Abstract
Chondrosarcomas are a heterogeneous group of malignant cartilage-forming tumors, which are resistant to conventional chemo- and radiotherapy. Consequently, patients presenting with inoperable disease cannot be offered any alternative treatment. Therefore it is important to identify the molecular pathways that are deregulated in chondrosarcoma. Different subgroups of chondrosarcomas exist, with conventional chondrosarcoma accounting for 85% of cases, which is also the most frequently observed subtype in the skull and spine. Chondrosarcomas develop following a multistep progression model in which they step-wise acquire different mutations and pathway alterations leading to a more malignant histological phenotype. Therapeutic interference with one of these alterations is the main strategy to develop an alternative treatment for chondrosarcoma patients.
Keywords
Chondrosarcoma, COL2A1 , EXT, IDH, Indian hedgehog, p53, pRb, Signaling
Outline
Introduction 31
Clinicopathological Classification of Chondrosarcoma 31
Molecular Drivers in Chondrosarcoma 33
Central, Dedifferentiated, and Periosteal Chondrosarcoma 33
IDH1 and IDH2 Mutations 33
COL2A1 Mutations 35
Dysregulation of the Hedgehog Pathway 35
P53 and pRb Pathway Alterations in the Progression From Low to High-Grade Chondrosarcoma 35
Apoptosis and Prosurvival Pathways Implicated in Chondrosarcoma 36
Peripheral Chondrosarcoma 36
EXT1 and EXT2 Mutations in Osteochondroma and Peripheral Chondrosarcoma 36
p53 and pRb Pathway Alterations 37
Mesenchymal Chondrosarcoma: HEY1-NCOA2 Fusion 37
Clear-Cell Chondrosarcoma 37
Summary/Conclusion 37
References 38
© 2018 Elsevier Inc. All rights reserved. Please note that the copyright for the original figures submitted by the contributors is owned by Contributors.
Introduction
Chondrosarcoma is a malignant cartilage-forming tumor accounting for 20% of all malignant bone tumors. Only a small percentage of chondrosarcomas is found in the skull or spine (2%–12%). Depending on the subtype, different molecular pathways are involved in development and progression of chondrosarcoma. In the skull or spine, chondrosarcoma needs to be distinguished from chordoma, which shows a similar presentation and anatomical location, but a different biological behavior. The distinction can be made using brachyury immunohistochemistry, as overexpression of brachyury is the hallmark of chordoma. On the other hand, chondrosarcomas represent a heterogeneous group of tumors, in which different cell signaling pathways are involved in tumorigenesis. In this chapter, the most important pathways will be discussed.
Clinicopathological Classification of Chondrosarcoma
Chondrosarcomas can be divided in conventional chondrosarcoma (85%) and more rare subtypes; dedifferentiated chondrosarcoma (10%), mesenchymal chondrosarcoma (2%), clear-cell chondrosarcoma (2%), and periosteal chondrosarcoma (1%). Central conventional chondrosarcoma is the most common in the skull and spine, followed by mesenchymal chondrosarcoma.
Conventional Chondrosarcoma
Conventional chondrosarcoma can be further subdivided into central (>85%) and peripheral chondrosarcomas (∼15%) based on its location in the bone. Central chondrosarcomas develop in the bone either directly or from a benign enchondroma (see Fig. 4.1 ).

Enchondromas can develop as a solitary lesion or as multiple enchondromas in Ollier disease or Maffuci syndrome. Ollier disease and Maffuci syndrome are both rare, nonhereditary disorders caused by somatic mosaic mutations in IDH1 or IDH2 . Among solitary enchondromas ∼1% undergoes malignant transformation toward a conventional central chondrosarcoma. Enchondromas from patients with multiple enchondromas have a much higher transformation rate of ∼50%.
