Chordomas are rare, slow-growing, but invasive, destructive, and metastatic malignant bone tumors of the axial skeleton. Despite their rarity, extreme challenges in treatment have kept chordomas constantly in focus. As the suffix “oma” denotes, chordomas have a differentiated morphological phenotype and on a cursory look may not appear overtly malignant under the microscope. However, this morphological phenotype obscures malignant clinical behavior. Chordomas have an indolent but relentless course and are challenging to treat. This is because of their frequent placement in the central skull base. Their invasive growth pattern in this delicate and intricate anatomy makes surgical resection of chordomas very challenging. In addition to these difficulties in surgery, the treatment of chordomas is further complicated by their poor response to the currently available adjuvant treatments. Most patients succumb to progressive tumor growth despite multimodal treatment. Nevertheless, there is variation in tumor behavior: some chordomas grow aggressively despite multimodal treatment protocols, finally to result in the patient’s demise, whereas other tumors grow slowly providing the patient many good years of life. Determinants of this difference in behavior are not known, but they are assumed to depend on the intrinsic biology of chordomas. Much has been learned on the biology of chordomas but the etiology of chordomas is still not known. There are no known predisposing conditions and no environmental factors that are associated with chordomas. Chordomas are thought to be developmental tumors related to the notochord. This hypothesis on notochordal origin was suggested very early based on histologic resemblance, and today it is supported by further compelling scientific evidence. Chordomas arise along the axial skeleton anywhere from the visceral cranium to the sacrococcygeal bone. Histopathologically, they are characterized by clusters of large vacuolated cells, which are called physaliphorous cells. These clumps of low cellularity are separated by fibrous septa and embedded in a basophilic extracellular matrix. This bears close histologic resemblance to notochordal cell remnants. 1 The notochord plays an important role in the development of the axial skeleton in the embryonic life and regresses after birth to leave cellular remnants located within the nucleus pulposus. Yamaguchi et al 2 have demonstrated that these remnants are co-localized with chordomas and that there were electron microscopical, histologic, and immunohistochemical resemblances and suggested that chordomas may arise from transformation of these notochordal remnants. Similarly, ecchordosis physaliphora, which are benign, non-proliferative notochordal cell remnants, have been suggested to represent an intermediate step in this transformation from notochordal cell remnants to chordoma. The first description of chordoma and its characteristic “physaliphorous cells” was given by Virchow in 1846 and published in 1857. 3 Virchow hypothesized that these tumors derived from cartilage, and speculating a possible relationship to other cartilaginous tumors, he named the lesion “ecchondrosis physaliphora spheno-occipitalis.” 3 Virchow’s detailed descriptions of the entity in his book in 1857 were then followed by further observations by Luschka 4 and Zenker 5 in the same year. In the following years, Kölliker 6 demonstrated that the mammalian nucleus pulposus derived from the notochord. Subsequently in 1858, Heinrich Müller 7 suggested a possible origin of chordomas from the primitive notochord and induced a nomenclature change to “ecchordosis physaliphora.” These ideas caused much controversy in morphological sciences and caused a division among the pathologists, with some calling these “jelly tumors” neoplasia, others developmental abnormalities. Strong support for the notochordal origin hypothesis came from Ribbert. It was also him who first used the term “chordoma”. In 1894, by puncturing the anterior intervertebral ligament in rabbits, Ribbert successfully produced experimental chordomas and by doing so, proved them as developmental tumors. 8,9 In his laboratory, Fischer and Steiner 10 confirmed Müller’s theory by creating a malignant chordoma. In 1909, Linck 11 for the first time established criteria for the histopathological diagnosis of chordoma and defined formation of mucin, the presence of physaliphorous cells, a lobular arrangement, nuclear vacuolization, and resemblance of notochordal tissue as characteristic findings. In 1920, Alezais and Peyron 12 gave a detailed description of histogenesis and evolution of chordomas. Stewart and Morin 13 were the first to suggest that chordomas may derive from ecchordosis physaliphora. Zülch and Christensen 14 in 1956 noted that different chordomas did exhibit a wide spectrum of clinical behaviors, and distinguished between slowly growing benign chordomas and rapidly growing malignant chordomas. Heffelfinger et al, 15 after analyzing 155 cases of chordoma and all