been recently demonstrated in children with Down syndrome (DS). Heller et al. (2004) reported significant improvement of expressive and receptive language performance in 7 DS children (ages 8–13 years) in a 16 week open-label trial (Donepezil, 2.5–5 mg/day, followed by 6 weeks of washout period). These findings were confirmed when the study was extended to a 22 week open-label trial (Donepezil 2.5 mg/day and 5 mg/day; Spiridigliozzi et al. 2007). Overall, Donepezil was well tolerated; subjects had language improvement, though some of the participants exhibited increased irritability and/or assertiveness. However, a later study by Kishnani et al. (2010), also conducted on young DS subjects (10–17 years of age, with mild to moderate degree of intellectual disability) failed to replicate these findings.
Similar results of improvement of adaptive function, attention, memory and language have been reported for another ChEI, rivastigmine, in 11 DS children and adolescents (ages 10–17 years; Heller et al. 2006). However, in the follow-up study, the long-term use of rivastigmine (38 months) in the same group of DS subjects did not result in significant improvement of cognitive and language performance (Heller et al. 2010). These results, coming from a small number of participants in these trials, argue that the initial benefits of the ChEIs seen in the DS children may level off with the ageing process. However, larger controlled studies that will employ tests that will cover the performance across multiple psychological domains, including functional ability of the DS subjects are required.
Studies in young DS children treated with ChEIs did not highlight overt side effects, and were consistent with the adverse events noted for ChEIs in older adults. Thus, mild, transient, worsening of gastrointestinal functions, e.g. diarrhea, nausea and vomiting, decreased appetite and moodiness appeared with the initiation of the treatment, whereas problems with sleep, fatigue and emotional lability (e.g. rivastigmine 4.5 mg daily; Heller et al. 2006), cramps and hypotension (donepezil 10 mg daily; Heller et al. 2004) were transient late adverse effects, associated with increasing ChEI dose and resolved within few days.
15.4.2 ChEIs Treatment in Autism
The studies of the use of ChEIs in DS confirmed the good tolerability of these drugs in children, and their potential in regulating some of the similar behavioral and cognitive symptoms in DS and ASD. Over the last decades, all 3 ChEIs have been used in behavioral and cognitive studies in both animal models of autism, as well as children and adolescents with a clinical diagnosis of ASD (Table 15.2).
Table 15.2
Review of studies using cholinesterase inhibitors for treatment of ASD subjects. All currently published studies on use of ChEIs (donepezil, rivastigmine and galantamine) in ASD reviewed
ChEI | Study | Trial/Subjects | Criteria for Diagnosis | Main Findings |
---|---|---|---|---|
Donepezil | Hardan and Haden 2002 | 8 autistic children and adolescents (7–19 years) Open trial | DSM-IV | 50 % of subjects had improvement on ABC and Clinical Global impression scale |
Chez et al. 2003 | 43 children with autism or pervasive developmental disorder. Double blind study over first 6 weeks, followed by open labelled 6 weeks study | DSM-IV | Improvements in receptive and expressive language scores, using CARS | |
Hetrzman 2003 | 1 adult treated with 5 mg/day donepezil | DSM-IV | Verbal and behavioural regression after 1 month of treatment. | |
Handen et al. 2011 | 34 children (8–17 years) with autism (IQ > 75), treated with 5–10 mg donepezil. Double-blind, placebo-controlled trial over 10 weeks, followed by a 10-week open label trial for placebo non-responders | DSM-IV | Improvement in a number of executive function measures, but no statistically significant between-group differences were found | |
Srivastava et al, 2011 | A 5 years old boy (IQ90) treated with donepezil 5mg as an add-on therapy to risperidone 0.5mg Open trial | ICD-10 | CARS score improved from 50 to 38 within 6 weeks | |
Buckley et al, 2011 | 5 boys (2.5–6.9yeras) Open label trial, 1.25mg donepezil titrated to 5mg | DSM-IV | Significant decrease in REM latency and increase in REM sleep) (p=0.02) | |
Rivastigmine | Chez et al, 2004 | Open labelled study. 32 children (unspecified age) | DSM-IV | Improvements in autistic behaviour, particularly verbalization. Assessments done using Childhood Autistic Rating Scale, Gardner’s Expressive and Receptive One- Word Picture Vocabulary tests, and the Conners’ Parent Rating Scale. |
Galantamine | Niederhofer et al, 2002 | 20 boys (mean age 7.4 years). Dose and duration of treatment not specified. | ICD-10 | Improvements in hyperactivity, inadequate eye contact and inappropriate speech. ABC used. |