Peripheral chondrosarcomas develop at the surface of the bone from a preexisting benign osteochondroma. They develop from bones that are formed by endochondral ossification; consequently, they are extremely rare in the skull. Central and peripheral chondrosarcomas show similar histological features and are graded using a three-tiered scale. Atypical cartilaginous tumors (previously chondrosarcoma grade-I) show low cellularity and do not metastasize, but are locally aggressive, and local recurrences can develop. Grade-II and -III chondrosarcomas are high-grade tumors with increased cellularity and mitoses. These tumors show a higher metastatic rate and poor patient survival. Patients with atypical cartilage tumors show a 10-year overall survival rate of 83%, while patients with grade-II chondrosarcomas show a 10-year survival rate of 64% and patients with a grade-III chondrosarcoma show the poorest 10-year survival rate of 29%. So far, histological grade is the most important predictor of prognosis. Upon recurrence of low-grade tumors, progression toward a higher histological grade can occur in ∼13% of the cases, resulting in a poorer prognosis. Chondrosarcomas are resistant to conventional chemo- and radiotherapy, leaving surgical removal as the only treatment option. This has major implications for patients with inoperable disease for whom no good treatment option exists.
Rare Chondrosarcoma Subtypes
Dedifferentiated chondrosarcoma comprises ∼10% of all chondrosarcomas. It is a high-grade chondrosarcoma variant characterized by a mixed histological appearance of dedifferentiated cells and a cartilaginous component. Patients with dedifferentiated chondrosarcoma show a 5-year overall survival between 7% and 24%. Like conventional chondrosarcoma, dedifferentiated chondrosarcoma is resistant to conventional chemo- and radiotherapy. Mesenchymal chondrosarcoma is a rare high-grade chondrosarcoma consisting of differentiated cartilage mixed with undifferentiated small round cells. The 10-year survival rate ranges between 27% and 67%. The small, round cell component of mesenchymal chondrosarcoma may respond better to chemotherapy than do other chondrosarcoma subtypes. Clear-cell chondrosarcoma is a rare low-grade chondrosarcoma mainly found in the epiphysis of the humoral or femoral head. Histologically, clear-cell chondrosarcoma shows malignant cells with clear, empty cytoplasm surrounded by hyaline cartilaginous matrix. Surgery is the only treatment option for patients with clear-cell chondrosarcoma. The prognosis is relatively good, with a mortality rate of 15%. Periosteal chondrosarcoma is the rarest chondrosarcoma subtype; it accounts for less than 1% of the cases and originates from the periosteum.
Molecular Drivers in Chondrosarcoma
This chapter discusses the mutations and deregulated pathways identified in the different chondrosarcoma subtypes. It will focus on central conventional chondrosarcoma, the most extensively studied subtype. Mutations in IDH1 and IDH2 occur in central as well as dedifferentiated and periosteal chondrosarcoma. COL2A1 mutations and alterations in Indian hedgehog genes are seen in a small percentage of central chondrosarcoma. Deregulation of the hedgehog pathway occurs on most chondrosarcomas, indicating its importance to the development of these tumors. Peripheral chondrosarcomas are characterized by mutations in EXT1 or EXT2 . Both central and peripheral chondrosarcomas show alterations in p53 and pRb pathways when progressing from low to high grade.
Central, Dedifferentiated, and Periosteal Chondrosarcoma
IDH1 and IDH2 Mutations
Mutations in isocitrate dehydrogenase 1 and 2 ( IDH1 and IDH2 ), first identified in glioblastoma multiforme are found in 87% of enchondromas, 38%–70% of primary central chondrosarcomas, 54% of dedifferentiated chondrosarcomas, and 15% of periosteal chondrosarcomas. The occurrence of IDH1 and IDH2 mutations in enchondroma indicates that this is an early genetic event in the development of central chondrosarcoma (see Fig. 4.1 ). Also, in intracranial chondrosarcomas, but not in chordomas, IDH1 and IDH2 mutations have been reported, making it a good diagnostic marker in the differential diagnosis between the two different entities.