other cartilaginous tumors treated at Mayo clinic from 1910 to 1973, described a “chondroid” subtype in 1973. One hundred and fifty years of accumulating morphological and experimental data on chordoma biology was supported in the 2010s by sophisticated molecular biology techniques, which brought a new understanding of chordoma biology. Today we know that the major determinant of the disease course of a chordoma is its intrinsic biology. Current treatment modalities including surgery and radiation therapy, irrespective of how aggressive or powerful they may be, only modify the disease course, but do not significantly alter the intrinsic biology of the tumor. Only a better understanding of the tumor biology can show us the Achilles’ heel, and this review is aimed at summarizing current knowledge on chordomas. The notochord is a rod-shaped body that defines the primitive axis of the embryo. 16,17,18 In higher vertebrates, the notochord exists transiently during embryogenesis and provides position and fate information to surrounding mesenchymal structures. 16,18 The human notochord originates from mesoderm during the third week of embryogenesis. 16 “Nodal” signaling in the bilayered embryo induces mesoderm formation. Chordamesoderm, which is the precursor of the notochord, develops at the same time point through less prominent Nodal signaling. When formed, the notochord serves two main functions: it acts as the axial skeleton of the embryo and provides structural support until the formation of other structures such as the vertebral bodies. Without a fully developed notochord, embryos fail to elongate. 17,18 But the notochord also has a modulatory role in the formation of the axial skeleton via sonic hedgehog and bone morphogenic protein. The notochord likely represents a primitive relative of cartilage and shares many characteristics with common cartilage tissues, such as expression of type II and type IX collagens, aggrecan, Sox9, and chondromodulin. 19 Chondrocytes normally secrete a highly hydrated extracellular matrix, which gives cartilage its main structural properties. 20 Notochord cells, instead of secreting these hydrated materials into the extracellular matrix, retain them in large cellular vacuoles, which allow notochordal cells to exert pressure against the thick basement membrane and create the shape, turgor, and structure of the notochord. 17 The cranial extension of this embryonic structure extends as far as the sella turcica, and some authors claim that the adenohypophysis limits the rostral elongation of the notochord. 21 During the development of the skull base, the notochord is incorporated into the caudal part of the sphenoid and the basilar part of the occipital bone. 22,23,24 The ossification process is similar in the skull base and the axial skeleton. Ultimately, the notochord that runs through the middle of each vertebra is dismantled and replaced by bone. This ossification process is also very similar to enchondral bone formation that occurs in the cartilage and involves deposition of type X collagen into the collagen II–rich extracellular matrix (ECM) to result in ossification. 25 In contrast, in the intervertebral space, the notochord does not express collagen X and eventually expands to form the nucleus pulposus. 20,26,27 At the rostral end, the notochord bends towards the pharyngeal wall and forms secondary unions with the pharyngeal epithelium to result in development pharyngeal bursa ventral to the sphenooccipital synchondrosis. 21 Embryological rests of this process, benign chordal ectopias (ecchordosis physaliphora), are commonly encountered in the skull base or in the prepontine area. In the mobile spine, within vertebral bodies these lesions are called “benign notochordal cell tumors” of the spine. Autopsy series reported incidences of 0.5 to 2%. 28 Mehnert et al 29 found them in 1.7% of the subjects in a review of 300 magnetic resonance imaging (MRI) studies of the skull base. These lesions are distinct and represent an intermediate form between the normal notochord and chordomas. The leading hypothesis on the formation of chordomas is based on the assumption that these benign chordal ectopias are the precursors of chordomas. 2,30,31,32,33 This hypothesis dates back as early as 1926. 13 Supporters of the hypothesis proposed a progressive tumorigenesis from benign notochordal remnants to these benign tumoral intermediates to chordoma. Others advocated a de novo origin from notochordal remnants. The hypothesis on the origin of chordomas from premalignant lesions (ecchordosis physaliphora) is supported by similar morphology (light microscopy and electron microscopy), 34,35,36 similar immunophenotype, 35,37,38 and similar localization. 2,30,31,32,33,35 Cell fate tracking experiments in mice showed that the notochordal cell rests localize along the axial skeleton, where chordomas are most frequently seen. 39 Perhaps the strongest evidence comes from molecular studies, which demonstrated corresponding molecular changes in ecchordosis physaliphora and chordomas. 