Hertzman 2003 | 3 autistic adults treated with 4mg/day of galantamine, the dose increased to 12mg/ day after 2 months. | DSM IV | Improvement in expressive language and communication | |
Nicolson et al, 2006 | 13 children (mean age 8.8 years). 12 weeks open-label trial | DSM-IV | 61.5 % children responded. Improvements in irritability, social withdrawal, emotional lability, inattention and aggression. ABC, Conner’s Parent Rating Scale-Revised, Children’s Psychiatric Rating Scale and Clinical Global Impressions Scale used. | |
Cubells et al, 2011 | 39 year male with 15q13.3 deletion syndrome (IQ=55). Galantamine initiated 4mg daily, and dose titrated over 2 weeks to 12mg bid, and followed over 10 months period. | DSM-IV | Dramatic decline in frequency and intensity of rage outbursts |
15.4.2.1 Donepezil
Donepezil inhibits acetylcholinesterase activity and improves memory and spatial learning. In a recent animal study, an intraperitonal injection of donepezil in a mouse model of autism (BTBR mice) resulted in significant improvement of autism-relevant phenotypes, including decreasing cognitive rigidity, improving social preference, and enhancing social interaction, whereas when injected in the dorsomedial striatum, donepezil improved cognitive-rigidity and social-deficiency phenotypes (Karvat and Kimchi 2013). These findings provide further evidence for the key role of the cholinergic system in ASD, and suggest that increased cognitive flexibility, as a result of acetylcholine augmentation, may result in enhanced social attention .
There are few studies assessing the value of treating autistic subjects with donepezil (Table 15.2). Hardan and Handen (2002) studied the effects of donepezil on eight autistic children and adolescents (7–19 years age). All of the patients were diagnosed using DSM-IV criteria and underwent an open-label trial with donepezil, to assess benefits on their behaviour. Four of them showed improvements in their irritability and hyperactivity; however, assessments of their cognitive functioning and memory were not conducted. Only two side effects were reported: one patient had gastrointestinal problems and another one a mild increase in irritability. Chez et al. (2003) conducted a 6 week double blind study with donepezil, followed by a 6 week open trial with donepezil on a group of 43 children with autism (2–10 years of age) or pervasive developmental disorder. After 6 weeks, the donepezil treated group showed, improvements in overall autistic behaviour, and receptive and expressive language compared to the placebo group.
Although these two studies suggest that donepezil may be effective in treating children and adolescents with autism, the results are not conclusive and more double blind placebo studies need to be carried out. A recent study also dealt with use of donepezil in regulation of Rapid Eye Movement (REM) sleep disorder in young children with autism (Buckley et al. 2011). Since REM sleep duration is greatest in the developing brain and represents the privileged time for neuroplasticity, REM sleep augmentation in children with autism may also have an impact on their cognition and behaviour. This warrants further studies.
15.4.2.2 Galantamine
Besides being a ChEI, galantamine has also allosteric nicotinic receptor modulatory effect which could be particularly relevant in autism in view of nicotinic receptor pathology. As with donepezil, there are few studies of galantamine and autistic patients (Table 15.2). A placebo controlled, double blind crossover, randomized trial with galantamine was conducted by Niederhofer et al. in 2002. Twenty boys (mean age = 7.4 years) with autistic spectrum disorder, diagnosed by ICD-10 criteria, were treated with placebo or galantamine. On average, the subjects receiving the placebo scored slightly higher than the subjects receiving galantamine. Improvements were seen in hyperactivity, inadequate eye contact and inappropriate speech. Clinicians’ scores were not significantly different between the placebo and galantamine groups. No side effects were reported but most subjects had very limited language capacities. The study concluded that galantamine may be moderately effective in the short term treatment of irritability in autism.
In another study, galantamine improved verbal skills in 3 autistic adults (Hertzman 2003). Each subject exhibited an improvement in verbalization, and in some, social behavior improved. Only one person experienced side effects. An open label trial by Nicolson et al. (2006), evaluated the use of galantamine with thirteen children (mean age = 8.8 years) with autism. Eight of the thirteen children (61.5 %) benefited from treatment with improvements in irritability, social withdrawal, emotional liability, inattention and aggression. There were no side effects reported except headaches in one patient.