Cellular Consequences of an IDH1 or IDH2 Mutation
IDH1 and IDH2 are the enzymes that convert isocitrate to alpha-ketoglutarate (αKG) in the Kreb’s cycle. IDH1 is located in the cytoplasm and IDH2 in the mitochondria and they both convert isocitrate to αKG while NADPH is produced from NADH+. Mutations in IDH1 and IDH2 are heterozygous and affect only a single amino acid. In chondrosarcoma, the IDH1 mutation is always located on the R132 position in exon 4, and the IDH2 mutation on R172, which are the active sites of the enzymes. IDH1 and IDH2 mutations are mutually exclusive. When IDH1 or IDH2 is mutated, isocitrate cannot be converted to αKG because affinity for isocitrate is reduced. However, affinity for αKG and NADPH is increased, which leads to the conversion of αKG to the oncometabolite D2-hydroxyglutarate (D2HG) which consumes instead of produces NADPH. Also the enzyme activity of the wild-type IDH is inhibited by the mutant.
D2HG production has major consequences for enzymes that depend on αKG as a cosubstrate (2-OG-dependent dioxygenases). D2HG and αKG are structurally the same, except that the C2 carbonyl group of αKG is replaced by a hydroxyl group in D2HG. Because of the similarity of D2HG to αKG, D2HG acts as a competitive inhibitor by binding to the αKG binding pocket in the active site of the enzymes that depend on αKG.
Increased D2HG levels affect the ten-eleven translocation (TET) enzymes (TET 1/2/3). TET enzymes are demethylating enzymes that convert 5-methylcytosine (5-mC) to 5-hydroxymethylcytosine (5-hmC) in the first step in demethylation of cytosines. TET enzymes and 5-hmC have an important role in stem-cell development and differentiation. Simultaneous knock-down of all three TET proteins in mouse ES cells reduces 5-hmC, impairs differentiation, increases promoter hypermethylation, and deregulates expression of genes of embryonic development.
JMJC domain containing histone lysine demethylases (JHKDMs) are also inhibited by D2HG. These enzymes remove methyl groups from the lysine residues of histone proteins, changing the histone code and altering chromatin structure and transcription of genes.
Collagen and HIF prolyl-4-hydroxylases (PHDs) are additional αKG-dependent enzymes influenced by D2HG. Collagen PHDs are important for collagen folding and maturation. When these enzymes are inhibited, collagen cannot be folded properly and accumulates in the endoplasmic reticulum (ER). Hydroxylated collagen is a part of the extracellular matrix (ECM), which influences proliferation, differentiation, and invasion.
HIF PHDs are oxygen sensing enzymes that hydroxylate HIF1α and HIF2α under normoxic conditions, marking them for recognition by the Von Hippel Lindau (VHL) protein, which targets them for proteosomal degradation. Under hypoxic conditions there is no hydroxylation, and HIF1α and HIF2α can dimerize with HIF1β to form complete transcription factors targeting genes that function to increase oxygen delivery or enhance angiogenesis. Since HIF PHDs need αKG to hydroxylate HIF1α and HIF2α, the hypothesis is that IDH1 or IDH2 mutant tumors show a higher frequency of HIF1α and HIF2α activation, because they will not be recognized by VHL and targeted for degradation. The conflicting findings of both up- and downregulation of HIF1α and HIF2α in IDH1 or IDH2 mutant glioblastoma cells relative to that in wild-type tumor cells, however, suggest that a more complex mechanism mediates the function of HIF PHDs in IDH1 or IDH2 mutant tumors.
Mutations in IDH1 or IDH2 are expected to alter the metabolism of cells, due to IDH1 or IDH2 involvement in the Krebs cycle converting isocitrate to αKG or αKG to isocitrate. Mutations in IDH1 or IDH2 by inhibiting this process alters metabolic processes that depend on the Krebs cycle, such as glucose and glutamine metabolism and fatty acid production.
Mutant IDH1 or IDH2 expressing cells also have an impaired ability to neutralize reactive oxygen species (ROS) because the conversion of αKG to D2HG consumes one molecule of NADPH, which depletes NADPH and reduces formation of GSH (glutathione), an important antioxidant. Consequently, IDH mutant cells remove free radicals less effectively, which can lead to DNA mutations. The increased ROS in IDH1 or IDH2 mutant tumors can also affect sensitivity to chemo- and radiotherapy in gliomas and perhaps other tumor types with IDH1 or IDH2 mutations.