40,41 In addition to pointing out its precursor lesions, studies on the biology of chordomas have also helped to differentiate chordomas from tumors with similar morphological features. The most striking example for this is chondrosarcoma. Low-grade chondrosarcomas and chordomas can occur at the same anatomical site, and exhibit similar imaging and histological appearances and therefore have traditionally been analyzed together in clinical case series to create sufficiently large, artificial cohorts. 42 Today there is compelling evidence that chondrosarcomas have a more benign and predictable tumor biology and therapeutic response when compared with chordomas. Low-grade chondrosarcomas respond much better to surgical resection and radiation therapy and recur less frequently. 42 In selected cohorts, more than 99% of patients with chondrosarcomas were still alive 10 years after diagnosis. 43 Chondrosarcoma patients also fare much better than patients with chordoma in terms of median- and long-term survival. 42 Therefore, it is widely accepted today that chordomas and chondrosarcomas are distinct tumor entities with distinct biology. In addition to morphology, two molecular markers also aid in the differential diagnosis of chordomas and chondrosarcomas: As will be discussed below in detail, nuclear T gene (brachyury) expression is present in chordomas but not chondrosarcomas or other soft tissue tumors of the skull base. 44,45,46,47 Another very useful marker for differentiating chondrosarcomas is the isocitrate dehydrogenase-1 (IDH1) mutation, which was found positive in of the cases. 48,49 The most frequent mutation in these cases is the IDH1-R132. This specific mutation cannot be detected using the routine IDH1-R132H immunohistochemistry, which is commonly used to classify low-grade gliomas but can be simply and reliably demonstrated using molecular studies. 45,48,49,50 Strongest findings on pathogenetic mechanisms in chordomas were provided by genetic studies in familial chordomas, which showed associations with 1p36, 7q33, and the embryonic transcription factor T (brachyury). Familial chordomas are exceptionally rare and make up less than 0.5% of all chordomas. 51 In total, less than 40 cases have been reported in the literature so far. 51,52,53,54,55,56,57,58,59,60,61,62 However, such familial occurrence indicate common genetic alterations, which have been studied by various groups so far. In 1958, Foote et al 63 reported the familial occurrence of chordoma for the first time in a brother and sister, who presented with metastatic sacrococcygeal chordomas. This was flowed by a report of two brothers with “nasopharyngeal” chordoma, 54 a man with nasopharyngeal chordoma whose mother and daughter developed the same tumor, 57 a man with sacrococcygeal chordoma whose sister and niece developed clival chordomas and whose first cousin had a nasopharyngeal chordoma, 59 and a father and daughter with clival chordoma. 53,58 In the genetic analysis of one family, Stepanek et al 59 reported an autosomal dominant inheritance pattern. This was followed by a genome-wide linkage analysis in an extended pedigree of 10 affected individuals of the same family, and the defect was localized to a “minimum disease gene region” of 7.1 Mb at 7q33. 55 In a later study the authors reconfirmed this disease region in the same three families by linkage analysis, but they were unable to detect the same changes in a fourth family. 61 Dalprà et al 53 reported a father and a daughter with recurrent chordoma. Another daughter also had a cerebellar astrocytoma. Cytogenetic analysis revealed pronounced heterogeneity of the karyotypes, with a number of unbalanced translocations leading to 1p losses. 53,58,64 An analysis of this family and several other sporadic cases mapped the genetic defect to a 25 cM long segment between 1p36.31 and 1p36.13 and possibly involving a tumor suppressor gene. 58 However, the most dramatic finding was the discovery of the expression of T gene (brachyury) in sporadic chordomas which led to review of the familial genetic profiles 41,53,58,64 and the finding of germline duplication of the T transcription factor, which is located at 6q27, in members of 4 families with familial chordoma. 62 The discovery of the T gene duplication in familial chordomas and the expression of brachyury in all chordomas, other than the dedifferentiated variant, was an exciting finding. This is because the T gene (brachyury) has a significant role in the development of the notochord. 16,65,66,67 Since then, the T gene has been shown to play an important role in germ cell tumors, and in carcinomas where it was reported to promote epithelial–mesenchymal transition in human tumor cells. 