15.4.2.3 Rivastigmine
Only one open label study on use of rivastigmine in autism has been published (Chez et al. 2004; Table 15.2). 32 autistic children (unspecified ages) were recruited to take part in a 12 week open label trial, and repeatedly tested at baseline, 6 and 12 weeks. Improvements were seen with regards to autistic behaviour, particularly in verbalization.
15.4.2.4 Combined Use of ChEI with Neuroleptic and Antidepressant Medications
Although the successes of most pharmacological treatments for autistic adults are limited, combining medications may prove to be more effective. Studies on the joint use of ChEIs and neuroleptic medications in adults with schizophrenia are encouraging. Thus adjunctive treatment with donepezil improves cognition in patients with schizophrenia who are stabilized on atypical antipsychotics (Chung et al. 2009). The most recent meta-analysis, based on six open-label and 24 double-blind randomized controlled studies reported that donepezil, rivastigmine and galantamine adjunctive therapy improve specific cognitive deficits, such as memory, motor speed and attentional aspect of executive function in patients with schizophrenia and schizoaffective disorder (Ribeiz et al. 2010). Similar adjunctive treatment approach was reported recently by Srivastava et al. (2011) in a child with autism. In their case report, they discuss the management of behavioral problems (e.g. hyperactivity) in a 5 years old boy with autism, who was initially treated with Risperidone 0.5 mg for 8 weeks with no improvement. The add-on treatment with donepezil (5 mg daily) resulted in significant improvement of his behavior within the next 6 weeks that was characterized as better verbal communication, eye contact and emotional response, as well as decreased hyperactivity. Similar results were recently confirmed in a randomized control study on 40 children with autism (age 4–12 years) in which the add-on therapy of galantamine to risperidone improved irritability, lethargy and social withdrawl (Ghaleiha et al. 2013). This suggests that the adjunctive treatment with ChEIs may find a place in the treatment of various behavioral and cognitive changes even in young children. However these findings need to be replicated in larger studies. Furthermore, this approach can potentially be extended to concomitant use of ChEIs and antidepressant medications, e.g. SSRIs, similar to that used in elderly with Alzheimer’s disease, which had improvements in both cognitive functioning and functions of daily living (Mowla et al. 2007).
15.4.3 Treatment of Autism with Nicotinic Agonists or Antagonists
15.4.3.1 Nicotinic Agonists
In clinical studies, nicotine has been documented to ameliorate some of autistic spectrum symptomatology. Nicotine-agonists (Gaynor and Handley 2001) and nicotine patches improved attention and reduced complex tics in autistic subjects with Tourette’s syndrome (Howson et al. 2004). Most recently we also reported on the benefits of cholinomimetic therapy (e.g. nicotine patch) on a vast range of behavioral problems (e.g. hyperactivity, behavioral dysfunction, impulsivity, restlessness and aggression, and a pronounced sleep disorder) in a 22-year old adult with autism who had profound mental disability (Mukaetova-Ladinska et al. 2012). Interestingly, even the use of a single nicotine patch produced a significant reduction of the symptomatology with an average duration of 1–2 weeks post-application (Shytle et al. 1998), whereas prolonged use of the patch also contributes to discontinuation of adjunctive medication, including neuroleptics and antidepressants (Mukaetova-Ladinska et al. 2012). This effect is not mediated via the tryptophan metabolism and/or its catabolites (Gaynor and Handley 2001).
15.4.3.2 Nicotinic Cholinergic Antagonists
Treatment with nicotinic cholinergic antagonists may also alleviate autistic symptoms (Lippiello 2005). The loss of nicotinic receptors from the frontal, cerebellar and thalamic cortices (summarized in Table 15.1) in autistic adults may be a compensatory measure (or a result of negative feedback), for an excess of cholinergic neurons found in the enlarged young autistics’ basal forebrain (Baumann and Kemper 1994). Treatment with a nicotinic antagonist could potentially reduce the effects of the excess cholinergic neurons, preventing the reduction of nicotinic receptors in other parts of the brain.