IDH1 and IDH2 Mutations in Enchondroma and Chondrosarcoma
In enchondromas with an IDH1 or IDH2 mutation, DNA hypermethylation is observed and levels of D2HG are higher in mutated cartilaginous tumors than in wild-type tumors.
Based on the hypothesis that enchondromas and chondrosarcomas result from altered differentiation of MSCs during bone development, mesenchymal stem cells (MSCs) have been used as a model system to assess the influence of the IDH1 or IDH2 mutation on the development of enchondroma. MSCs are the precursors of bone, cartilage, and fat tissue, and can be forced to differentiate into these specific lineages in vitro by adding stimulating growth factors. Addition of D2HG or introduction of an IDH1 R132C mutation blocks osteogenic differentiation and increases differentiation toward the chondrogenic lineage in human MSCs. Also, in zebra fish D2HG impairs the development of vertebrate rings, indicating a block in osteogenic differentiation during skeletogenesis. The introduction of IDH1 R132C both increases cartilage differentiation and results in formation of abnormal cartilage. This may involve an effect of increased D2HG levels on the PHD enzymes involved in collagen maturation.
Recently Hirata et al. described a conditionally inducible COL2A1 specific IDH1 132 knock-in mouse model, which showed multiple enchondroma-like cartilage lesions adjacent to the growth plates of the knee after 3 months. Also, after 6 months the same lesions were still present, without significant differences in size or amount. Cartilage lesions showed patchy expression of COL10A1 , indicating deregulated chondrocyte differentiation. Chondrocytes cultured from the lesions of those mice showed increased expression of hedgehog target genes and genes expressed by hypertrophic chondrocytes. A defect in differentiation and a hypermethylation phenotype were observed in IDH2 R172 knock-in murine 10T1/2 mesenchymal progenitor cells. Moreover, subcutaneous injection of these cells in mice led to the formation of undifferentiated sarcomas in vivo, indicating that IDH2 R172 also has tumor-promoting potential.
In chondrosarcoma cell lines, and in primary tumors of patients with enchondroma and chondrosarcoma with an IDH1 or IDH2 mutation, increased DNA methylation relative to that in wild-type cell lines is observed. This hypermethylation was only found at CpG islands.
Increased levels of D2HG were found in chondrosarcoma cell lines with IDH1 or IDH2 mutations compared to wild-type cell lines and could be diminished by treatment with a mutant-specific IDH1 inhibitor (AGI-5198), but no effect was observed on cell viability, proliferation, and migration. Likewise, culturing cells for longer periods in the presence of AGI-5198 did not reduce cell viability. Only after treatment with extremely high concentrations an effect on colony formation, migration, and apoptosis was observed.
The observation of only minor effects after inhibition of IDH1 mutant function, and consequently D2HG levels, suggests that mutations in IDH1 and IDH2 are not essential for the proliferation and metastatic capacity of malignant chondrosarcoma cells and that IDH1 or IDH2 mutations fail to be driver mutations once progression toward malignant chondrosarcoma is completed.
COL2A1 Mutations
Collagen type-II alpha1 ( COL2A1 ) mutations have been identified in 37% and 19.3% of central chondrosarcomas and 31.7% of enchondromas. Type-II collagen is the major extracellular component of cartilage and is important for the strength and flexibility of the tissue. Mutations in the COL2A1 gene can lead to abnormalities in collagen formation. Immunohistochemical analysis has revealed that only a small part of chondrosarcomas have complete loss of the COL2A1 protein, while most others retain focal staining. Mutations in COL2A1 are associated with several developmental disorders called type-II collagenopathies, but the incidence of enchondromas or chondrosarcomas is not increased in these disorders. Also, in mice expressing a mutant COL2A1 , increased chondrocyte proliferation was not observed but the structure of the collagen network was altered and the growth plate was disorganized. Downregulation of COL2A1 in cultured mouse chondrocytes resulted in upregulation of collagen I. Also Hedgehog signaling was affected by knock-down of COL2A1 , which disrupted chondrocyte differentiation. Whether mutations in COL2A1 are drivers of chondrosarcoma formation is still to be determined.