68,69 Brachyury is a transcription factor and in normal physiology is almost exclusively expressed during embryogenesis. In adult tissues, the expression is confined to the testes. Among bone and cartilage tumors, immunopositivity for brachyury is fairly specific for chordomas: among cranial tumors, brachyury-immunoreactivity is only seen in chordomas and hemangioblastomas. The absence of brachyury expression in chondrosarcomas aids in the differential diagnosis. In familial chordomas, the pathologic process underlying brachyury expression is a germline alteration and most commonly caused by amplification. In sporadic cases of chordoma, somatic gain of T was observed in other contexts, but nevertheless copy number gain of the T gene was a common event in both familial and sporadic chordomas. In an analysis of 181 patients utilizing combination of fluorescence in situ hybridization (FISH) and array comparative genomic hybridization (CGH), Presneau et al 70 reported amplification in 7% of the cases, polysomy of chromosome 6 in 39%, and minor allelic gain in 4.5% of cases. The changes were reported to be somatic and were not observed in non-neoplastic tissue of 40 patients. 70 FISH experiments also showed that gain of the T locus was not uniformly present in all cells. 70 It is noteworthy that gain of T was uncommon if a comprehensive search for it was not performed. Le et al 71 reported no amplification of the T gene in 20 sporadic chordomas although minor allelic gain (3N and 4N) was noted in 12.5% of the cases. Shalaby et al 72 failed to show any mutations (substitutions) by sequencing. 72 Expression of brachyury is observed in all sporadic chordomas, other than the dedifferentiated chordomas. Vujovic et al 41 demonstrated that all of 23 studied tumor samples expressed T, as determined on quantitative polymerase chain reaction (qPCR) analysis, regardless of whether the 6q27 locus was amplified or not. Using in vitro studies, the same study also showed that the knockdown of the T gene by RNA interference in U-Ch1 chordoma cell-lines, which are polysomic in 6q27, resulted in a senescence-like phenotype with growth arrest, and morphological changes. The same finding (phenotypic changes and loss of proliferation upon T silencing) was confirmed in a second sacral chordoma cell line CHJ-7. 73 In further support of the central role of brachyury in chordoma pathogenesis, Pillay et al 74 have shown that occurrence of chordomas is strongly associated with the common single-nucleotide polymorphism (SNP) rs2305089. This SNP is located within the coding region of the T gene and is associated with an exceptionally high odds ratio of 5.3 (p = 4.6 × 10-12) for development of chordomas. Others had previously shown that this polymorphism has functional consequences alters the binding ability of the T gene, and Pillay et al demonstrated that the homozygous rather than the heterozygous SNP alters more significantly brachyury expression levels and the levels of its downstream targets in chordoma. 74,75The finding of the extremely strong association of the T rs2305089 SNP in patients with chordoma appears to be at odds with the rarity of chordoma in the population. It has therefore been proposed that the SNP rs2305089 is a requirement for the development of notochordal rests, which as shown by Yamaguchi are common in the population, and that transformation to a benign notochordal tumor and chordoma requires acquisition of additional genetic alterations. Nelson et al 76 showed that the T-box binding motif of human T gene was identical to that found in mouse, Xenopus, and zebrafish development, and that T acted as an activator of transcription. The transcriptional targets of T included 99 direct genes as well as 64 other genes, expression of which was regulated by indirect influences. Hotta et al 66 extended this network to 450 nonredundant genes downstream of brachyury. These findings established brachyury T as a master regulator of an elaborate oncogenic transcriptional network encompassing diverse signaling pathways, including WNT/PCP, NF-κB, and TGF-β signaling to regulate the cell cycle, and extracellular matrix components. 14, 15, 16, 64, 65, 73 Conversely, brachyury function is regulated by upstream intracellular signaling mechanisms, and this may be the mode of T activation in tumors that have no genetic alteration in the T gene. Using a candidate gene approach, several authors have focused on receptor tyrosine kinase (RTK) activation, which in turn can activate brachyury signaling. 77,78,79,80,81,82,83,84,85 Fig. 4.1 Experimental studies have shown that Brachyury plays a central role in chordoma biology. Compelling evidence for this central role came from translational studies that have demonstrated various genetic mechanisms that lead to brachyury overexpression in familial as well as in sporadic chordomas. Further experimental studies have provided evidence that brachyury is a master transcriptional activator which directly increases the expression of 99 target genes, which are involved in key cellular signaling cascades and result in significant effects on extracellular matrix as well as cell cycle regulation. Studies have shown that 60 to 69% of skull-based chordomas express EGFR and that 40% express it in its phosphorylated form, indicating that the EGFR/PI3K/AKT pathway is a common event in chordomas. 