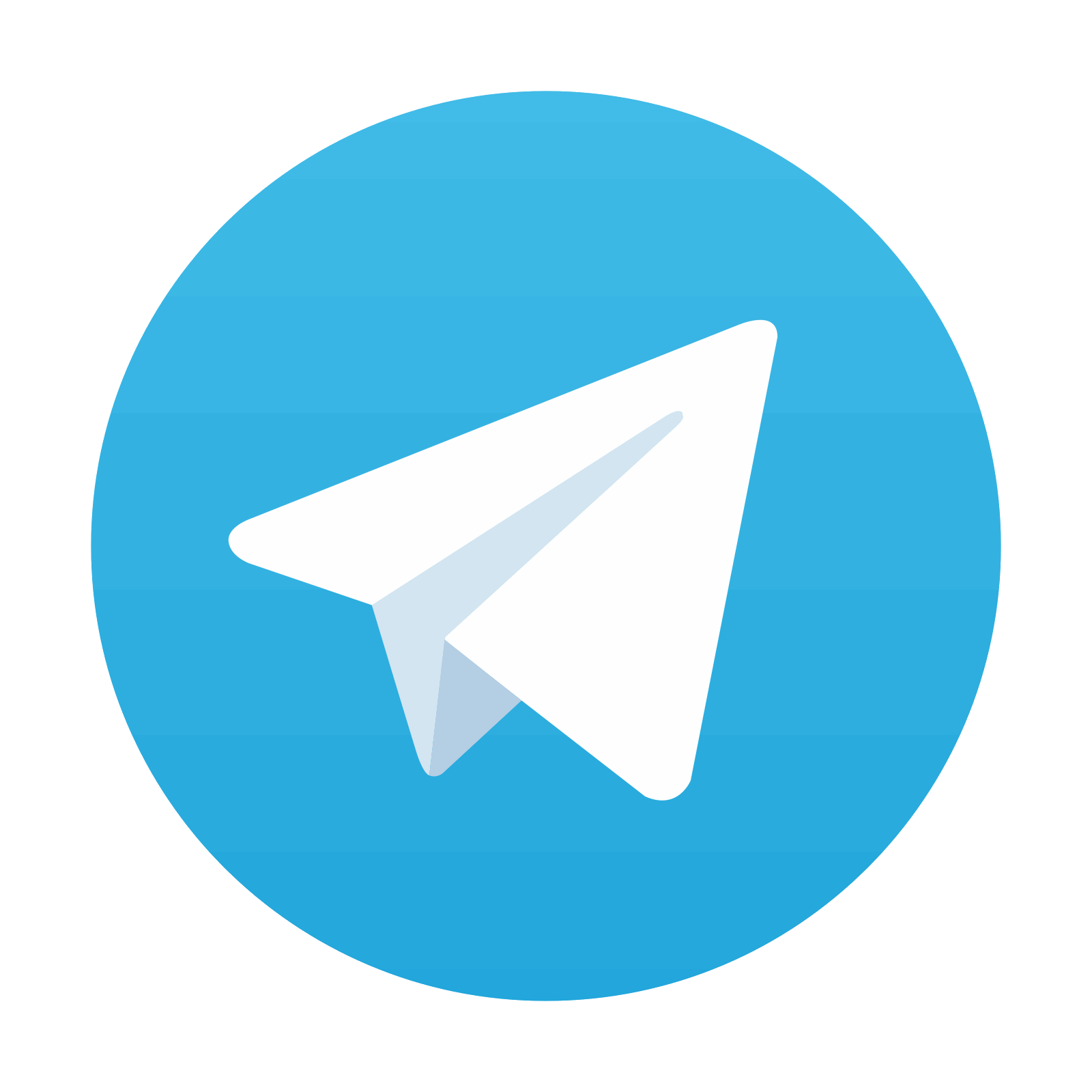
Stay updated, free articles. Join our Telegram channel

Full access? Get Clinical Tree
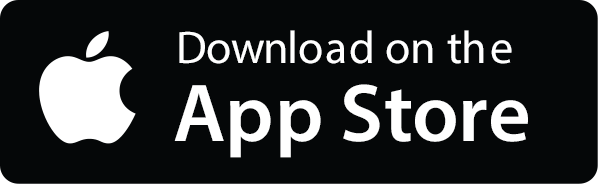
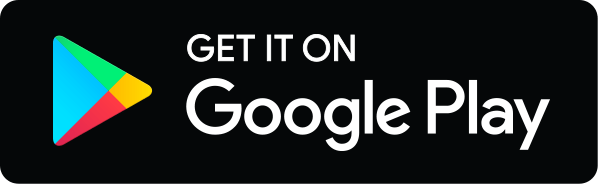