Dysregulation of the Hedgehog Pathway
The Indian hedgehog (IHH) pathway is important for bone development. Chondrocytes of the growth plate undergo differentiation, resulting in longitudinal growth of the bone. This process is regulated by Indian hedgehog and parathyroid hormone–like hormone (PTHLH) in a negative feedback loop. This feedback loop inhibits terminal differentiation of chondrocytes. Chondrocytes are differentiating toward hypertrophic chondrocytes which will be replaced by osteoblasts. Prehypertrophic chondrocytes produce IHH, stimulating proliferation of chondrocytes and stimulating the cells of the perichondrium to produce PTHLH. PTHLH inhibits IHH as well as terminal differentiation of chondrocytes. Constitutive activation of the hedgehog pathway results in failure to induce terminal differentiation of growth plate chondrocytes, which can result in the development of enchondromas.
Expression studies show that the hedgehog pathway members are highly expressed in enchondromas and in central chondrosarcomas, maintaining tumor cells in a less differentiated, but proliferative state. Levels of expression are variable, but there is no correlation with histological grade. Blocking of the hedgehog pathway with triparanol or IPI-926 reduces tumor volume in chondrosarcoma xenografts, while cyclopamine does not decrease proliferation of chondrosarcoma cell lines suggesting that the tumor microenvironment affects this pathway. Also the potency of the different compounds used in these studies can differ.
Patients with enchondromatosis show mutations in one of the genes involved in the IHH pathway in 8% of cases. Also, mice expressing a mutant parathyroid hormone–like hormone receptor specifically in chondrocytes have a deregulated growth plate and form cartilaginous lesions.
Hedgehog signaling is activated by binding of hedgehog ligand to the patched receptor, releasing the smoothened receptor and freeing Gli transcription factors to travel to the nucleus to activate transcription. Similar to the mutant PTHLH receptor expressing mice, mice constitutively expressing Gli2 in their chondrocytes also develop cartilaginous lesions.
Primary cilia have been shown to be involved in IHH signaling and to regulate its activity. Primary cilia are also important for the columnar organization of the growth plate. Chondrosarcoma cells express fewer cilia than do normal chondrocytes, and fewer cilia correlates with deregulated hedgehog signaling. Inhibition of the hedgehog pathway in enchondromas and central chondrosarcomas is a potential therapeutic strategy for patients with inoperable or metastatic disease. A phase-II clinical trial investigating the efficacy of GDC-0449 smoothened inhibitor in 45 patients with progressive advanced chondrosarcoma found only modest activity, with stable disease in only 10 patients with grade-I tumors after 6 months.
Recently, mutations in IHH genes have been identified in 18% of chondrosarcomas using whole exome sequencing. If these tumors respond better to hedgehog pathway inhibitors, this mutation may be a marker for selecting patients for such treatment.
P53 and pRb Pathway Alterations in the Progression From Low to High-Grade Chondrosarcoma
Mutations in the TP53 gene are the most frequently observed mutations in cancer and are often associated with a more aggressive phenotype and worse prognosis. Mutations in TP53 can lead to either loss or gain of function. Wild-type p53 has important functions in controlling cell proliferation and apoptosis, and loss of function mutations lead to cancer, as shown in a TP53 knock-out mouse model. However, point mutations in TP53 will lead to pathogenic accumulation of p53 in the nucleus, and mutant TP53 knock-in mice develop highly invasive tumors.
In chondrosarcoma, TP53 mutations are observed in 20% of cases and are associated with more malignant tumors and a higher histological grade. Also, increased nuclear p53 expression is correlated with a higher histological grade and worse survival. Mice constitutively expressing Gli, crossed with p53 knock-out mice, developed low-grade chondrosarcomas, indicating that deregulation of p53 is important in the progression of enchondromas to chondrosarcoma.
The retinoblastoma (pRb) pathway is important in the control of the cell cycle. It governs the restriction point transition (R-point), determining whether a cell progresses to S-phase or retreats to G0. RB gene mutations have been found in several cancer types but not in chondrosarcoma. Also, pRb expression was normal in chondrosarcomas.