81,83,86 Although it is observed in a fraction of cases, amplification of chromosome 7 is a recurring event in chordomas. 71,87,88,89,90 When specifically analyzed for EGFR-related genetic changes, chordomas display high-level EGFR polysomy in 38%, high-level polysomy with focal amplification in 4%, low-level polysomy in 18%, and disomy in 39%. 81 No activating mutations have been demonstrated to date. Immunohistochemical studies have demonstrated EGFR expression and phosphorylated EGFR expression in chordomas. 80,81,82,83,84,85,91,92,93,94 Another upstream regulator of brachyury is fibroblast growth factor (FGF), which acts through the Ras/Raf/MEK/ERK cascade. The hypothesis that FGF deregulation might increase brachyury signaling in chordomas was based on by the observation that at least one form of FGF was expressed in 47 of the 50 chordomas. 72 The FGFR receptors are linked via the FRS2-α linker to the RAS/RAF/MEK/ERK pathway. However, Shalaby et al 72 sequenced the promoter region and the coding region of T, FGFR1–4, and KRAS (codons 12, 13, 51, 61) and BRAF (exons 11 and 15) but failed to show any genetic alterations in 23 chordomas. Another study also confirmed the absence of KRAS and BRAF mutations. 71 No direct regulatory effect of FGFR on downstream effectors could be demonstrated in chordomas. 72 Contrary to this finding, Hu et al 95 also have analyzed the FGFR/MEK/ERK/brachyury signaling pathway and reported that inhibition of FGF2 signaling resulted in decreased phosphorylation of the MEK/ERK and decreased expression of T and that this correlated with increased apoptosis and decreased cell growth. Activation of other RTKs are also observed in chordomas, but their role in T induction is not known. One of the most consistently activated RTKs is platelet-derived growth factor (PDGF). 77,79,80,83,84,96 The activation, as in many other cancers, follows the autocrine activation loop pattern, where both the ligand and the receptor are synthesized by the tumor. Both PDGFB and PDGFBR are expressed in most chordomas. 77,79,80,84 These alterations related to brachyury have also made their way into individual therapeutic efforts and also clinical trials. A randomized, double-blind, phase 2 trial of GI-6301 (yeast brachyury vaccine) (clinical trial identifier NCT02383498) was started for locally advanced, unresectable chordoma at the National Institutes of Health (NIH). Also, a phase 2 trial of PDGF inhibitor nilotinib in conjunction with radiotherapy for high-risk disease is currently ongoing at Massachusetts General Hospital (clinical trial identifier NCT01407198). There are also other cellular signaling changes related to brachyury that have been studied in chordomas. The T gene was shown to be a transcriptional target of TP63, which is known to play a central role in formation of the epidermis and other stratified epithelia and play a role in several cancers. Cho et al 97 showed that the ΔN-TP63 isoform was coexpressed at high levels in murine osteosarcoma cell lines and that its knockdown resulted in decreased T levels and decreased proliferation, migration, and invasion, and the authors claimed a functional link between TP63 and T. However, in an analysis of 50 chordomas, TP63 immunoreactivity was not found in any cases. 98 The same study showed that the pan p63 transcript was present but the ΔN-TP63 transcript was absent in 26 chordoma samples. 98 The canonical WNT/β-catenin pathway is a downstream target of T. 16 β-Catenin is involved in skull base development, and it is a common alteration in other cancers. 16,99,100,101 Although these properties make the WNT/β-catenin pathway an appealing candidate for chordoma oncogenesis, there is no experimental finding to indicate pathogenic activation of this pathway in chordomas. Copy number loss of β-catenin (CTNNB1) is commonly observed in chordomas, but only a few point mutations were documented and none of these reported mutations result in a constitutively active protein variant. Other studies have failed to show alterations in cadherin–catenin protein expression in chordomas. 99,100,101 Studies of the molecular biology of chordomas started with G-banding experiments. These early cytogenetic studies, which utilized classical cytogenetic techniques, have reported low incidence of abnormal karyotypes in chordomas and led to the early conclusion that chromosomal instability was a late event in chordoma oncogenesis. In these studies, up to 65% of the studied chordomas had normal karyotypes and the majority of the abnormal ones were hypodiploid or near diploid. 102 The remaining cases displayed very diverse chromosome aberrations. Modern whole-genome technologies such as array CGH and whole-genome SNP arrays have consistently shown that copy number alterations are very common in chordomas and that genomic instability is a common event. 70,71,88,103,104,105,106 The underlying mechanism resulting in this chromosomal instability in chordomas is unknown.
4.2 Early Studies on Chordoma Biology
4.3 The Notochordal Origin Hypothesis
4.4 Genetic Findings in Familial Chordomas
4.5 Brachyury in Chordoma Oncogenesis
4.6 The Chordoma Genome
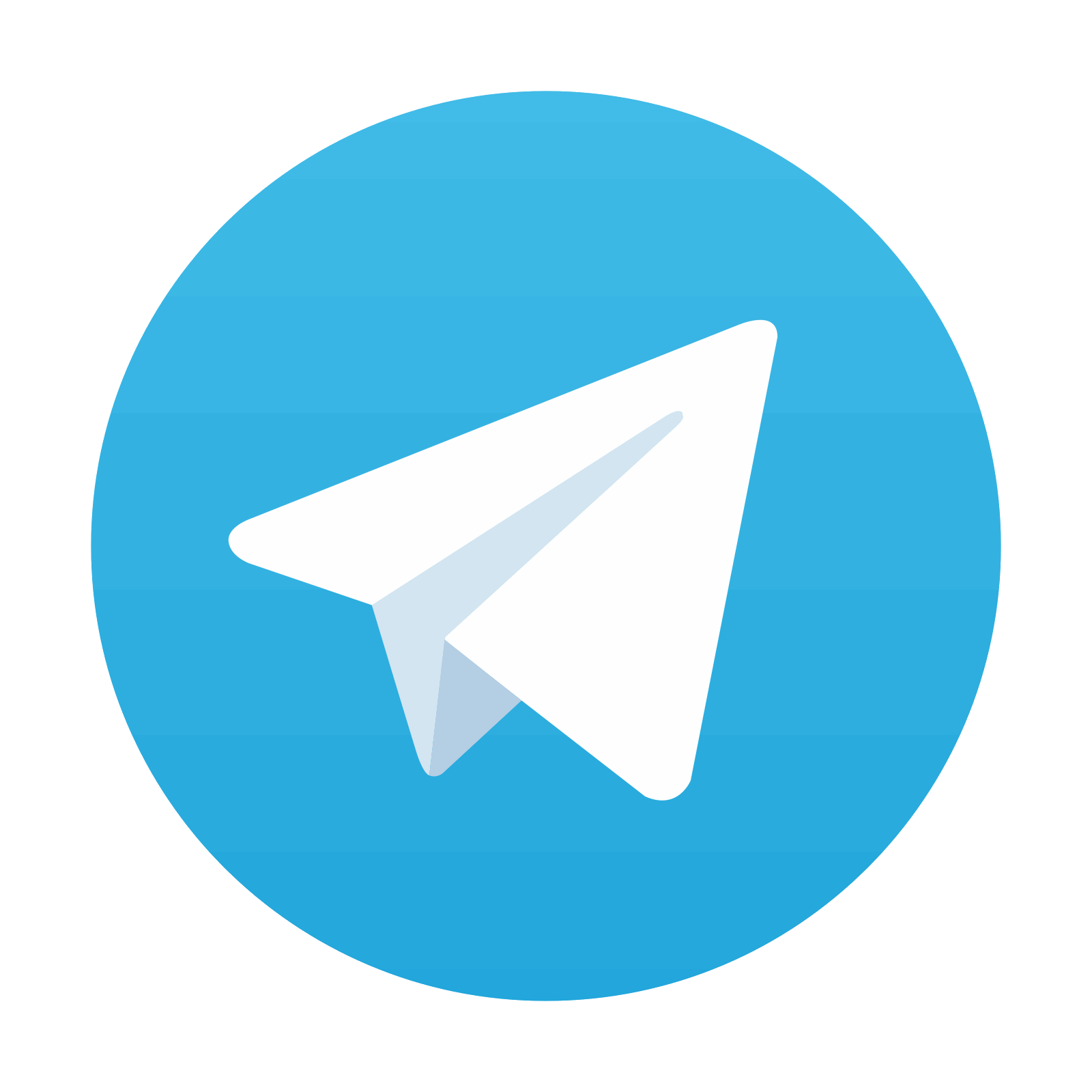
Stay updated, free articles. Join our Telegram channel

Full access? Get Clinical Tree
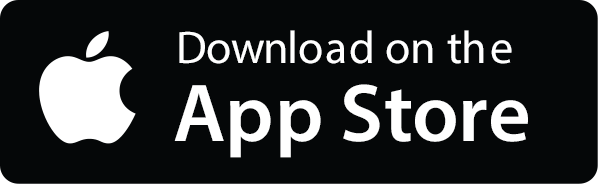
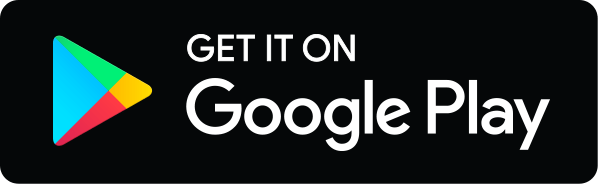