Amplification of 12q13 occurs consistently in central chondrosarcoma. Both cyclin-dependent kinase 4 ( CDK4 ) and mouse double minute 2 homolog ( MDM2 ) are located in this region; they have important roles in the pRb and p53 pathways. CDK4 forms a complex with cyclin D1 and together they control the transition through the G1 restriction point by inactivating pRb. Consistent with the 12q13 amplification observed in a subset of chondrosarcomas, CDK4 and Cyclin D1 expression is upregulated in 62%–73% of high-grade chondrosarcomas. Furthermore inhibition of CDK4 decreases cell proliferation in chondrosarcoma cell lines. CDKN2A/p16 is a negative regulator of the CDK4-cyclin D1 complex and is downregulated in 20% of chondrosarcomas, which correlates with histological grade. Moreover, in 28% of chondrosarcomas mutations in CDKN2A are observed. However, no loss of CDKN2A/p16 has been found in enchondromas. Decreased cell growth, but not apoptosis, was observed in cells in which CDKN2A/p16 was reexpressed. MDM2 is a negative regulator of p53, targeting it for proteosomal degradation. MDM2 is overexpressed in 34% of high-grade chondrosarcomas, indicating that p53 is degraded at a higher rate in these tumors.
The combined incidence of mutations in either the p53 or the pRb pathway in high-grade chondrosarcomas is 96%. This suggests that these pathways are important in the progression of chondrosarcoma toward a more malignant histological phenotype.
Apoptosis and Prosurvival Pathways Implicated in Chondrosarcoma
A downstream target in the hedgehog pathway is Bcl-2, which is upregulated in the growth plate and inhibits differentiation of proliferating chondrocytes after PTHrP binds to its receptor. In most conventional chondrosarcomas, and rarely in other chondrosarcoma subtypes, Bcl-2 is highly expressed.
By upregulating antiapoptotic proteins of the Bcl-2 family, chondrosarcoma cells acquire resistance to chemotherapy, a major problem in the treatment of these tumors. This has been shown in a cell model where chondrosarcoma cells could be sensitized to chemotherapy by a Bcl-2 family inhibitor (ABT-737).
Survivin is also highly expressed in chondrosarcoma. Survivin plays important roles in the cell cycle and the apoptotic pathways. As it is expressed only at very low levels in normal tissue, it is an ideal therapeutic target. In preclinical studies a small molecule survivin inhibitor (YM155) decreased cell viability in chondrosarcoma cell lines, a response dependent on TP53 mutation status. Also downregulation of survivin using siRNAs decreased cell viability and increased cell sensitivity to chemotherapeutics.
Several studies have shown the importance of the Akt-Pi3K-mTOR pathway in chondrosarcoma. In 69% of conventional chondrosarcomas and 44% of dedifferentiated chondrosarcoms, S6 was phosphorylated, indicating that the PI3K/mTOR was active. Also treatment with a dual PI3K/mTOR inhibitor (Bez235) reduced cell viability in chondrosarcoma cell lines and inhibited tumor growth in a mouse xenograft model. In a rat chondrosarcoma model, treatment with everolimus inhibited tumor growth.
Peripheral Chondrosarcoma
EXT1 and EXT2 Mutations in Osteochondroma and Peripheral Chondrosarcoma
Multiple osteochondromas (MO) is an autosomal dominant condition in which mutations in the tumor suppressor genes, exostosin glycosyltransferase 1 or exostosin glycosyltransferase 2 ( EXT1 and EXT2 ), have been identified. Patients with this disease develop osteochondromas at the surfaces of their bones and show heterozygous germline EXT1 or EXT2 mutations, with loss of the wild-type allele in the osteochondroma cartilaginous cap. Also, homozygous deletions in EXT1 have been identified in a subset of sporadic osteochondromas. Both hereditary and sporadic osteochondromas can progress toward peripheral chondrosarcoma (see Fig. 4.1 ). This occurs in ∼1% of sporadic and up to 5% of hereditary osteochondromas.
Exostosin-1 and exostosin-2 are both type-II glycosyltransferases, which participate in the biosynthesis of the heparan sulfate chains of proteoglycans. Proteoglycans regulate the signaling of several growth factors in many different ways. They can act as receptors or coreceptors in signaling pathways, regulate receptor trafficking by endo- and exocytosis, facilitate ligand secretion, function as a signal to other cells, or present a ligand to a receptor. In addition, they can regulate the structure of the extracellular matrix by providing a signaling gradient to ligands. In the growth plate, heparan sulfate is important for the establishment of a gradient of IHH (as discussed above). When the biosynthesis of heparan sulfate is deregulated, the diffusion of IHH signaling is disturbed. This disrupts the polar organization of growth plate chondrocytes and the formation of the bony collar, leading to the formation of an osteochondroma.
In a conditionally inducible mouse model, where both Ext1 alleles were inactivated specifically in chondrocytes, osteochondromas developed, mimicking the human situation. The osteochondromas consisted of a mixture of wild-type and mutated cells, indicating that cells need normal heparan sulfate synthesis to survive. In another mouse model with heterozygous loss of both Ext1 and Ext2 , heparan sulfate was decreased and more exostoses appeared in comparison to mice with heterozygous loss of Ext1 or Ext2 . Zebra fish with homozygous loss of Ext2 in their chondrocytes show osteochondroma-like lesions and altered mesenchymal stem-cell differentiation.
Osteochondromas can progress to peripheral chondrosarcomas in a small percentage of cases. Most peripheral chondrosarcomas have at least one wild-type allele for EXT1 and EXT2 , indicating that functional EXT is required for cells to survive and to undergo malignant progression. Most likely, additional genetic alterations are needed for progression to peripheral chondrosarcoma and they probably arise in the EXT1 and EXT2 wild-type cells.
p53 and pRb Pathway Alterations
In peripheral chondrosarcoma, pRb and p53 pathway alterations occur in most cases, especially in tumors with higher histological grade. A mouse model with conditionally inducible Ext1 inactivation specifically in chondrocytes combined with conditional loss of Trp53 or Ink4a/Arf developed peripheral chondrosarcomas from Ext -null and Ext -mutated cells, mimicking the human situation. The tumors lacked both primary cilia and polar organization. This indicates that in addition to the EXT mutation, an alteration in one of the pathways regulating the cell cycle is needed for the development of peripheral chondrosarcoma from an osteochondroma.
Mesenchymal Chondrosarcoma: HEY1-NCOA2 Fusion
Mesenchymal chondrosarcoma is characterized by a specific gene fusion between HEY1 (Hes-related family BHLH transcription factor with YRPW motif 1) and NCOA2 (nuclear receptor coactivator 2). HEY1 is a basic helix-loop-helix transcription factor that functions downstream of the Notch signaling pathway. In rhabdomyosarcoma, inhibition of Hey1 downregulates Notch1 expression and myogenic differentiation. NCOA2 , also known as SRC2 (steroid receptor coactivator 2), is a nuclear hormone receptor coactivator important for chromatin remodeling. Overexpression of NCOA2, found in prostate cancer, correlates with metastatic disease. Rearrangements involving NCOA2 have been identified in other sarcomas as well. The exact mechanism by which the HEY1-NCOA2 fusion leads to the formation of mesenchymal chondrosarcoma is currently unknown.
Clear-Cell Chondrosarcoma
Clear-cell chondrosarcomas are rare chondrosarcomas subtypes, and few genetic defects have been identified in these tumors. Array-CGH analysis has identified hemizygous loss of the CDKN2A/p16 locus in 4 of 12 clear-cell chondrosarcomas. However, loss of p16 protein expression has been identified in 95% of cases, indicating that aberrations in the pRb pathway are important for the development and/or progression of this tumor type.
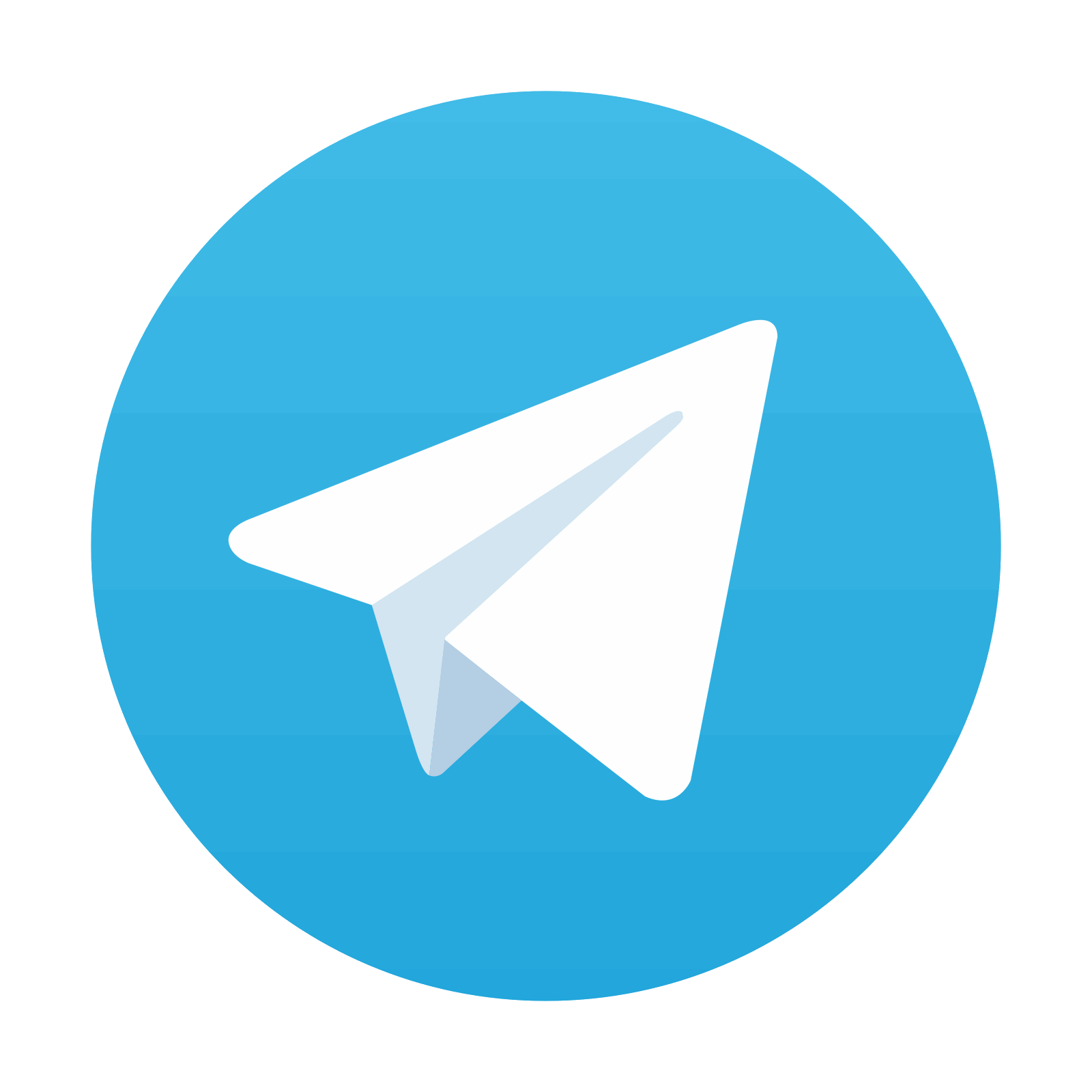
Stay updated, free articles. Join our Telegram channel

Full access? Get Clinical Tree
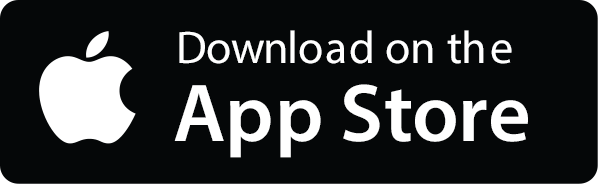
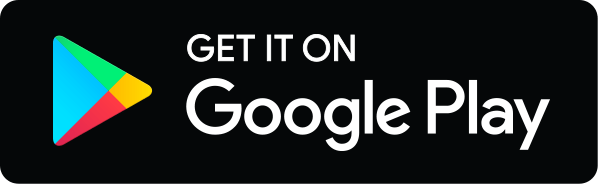